Jump to a key chapter
Leidenfrost Effect Definition
The Leidenfrost Effect is a fascinating physical phenomenon that occurs when a liquid comes into near contact with a surface significantly hotter than its boiling point. Due to rapid vaporization, a layer of vapor forms between the liquid and the surface, causing the liquid to skitter across the surface instead of boiling immediately.
In the realm of physics, the Leidenfrost Effect refers to the creation of an insulating vapor layer beneath a liquid droplet, preventing direct contact and prolonging evaporation due to excessive temperatures of the substrate.
This effect is named after Johann Gottlob Leidenfrost, who described it in 1756.
Mechanism of the Leidenfrost Effect
To understand how the Leidenfrost Effect works, consider what happens when a droplet of water falls onto a heated skillet. If the skillet is only moderately heated, the water will boil rapidly. However, when the skillet is much hotter than 100°C, the water droplet hovers and moves smoothly over the surface. This is due to a layer of steam forming beneath the droplet, which drastically reduces heat transfer due to its insulating properties.
Imagine placing a droplet of water on a pan heated to a temperature of 300°C. Due to the Leidenfrost Effect, instead of evaporating immediately, the droplet forms an insulating vapor cushion, allowing it to move around effortlessly.
The efficiency of this vapor layer can be quantified using the Leidenfrost temperature, which is specific to the fluid and surface properties involved. This temperature is significantly higher than the fluid's boiling point. Interestingly, the physics behind this can be investigated through the well-known Newton's Law of Cooling, and the effect can be observed when the surface temperature exceeds the Leidenfrost temperature threshold, \[ T_s > T_L \] where \( T_s \) is the surface temperature and \( T_L \) is the Leidenfrost temperature.
The Leidenfrost Effect has intriguing implications in physics and engineering applications. For example, it influences the design of systems where heat transfer efficiency is critical, such as in nuclear reactors or industrial cooling processes. The situation gets even more interesting when considering the phase change dynamics and thermal stresses experienced by materials in extreme environments.
Practical Applications and Observations
Understanding the Leidenfrost Effect is crucial for improving systems requiring effective heat management. In manufacturing, certain processes use this phenomenon to enhance safety and efficiency by controlling cooling rates. Digital circuit cooling is an area where this effect is actively considered to prevent overheating and ensure reliable performance.
Leidenfrost Effect Explained
The Leidenfrost Effect is a captivating thermal phenomenon that is observed when a liquid droplet hovers over a surface much hotter than the liquid's boiling point. A thin insulating layer of vapor is created between the droplet and the surface, reducing heat transfer. This effect causes the droplet to levitate and move with little friction across the surface.
The Leidenfrost Effect occurs when a liquid in near contact with a surface significantly surpassing its boiling temperature forms an insulating vapor layer, leading to droplet motion and reduced evaporation rate.
In simple terms, the Leidenfrost Effect can make water droplets dance on a very hot skillet.
Key Principles of the Leidenfrost Effect
When examining the mechanics of the Leidenfrost Effect, a few critical aspects must be understood:
- Surface Temperature: The substrate temperature must be considerably higher than the boiling point of the liquid for the effect to occur.
- Vapor Layer: This layer serves as an insulating barrier that minimizes direct contact, hence decreasing heat transfer.
- Droplet Motion: The vapor layer allows the droplet to glide effortlessly across the surface.
Consider a droplet of water placed on a metal plate heated to 250°C. Instead of evaporating immediately, the droplet forms a vapor shield that allows it to spin and roll over the plate surface, demonstrating the Leidenfrost Effect.
Exploration into the Leidenfrost Effect provides valuable insights into evaporation dynamics and thermal properties of materials. It is applied in various fields, such as:
- Engineering: Enhancing the performance of cooling systems by optimizing evaporation under extreme conditions.
- Material Science: Studying surface characteristics to reduce friction and heat loss in manufacturing processes.
- Thermal Management: Improving design of heat shields and insulating layers in aerospace technology.
Leidenfrost Effect Temperature and Leidenfrost Point
The Leidenfrost Effect manifests uniquely at different temperatures, revealing critical thermal properties. Understanding the temperature dynamics is essential for practical applications in fields ranging from cooking to industrial cooling.
Understanding the Leidenfrost Temperature
The Leidenfrost Temperature is the point beyond which the Leidenfrost Effect becomes evident. Before this temperature, a liquid droplet sitting on a heated surface will evaporate quickly and violently. However, at temperatures exceeding the Leidenfrost Point, the droplet levitates on a cushion of vapor. Several factors influence this temperature, including:
- Surface Material: Different materials conduct heat differently, affecting the Leidenfrost Temperature.
- Fluid Properties: Specific heat and boiling points of fluids have a direct impact.
- Surface Texture: Rough surfaces disrupt the vapor layer, changing the dynamics.
For water, the Leidenfrost Temperature is typically around 200°C to 250°C on a stainless steel pan. At this temperature, a droplet starts to dance rather than evaporating instantly, showcasing the insulating vapor layer.
Did you know? The phenomenon of droplets dancing on a hot pan is not just a curious sight but also a demonstration of heat transfer principles.
The physics behind the Leidenfrost Effect can also be explored through thermodynamic equations. For instance, the vapor layer's insulating capability is linked to the thermal conductivity of the fluid and its vaporization enthalpy. The formula for heat flow can be represented as: \[ q = k \times A \times \frac{\triangle T}{d} \] where \( q \) is the heat flow, \( k \) is the thermal conductivity, \( A \) is the surface area, \( \triangle T \) is the temperature difference, and \( d \) is the thickness of the vapor layer. This complex relationship defines the efficiency and sustainability of the vapor cushion beneath the droplet. Understanding and manipulating these parameters can lead to advanced applications in designing surfaces with desired heat retention and dissipation characteristics.
Leidenfrost Effect Applications and Examples
The Leidenfrost Effect is not just a scientific curiosity but an essential phenomenon for various practical applications. From culinary arts to advanced engineering, the understanding and manipulation of this effect have led to innovative solutions across different fields.
Industrial Applications of the Leidenfrost Effect
In the industrial sector, the Leidenfrost Effect is leveraged in numerous processes to enhance efficiency and safety.
- Chemical Industry: In high-temperature reactors, controlling the Leidenfrost Effect can help manage heat transfer and prevent runaway reactions.
- Metalworking: During quenching, the formation of a vapor layer can ensure controlled cooling rates, minimizing thermal stresses in the metal.
Imagine a scenario in steel manufacturing. By adjusting the temperature to favor the Leidenfrost Effect, a hot steel rod can be cooled rapidly and uniformly, preventing warping and cracking. This ensures a stronger product with fewer defects.
Culinary and Everyday Demonstrations
In the culinary world, the Leidenfrost Effect is well-known. Chefs often use the phenomenon to check if a pan is hot enough for cooking. A splash of water that beads and skitters indicates perfect cooking temperature. Additionally, this effect finds its way into household experiments, providing a safe and visually striking example of physics in action. For instance, holding a damp finger above a heated metal surface and watching water droplets levitate can be an eye-opening demonstration.
Next time you cook, try sprinkling a few drops of water onto the pan to see the Leidenfrost Effect in action!
Engineering Innovations and Heat Management
Engineers often draw on principles of the Leidenfrost Effect to design more efficient cooling systems. In microelectronics, where overheating can be detrimental, exploiting the vapor layer's insulating properties can enhance performance and extend component lifetimes. To better illustrate, consider a cooling system that maintains electronic chips at an optimal temperature by exploiting the Leidenfrost Effect. The presence of a vapor barrier reduces direct heat transfer, resulting in a consistent and efficient cooling process.
The physics behind the Leidenfrost Effect also inspires material scientists to create surfaces that can strategically manipulate heat transfer characteristics. By engineering surface textures and materials that influence vapor layer formation, they can control processes in new ways. Specifically, the formula: \( q = h \cdot A \cdot (T_{surface} - T_{vapor}) \) where \( q \) is the heat flux, \( h \) is the heat transfer coefficient, \( A \) is the area, and \( T_{surface} - T_{vapor} \) the temperature differential, provides the groundwork for designing these innovative surfaces.
leidenfrost effect - Key takeaways
- Leidenfrost Effect Definition: A phenomenon where a liquid droplet hovers over a surface hotter than its boiling point due to an insulating vapor layer.
- Leidenfrost Temperature: The temperature threshold above which the effect occurs, typically significantly higher than the fluid's boiling point.
- Mechanism Explained: The vapor layer under the droplet reduces heat transfer, allowing it to glide across the hot surface.
- Critical Conditions: Requires surface temperature (\
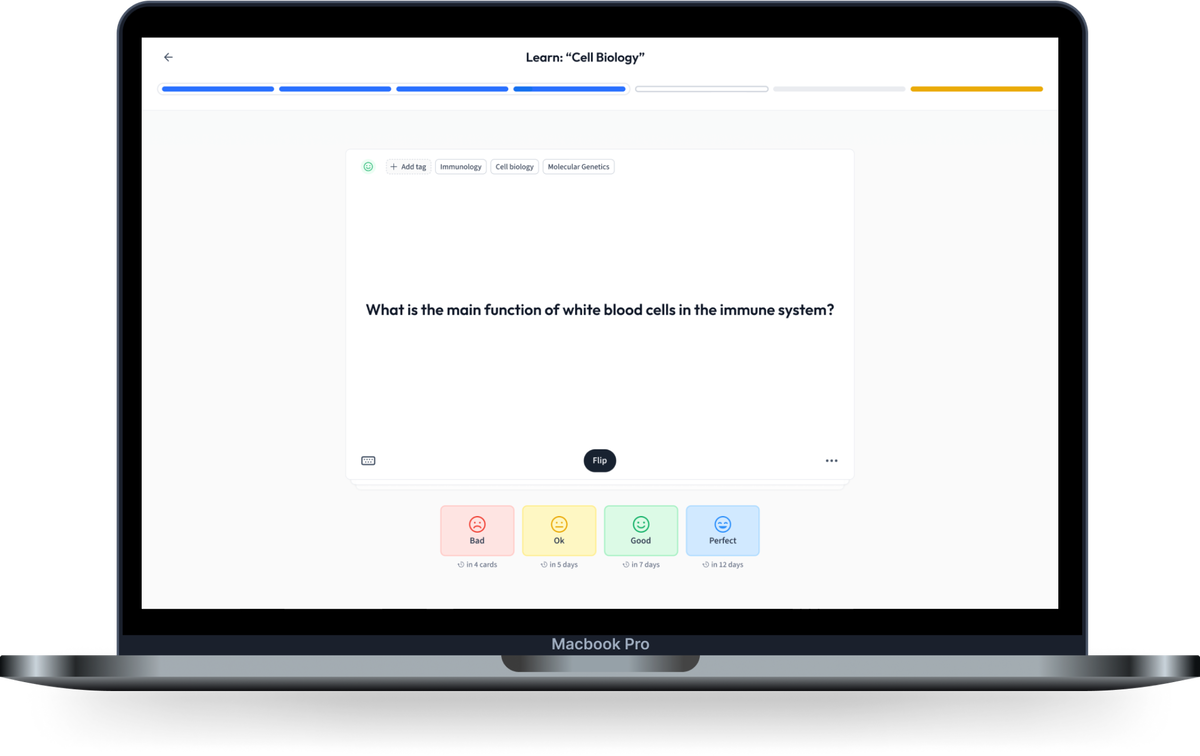
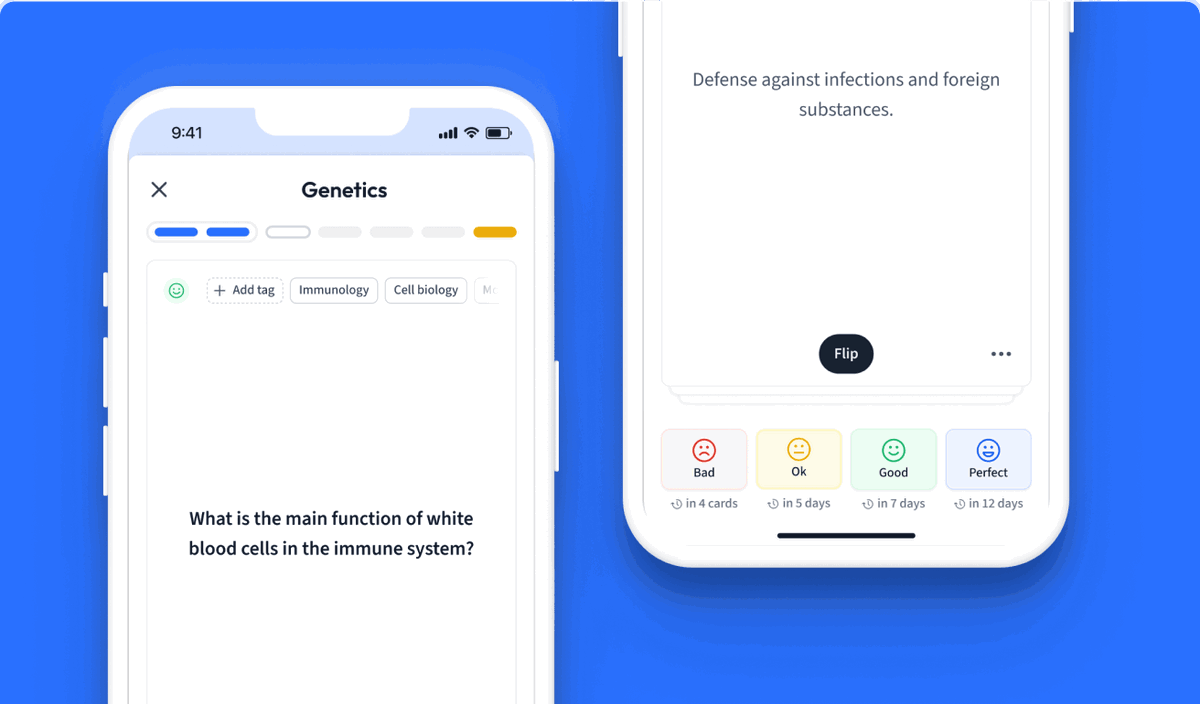
Learn with 12 leidenfrost effect flashcards in the free StudySmarter app
Already have an account? Log in
Frequently Asked Questions about leidenfrost effect
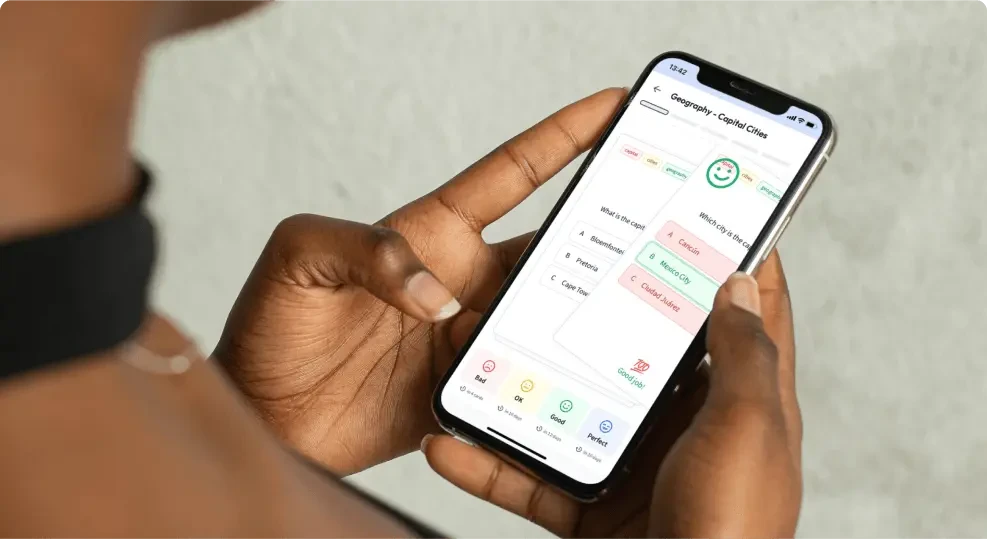
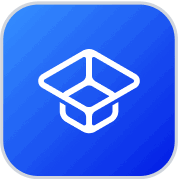
About StudySmarter
StudySmarter is a globally recognized educational technology company, offering a holistic learning platform designed for students of all ages and educational levels. Our platform provides learning support for a wide range of subjects, including STEM, Social Sciences, and Languages and also helps students to successfully master various tests and exams worldwide, such as GCSE, A Level, SAT, ACT, Abitur, and more. We offer an extensive library of learning materials, including interactive flashcards, comprehensive textbook solutions, and detailed explanations. The cutting-edge technology and tools we provide help students create their own learning materials. StudySmarter’s content is not only expert-verified but also regularly updated to ensure accuracy and relevance.
Learn more