Jump to a key chapter
Definition of Parallel Flow Heat Exchanger
Parallel flow heat exchangers are devices used to transfer heat between two fluids that flow in the same direction. They are common in various industries, including power generation and chemical processing. Understanding these devices helps in designing more efficient systems.
Basic Concept of Parallel Flow Heat Exchanger
In a parallel flow heat exchanger, both the hot and cold fluids enter the heat exchanger at the same end and move parallel to each other. This type of heat exchanger is easy to design and manufacture but has limitations in terms of heat transfer efficiency compared to other types like counter-flow exchangers. The general principle behind a parallel flow exchanger involves transferring thermal energy from the hotter fluid to the cooler fluid.Mathematically, the overall heat transfer coefficient can be calculated using:
- \[U = \frac{Q}{A \times \Delta T_{lm}}\]where:
- U is the overall heat transfer coefficient,
- Q is the heat transfer rate,
- A is the area available for heat exchange,
- \Delta T_{lm} is the log mean temperature difference.
When both fluids enter at similar temperatures, the efficiency of heat transfer in a parallel flow setup is limited due to the small temperature difference.
Design Characteristics of Parallel Flow Heat Exchanger
The design of parallel flow heat exchangers involves several key considerations:
- Material selection: The heat exchanger materials must withstand pressure and temperature conditions.
- Flow arrangement: Ensures the fluids enter and exit without mixing.
- Size and surface area: A larger surface area enhances heat transfer but also increases cost and space requirements.
- \rho is the fluid density,
- u is the fluid velocity,
- D is the characteristic diameter,
- \mu is the dynamic viscosity.
Consider designing a parallel flow heat exchanger for a power plant. The objective is to cool water using another fluid without them mixing. The choice of the cooling fluid and the material for the heat exchanger is essential based on the operational environment and desired outcomes.
Interestingly, while parallel flow heat exchangers are relatively straightforward, they do offer some benefits in specific situations. For example, when initial temperature differences are very high, a parallel flow design can adequately lower the temperature to a desired level. However, finding a balance between cost, material suitability, and efficiency remains central to effective heat exchanger design.
Applications of Parallel Flow Heat Exchanger in Engineering
Parallel flow heat exchangers are vital in many engineering applications due to their simplicity and ease of maintenance. Although other types of heat exchangers might offer higher thermal efficiencies, the straightforward design makes parallel flow exchangers useful in specific scenarios.
Industrial Uses of Parallel Flow Heat Exchanger
Parallel flow heat exchangers are employed in various industrial processes where space constraints and maintenance simplicity outweigh the need for maximum thermal efficiency. These exchangers are beneficial in the following industries:
- Chemical processing: Used to preheat chemical mixtures before reactions.
- Food and beverage: Essential for pasteurization and sanitation through heating processes without cross-contamination.
- Power generation: Helps in managing heat within turbines by cooling down fluids under controlled conditions.
- \(\Delta T_1\) represents the temperature difference at the entry.
- \(\Delta T_2\) is the temperature difference at the exit.
Industries often choose parallel flow exchangers when initial fluid temperature differences are large, as they are simpler to maintain and cost-effective.
Parallel Flow Heat Exchanger in HVAC Systems
In the realm of Heating, Ventilation, and Air Conditioning (HVAC) systems, parallel flow heat exchangers play an instrumental role by enabling effective temperature control with minimal energy wastage. They achieve this through efficient heat exchange between incoming and outgoing air streams.A key advantage in HVAC is their ability to integrate into compact designs, making them suitable for residential and commercial installations without excessive space requirements. When considering heat exchanger selection and operation, pay attention to parameters like:
- Size: Smaller units fit snugly into space-constrained HVAC configurations.
- Efficiency: Suitable for moderate efficiency requirements where simplicity is preferred.
- Cost: Provide a cost-effective solution for initial setup and regular maintenance.
- \(f\) is the friction factor,
- \(\Delta P\) represents the pressure loss,
- \(\frac{L}{D}\) is the length-to-diameter ratio,
- \(\rho\) is the fluid density,
- \(V\) is the velocity of the fluid.
Consider an HVAC system needing a thermostat controlled environment. The linking of a parallel flow heat exchanger offers a simple and compact design, enhancing air handling and thermal regulation when compared with more complex configurations.
Beyond basic uses, parallel flow exchangers offer unique possibilities. In HVAC, engineered systems might deploy phase change materials (PCMs) within the heat exchange process. PCMs absorb excess heat and store it efficiently, releasing it when temperatures drop. This can elevate typical parallel flow systems to handle temperature fluctuations without additional energy input, showcasing the potential for future advanced industrial applications.
Advantages of Parallel Flow Heat Exchanger
Parallel flow heat exchangers offer notable advantages that make them suitable for various applications. These benefits enhance their appeal in industries where maintaining simplicity and minimizing cost are crucial. Understanding these advantages can help in deciding when to use these heat exchangers.
Efficiency Benefits of Parallel Flow Heat Exchanger
Efficiency in parallel flow heat exchangers, while not as high as other configurations, remains sufficient for many applications. The key efficiency benefits include:
- Simplicity in Design: Easier construction and maintenance lead to reduced operational complexity.
- Uniform Temperature Reduction: Both fluids gradually reach equilibrium, offering a steady cooling or heating process beneficial for specific industrial tasks.
- \(T_{exit}\) is the exit temperature,
- \(T_{entry}\) is the initial temperature of the hot fluid,
- \(Q\) is the heat transferred,
- \(\dot{m}\) is the mass flow rate,
- \(c_p\) is the specific heat capacity of the fluid.
The simplicity in design also means fewer components, reducing the chance of mechanical failures.
Parallel flow exchangers can be part of more complex systems. When integrating into a larger setup, consider attributes like capacity limits and temperature thresholds, which define their operational scope and efficiency. Engineers often use them in tandem with other exchangers to balance efficiency and design constraints.
Cost-Effectiveness of Parallel Flow Heat Exchanger
Another major advantage of parallel flow heat exchangers is their cost-effectiveness.Factors contributing to cost-effectiveness include:
- Lower Manufacturing Costs: The simpler design requires fewer materials and less time to fabricate.
- Reduced Maintenance: Easier access to components allows for straightforward maintenance, keeping downtime and costs low.
- \(CPR\) is the cost-performance ratio,
- \(C_{maintenance}\) is the maintenance cost,
- \(C_{operational}\) refers to operational costs,
- \(\eta\) is the overall efficiency.
Consider a small manufacturing unit where budget constraints necessitate a focus on cost. Using a parallel flow heat exchanger in this setting allows the facility to maintain stable operations without the high costs of more complex systems.
Heat Transfer in a Parallel Flow Heat Exchanger
In examining parallel flow heat exchangers, it's crucial to understand the basic mechanisms of heat transfer that occur within these systems. By evaluating these processes, you can gain insights into how these exchangers operate across different industrial applications.
Mechanism of Heat Transfer in a Parallel Flow Heat Exchanger
The mechanism of heat transfer in a parallel flow heat exchanger involves both convection and conduction as it achieves thermal equilibrium. Here, both hot and cold fluids enter the system from the same side and flow in the same direction.The heat transfer in these units primarily follows:
- Convective heat transfer: As fluids flow, convection plays a key role in transferring heat from the hot fluid to the walls of the heat exchange surface.
- Conduction: Once heat reaches the metal walls separating fluids, conduction transfers the energy to the cold fluid.
- \(Q\) represents the rate of heat transfer,
- \(U\) is the overall heat transfer coefficient,
- \(A\) is the heat transfer area,
- \(\Delta T_{lm}\) is the log mean temperature difference between the fluids.
Imagine using a parallel flow heat exchanger to cool down hot oil using water. Both fluids enter from the same side, with the temperature of oil steadily decreasing along the exchanger length due to convection and conduction.
The proximity of the fluid to the exchanger's surfaces increases the rate of conduction, enhancing overall heat transfer efficiency.
Exploring heat transfer on a molecular level, molecular motion within fluids contributes heavily to convective currents. The convection coefficient's determination depends largely on factors like fluid velocity and temperature, influencing the gradient of temperature along the exchanger. To dive deeper: Computational Fluid Dynamics (CFD) software often models these intricate mechanisms, simulating how turbulence and laminar flow regimes affect heat transfer efficiency, especially when dealing with complex fluids like non-Newtonian substances.
Factors Affecting Heat Transfer in a Parallel Flow Heat Exchanger
Several factors affect how effectively a parallel flow heat exchanger transfers heat. Understanding these elements allows for improved design and operation.
Factor | Effect |
Fluid Properties | The specific heat, density, and viscosity of the fluids affect heat transfer rates. |
Flow Rate | Higher flow rates increase the turbulent flow, enhancing convective heat transfer. |
Surface Area | Increased area provides more space for heat exchange, improving performance. |
- \(\rho\) is the fluid density,
- \(u\) is the fluid velocity,
- \(D\) is the diameter of the pipe or characteristic length,
- \(\mu\) is the dynamic viscosity.
Interestingly, the influence of fluid dynamics on heat transfer efficiency becomes more pronounced under variable operating conditions. Factors such as fouling — the accumulation of unwanted materials like dirt on heat exchange surfaces — can drastically reduce efficiency over time. Periodic cleaning and maintenance schedules reduce fouling impacts. In some industries, innovative materials or surface coatings minimize fouling potential, extending the exchanger’s operational lifespan without efficiency loss. This advances parallel flow exchanger usability in systems handling viscous or particulate-heavy fluids.
Calculating Effectiveness of Parallel Flow Heat Exchanger
Effectiveness is a measure of how well a heat exchanger transfers heat relative to its maximum potential. For a parallel flow heat exchanger, this can be calculated using the effectiveness equation:\[ \varepsilon = \frac{T_{co, exit} - T_{ci, in}}{T_{hi, in} - T_{ci, in}} \]where:
- \(T_{co, exit}\) is the cold fluid exit temperature,
- \(T_{ci, in}\) is the cold fluid inlet temperature,
- \(T_{hi, in}\) is the hot fluid inlet temperature.
Let's calculate the effectiveness when a hot fluid enters at 150°C and a cold fluid starts at 60°C. If the cold side exits at 90°C, the effectiveness is: \[ \varepsilon = \frac{90 - 60}{150 - 60} = 0.33 \]This indicates the exchanger uses only 33% of its potential thermal capacity, suggesting room for design improvements.
Example of Parallel Flow Heat Exchanger
To truly grasp the concept of parallel flow heat exchangers, examining real-world examples is invaluable. These examples not only depict practical applications but also highlight how these exchangers function under specific industrial conditions. Understanding how these systems operate within different contexts provides a comprehensive view of their utility and limitations.
Real-World Example of Parallel Flow Heat Exchanger
In the food industry, a simple yet effective application of a parallel flow heat exchanger can be seen in pasteurization processes. Here, milk is heated using hot water flowing in the same direction in a heat exchanger system:
- The milk enters the system at a temperature of 4°C and needs to be heated to at least 72°C.
- The hot water, entering at 80°C, flows alongside the milk.
- As both fluids continue in the same direction, heat transfer occurs from the water to the milk until their temperatures equalize or closely approach each other.
Log Mean Temperature Difference (LMTD) is a crucial measurement in heat exchanger design, often simplified for parallel flow systems: \[ \Delta T_{lm} = \frac{\Delta T_1 - \Delta T_2}{\ln(\frac{\Delta T_1}{\Delta T_2})} \]where \(\Delta T_1\) and \(\Delta T_2\) are temperature differences at the entry and exit points.
Consider a scenario where heat exchange efficiency needs to be evaluated. If the initial temperature difference \(\Delta T_1\) is 76°C (80°C - 4°C) and the final \(\Delta T_2\) is 8°C (80°C - 72°C), using the LMTD equation helps calculate the real temperature differential for system design.
Parallel flow exchangers are particularly useful in applications where initial temperature differences are significant. Under such conditions, substantial heat transfer can still be achieved efficiently.
Case Study: Parallel Flow Heat Exchanger in Practice
A detailed case study within an industrial chemical processing plant provides another insight into the application of parallel flow heat exchangers. Here, the objective is to control reaction temperatures by removing excess heat from chemical mixtures:The plant faced issues with excess heat accumulation during exothermic reactions. By implementing a parallel flow heat exchanger, the process was optimized as follows:
- Entering Cold Stream: Cooling water enters at 15°C to absorb the heat dissipated from the reactions.
- Hot Stream: Chemical mixtures reach temperatures up to 100°C, requiring controlled temperature management to maintain operational stability.
- Outcome: As both streams travel parallel, the cooling water absorbs heat from the chemical mixtures, allowing a safe reduction in the reaction vessel temperature.
Initial Costs: | Lower construction and installation costs due to the design's simplicity. |
Maintenance: | Ease of cleaning and inspection ensuring less downtime. |
In advanced settings, the integration of a parallel flow exchanger can be part of a hybrid system. The addition of oil-based coolants or specific surface treatments enhances thermal conduction and reduces material stress. These innovations allow parallel flow heat exchangers to handle more demanding industrial contexts, combining initial simplicity with advanced engineering solutions for diverse operational challenges.
parallel flow heat exchanger - Key takeaways
- Definition of Parallel Flow Heat Exchanger: A device that transfers heat between two fluids flowing in the same direction, frequently used in power generation and chemical processing.
- Heat Transfer Mechanism: Heat is transferred through convection and conduction, with both fluids entering from the same side, achieving thermal equilibrium.
- Effectiveness: Measures heat transfer relative to maximum potential, calculated using the effectiveness equation. It highlights the exchanger's ability to utilize its thermal capacity.
- Advantages: Simplicity in design, cost-effectiveness, and uniform temperature reduction, making it suitable for applications where extreme efficiency isn't critical.
- Applications in Engineering: Utilized in chemical processing, food and beverage for pasteurization, and power generation for turbine cooling, advantageous in space-constrained environments.
- Example: In pasteurization, milk is heated parallel to a hot water flow to ensure efficient heating without direct contact.
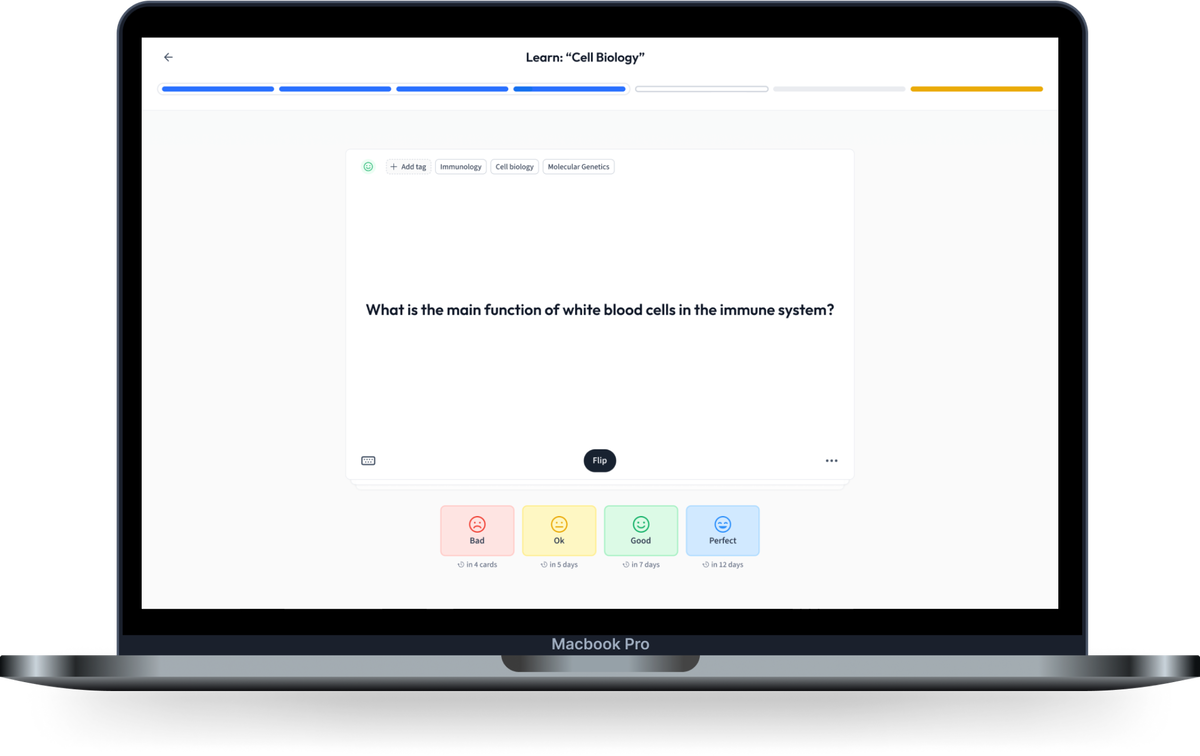
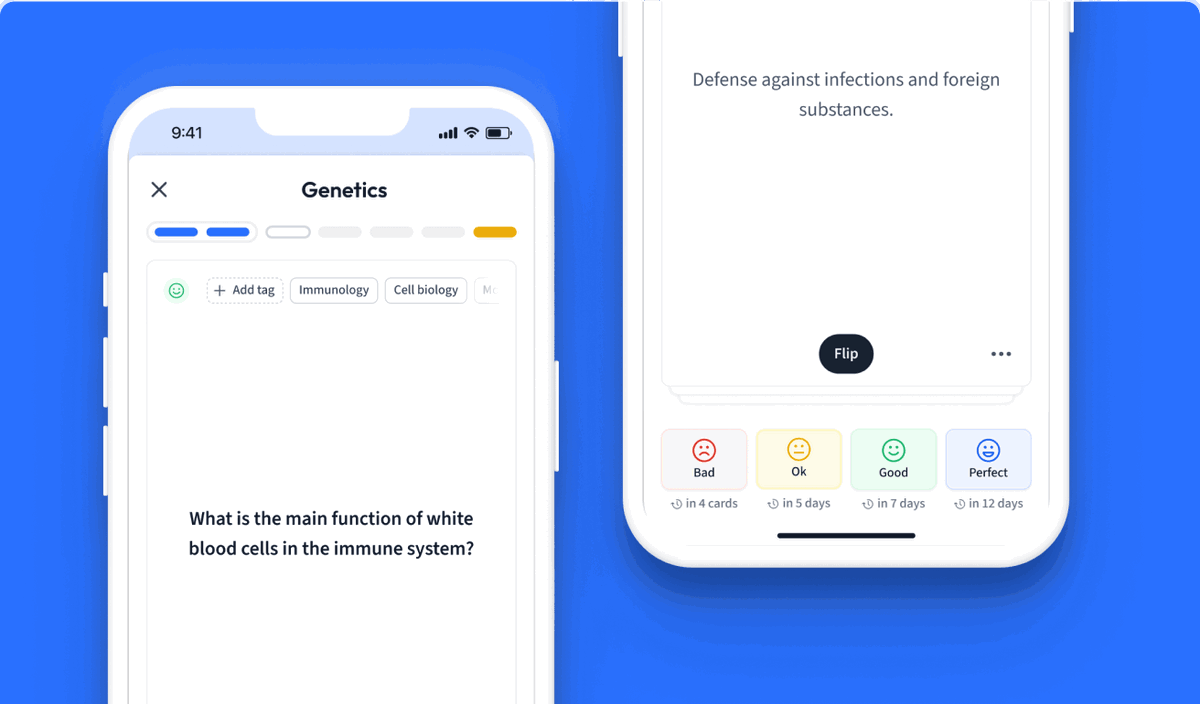
Learn with 10 parallel flow heat exchanger flashcards in the free StudySmarter app
Already have an account? Log in
Frequently Asked Questions about parallel flow heat exchanger
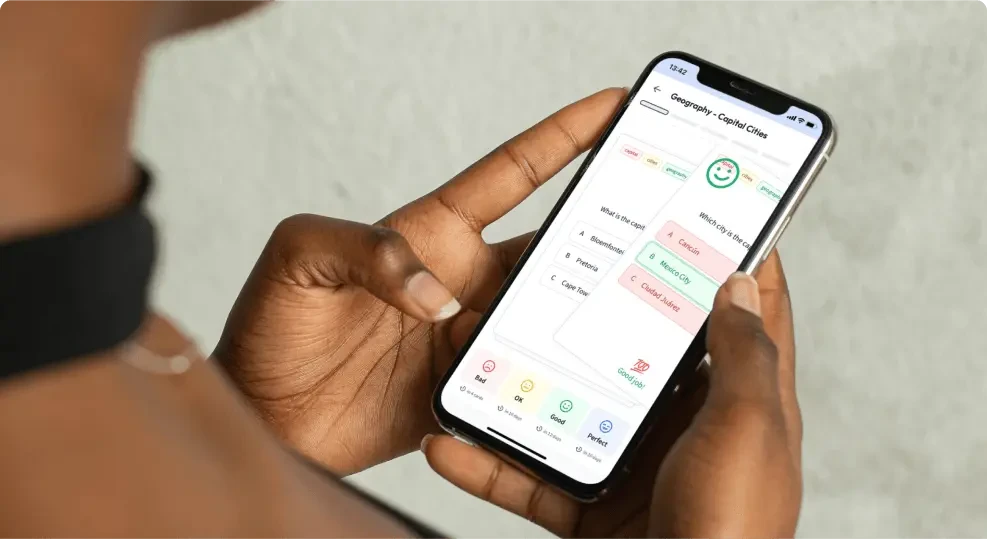
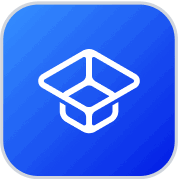
About StudySmarter
StudySmarter is a globally recognized educational technology company, offering a holistic learning platform designed for students of all ages and educational levels. Our platform provides learning support for a wide range of subjects, including STEM, Social Sciences, and Languages and also helps students to successfully master various tests and exams worldwide, such as GCSE, A Level, SAT, ACT, Abitur, and more. We offer an extensive library of learning materials, including interactive flashcards, comprehensive textbook solutions, and detailed explanations. The cutting-edge technology and tools we provide help students create their own learning materials. StudySmarter’s content is not only expert-verified but also regularly updated to ensure accuracy and relevance.
Learn more