Jump to a key chapter
Understanding Photoacoustics
The study of photoacoustics is fascinating, as it combines elements of physics, engineering, and medicine. By examining the intersection of light and sound, you gain insights into how these fundamental concepts interact.
Definition of Photoacoustics
Photoacoustics is the study of sound waves generated by the absorption of light. This absorption causes a rapid thermal expansion, producing sound waves that can be detected and analyzed.
- Photoacoustic Effect: When pulsed or continuous wave light is absorbed by a material, it results in thermal expansion and generates pressure changes, leading to sound waves.
- Interdisciplinary Nature: Integrates optics, acoustics, and laser technologies.
- Applications: Used in fields like medical imaging, environmental monitoring, and analytical chemistry.
Photoacoustics is also sometimes referred to as optoacoustics, highlighting the optical origins of the acoustic signals.
Key Photoacoustic Principles
To gain a thorough understanding of photoacoustics, it's crucial to grasp several key principles. These principles form the core of how photoacoustic systems operate and how they can be applied effectively. Some of these principles are:
Example: Consider a medical photoacoustic imaging application. A laser pulse is directed towards a tissue sample, where it is absorbed by blood vessels. The thermal expansion of the blood generates sound waves, which are then used to create a detailed image of the vasculature.
- Absorption: The material's capacity to absorb light affects the strength and quality of the generated sound waves.
- Thermal Expansion: The rapid heating resulting from laser absorption causes the material to expand, creating pressure waves.
- Acoustic Signal Detection: Sensitive detectors capture the sound waves generated, allowing for analysis and interpretation.
- Signal Processing: Critical for converting raw acoustic signals into usable data, often involving sophisticated algorithms.
The acoustic wave equation plays a pivotal role in modeling photoacoustic phenomena. This equation describes how pressure waves propagate within a medium. Researchers use it to predict the behavior of sound waves generated by photoacoustic effects, assisting in the design of more effective photoacoustic devices. Mastery of these equations and principles can greatly enhance the understanding and innovative application of this technology across various domains.
Photoacoustic Effect and Techniques
In the realm of science and technology, photoacoustics emerges as a bridge between light and sound, offering innovative applications and insights. By analyzing the sound waves induced by light absorption, you can uncover critical information across various fields.
Basics of the Photoacoustic Effect
The photoacoustic effect is rooted in the interaction between light and matter, leading to the generation of sound. When a material absorbs light, such as a laser pulse, it undergoes a rapid increase in temperature, which results in an expansion that produces sound waves. These sound waves carry vital information about the material composition and its properties.
Photoacoustic Effect: The conversion of absorbed light energy into acoustic waves due to thermal expansion.
The intensity of the sound produced in the photoacoustic effect is directly proportional to the absorbed light intensity.
The equation for the photoacoustic signal is expressed as: \[P(t) = \frac{\beta}{C_p} \frac{\text{d}T(t)}{\text{d}t}\],where \(P(t)\) is the pressure change, \(\beta\) is the thermal expansion coefficient, and \(C_p\) is the specific heat capacity at constant pressure. This equation shows how thermal properties of the material influence the acoustic signals.
Example: Imagine a laser beam striking a piece of tissue. The light energy absorbed by the tissue's pigments heats the local area. This rapid heating causes expansion and generates sound waves that form the foundation of medical imaging methods like photoacoustic tomography.
Various Photoacoustic Techniques Explored
There are several photoacoustic techniques that have been developed, each tailored for specific applications and materials. Some of the significant methods include:
- Photoacoustic Spectroscopy: Used in chemical analysis to detect gases and pollutants by examining the generated acoustic waves.
- Photoacoustic Imaging: A non-invasive imaging technique used primarily in medical diagnostics to visualize tissue structures.
- Photoacoustic Microscopy: Offers high-resolution images of biological samples by scanning at microscopic levels.
Photoacoustic imaging provides higher contrast images for blood-rich tissues due to stronger optical absorption by blood compared to surrounding tissues.
The advancement of these techniques often involves complex mathematical models and computational methods to accurately interpret the data. In particular, the reconstruction algorithms employed in photoacoustic imaging rely heavily on solving inverse problems, where measurements of acoustic waves are used to infer the optical properties of tissues. As a general approach, the photoacoustic wave equation is fundamentally described by:\[abla^2 p - \frac{1}{v^2} \frac{\text{d}^2 p}{\text{d}t^2} = -\beta\frac{\mu_a}{C_p}\frac{\text{d}H(t)}{\text{d}t}\],where \(p\) is the acoustic pressure, \(v\) is the speed of sound, \(\mu_a\) is the optical absorption coefficient, and \(H(t)\) is the heat source defined by the absorbed optical energy. Understanding these equations empowers you to grasp the technical intricacies of this blossoming field.
Photoacoustic Spectroscopy and Imaging
The integration of light and sound in photoacoustic spectroscopy and imaging opens up a wide array of possibilities across various scientific fields. It allows for non-invasive and highly sensitive analysis of structures and compositions by utilizing the benefits of both optical and acoustic properties.
Photoacoustic Spectroscopy Techniques
Photoacoustic spectroscopy leverages the photoacoustic effect to determine the absorption spectrum of a sample. The light-induced sound waves give insights into the chemical and physical properties of the material. Here are some prominent techniques:
- Gas Photoacoustic Spectroscopy: Useful in detecting trace gases for environmental monitoring.
- Solid Sample Analysis: Applied in material science to analyze metals and semiconductors.
- Liquid Spectroscopy: Used for detecting pollutants in water through absorption characteristics.
Consider the photoacoustic signal equation used in spectroscopy: \[S = C \cdot P_0 \cdot \alpha \cdot \/ e^{-\alpha z} \cdot f(t)\],where \(S\) denotes the signal amplitude, \(C\) is the calibration constant, \(P_0\) is the incident laser power, \(\alpha\) is the absorption coefficient, \(z\) is the penetration depth, and \(f(t)\) represents the time-dependent modulation function. The equation forms the basis for interpreting spectroscopy data and advancing precision in the study of diverse materials.
Example: In environmental science, photoacoustic spectroscopy is employed to measure air quality by detecting gases such as CO2 and CH4 in the atmosphere. Here, the laser beam passes through the air sample, and the generated acoustic signal is measured to identify the presence and concentration of specific gases.
Advancements in Photoacoustic Imaging
Photoacoustic imaging is at the cutting edge of biomedical diagnostics, offering non-invasive visualization of tissues and organs. Recent advancements in this field focus on enhancing image resolution and depth, making it vital in medical applications.
Current advancements have honed in on:
- High-Resolution Imaging: Developments in laser technology have led to finer imaging capabilities.
- Deep Tissue Penetration: Improved photoacoustic sensors facilitate the exploration of deeper tissue structures.
- Contrast Agents: Innovative contrast agents enhance the clarity of photoacoustic images, particularly in highlighting regions of interest.
The math behind photoacoustic imaging involves reconstructive algorithms based on signals acquired from multiple angles. The universal back-projection algorithm is one such method, represented by: \[p(\textbf{r},t) = \int_{{S}} p(\textbf{r}_s,t - \frac{R}{c})\frac{dA}{R}\], where \(p(\textbf{r},t)\) is the pressure at a point \(\textbf{r}\), \(\textbf{r}_s\) is the detector position, \(R\) is the distance from source to detector, \(c\) is the speed of sound, and \(dA\) denotes the differential area on the detector surface. This mathematical approach underscores the complexity and the critical role of computational techniques in translating acoustic data to vivid medical imagery.
The use of nanoparticles as contrast agents in photoacoustic imaging can significantly amplify the specificity and sensitivity of diagnostic results, providing clearer images of biological tissues.
Applications of Photoacoustics in Engineering
The intersection of photoacoustics and engineering opens a gateway to pioneering applications that revolutionize diverse technical fields. As you explore its expansive uses, you'll see its transformative impact, particularly in mechanical engineering.
Innovative Uses in Mechanical Engineering
In mechanical engineering, photoacoustics is a powerful tool that enhances various measurement and diagnostic techniques. Its ability to convert light into sound provides unique advantages in testing and material analysis.
- Non-Destructive Testing (NDT): Photoacoustic methods can identify structural defects without causing harm to the components being analyzed.
- Material Characterization: By analyzing the acoustic response, it's possible to determine material properties such as stiffness, density, and porosity.
- Thermal Conductivity Measurements: Utilizes the thermal response for precise measurements of thermal conductivity and diffusive properties.
Photoacoustic techniques can help detect tiny imperfections in metals and composites that traditional methods might overlook, thus ensuring better quality control.
Example: In aerospace engineering, photoacoustic systems are employed to inspect turbine blades for micro-cracks. Light is directed onto the blade surfaces, and the generated acoustic signals help detect stress points that are invisible to the naked eye.
The use of photoacoustic emission in mechanical engineering incorporates advanced modeling techniques. These focus on understanding wave propagation in various media, which is critical for accurate detection and analysis. Equation models like:\[abla^2p(\mathbf{r},t) - \frac{1}{v^2} \frac{\partial^2 p(\mathbf{r},t)}{\partial t^2} = \beta \frac{\partial}{\partial t}Q(\mathbf{r},t)\],where \(p(\mathbf{r},t)\) is the pressure field, \(v\) is the sound speed, and \(Q(\mathbf{r},t)\) is the heat source term, are instrumental in understanding how photoacoustic phenomena can be applied to solve complex engineering problems. These deep insights can lead to innovations that transform the field.
Future Applications of Photoacoustics in Engineering
The future of photoacoustics in engineering is promising, with numerous potential applications emerging as research in this field expands. This technology could revolutionize various sectors within engineering by offering enhanced precision and novel capabilities.
- Smart Infrastructure: Employing photoacoustic sensors to monitor the integrity of bridges and buildings in real-time.
- Advanced Prosthetics: Using photoacoustic systems to improve the quality and adaptability of prosthetic devices through better material understanding.
- Robotic Systems: Integrating photoacoustic principles to enhance the sensory capabilities of robots, aiding in navigation and interaction with complex environments.
Exploring the potential of photoacoustic sensors emphasizes their utility in the Internet of Things (IoT) framework for smart cities. These sensors can continuously monitor environmental changes or structural health, sending real-time data to control systems. The key to implementing such systems lies in miniaturizing photoacoustic components and enhancing signal processing capabilities to efficiently analyze large amounts of data. Techniques derived from Maxwell's equations are promising in this domain, particularly in optimizing sensor arrays for better sensitivity and accuracy.This technological convergence suggests a future where photoacoustic-enabled devices aren't just supplementary but become integral to the functioning of advanced engineering systems.
photoacoustics - Key takeaways
- Photoacoustics: The study of sound waves generated by the absorption of light, resulting in thermal expansion and sound wave generation.
- Photoacoustic Effect: The conversion of absorbed light energy into acoustic waves due to rapid thermal expansion.
- Photoacoustic Techniques: Methods such as photoacoustic spectroscopy and imaging that utilize the photoacoustic effect for analysis and visualization.
- Photoacoustic Spectroscopy: A technique used for chemical analysis by examining acoustic waves generated from light absorption in gases, solids, or liquids.
- Photoacoustic Imaging: A non-invasive imaging method used in medical diagnostics to visualize tissue structures with high contrast.
- Applications in Engineering: Utilized in non-destructive testing, material characterization, and innovative applications in smart infrastructure and prosthetics.
Learn faster with the 12 flashcards about photoacoustics
Sign up for free to gain access to all our flashcards.
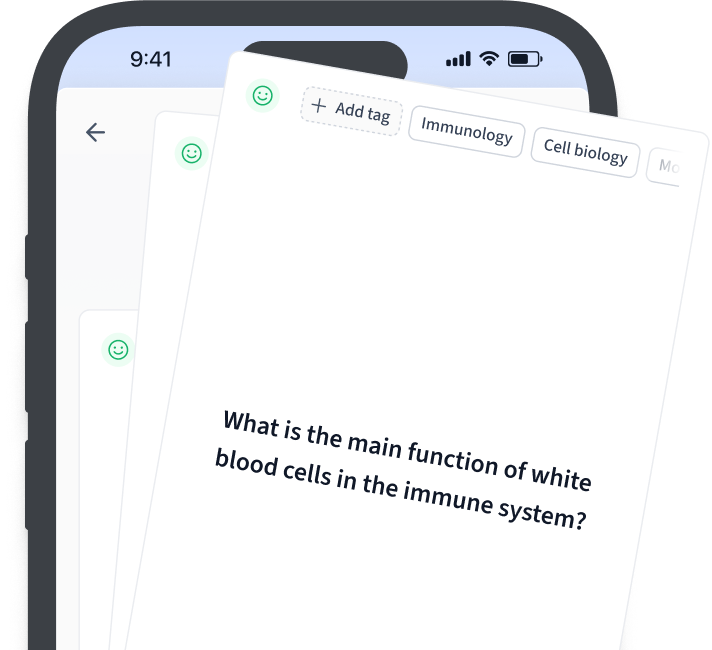
Frequently Asked Questions about photoacoustics
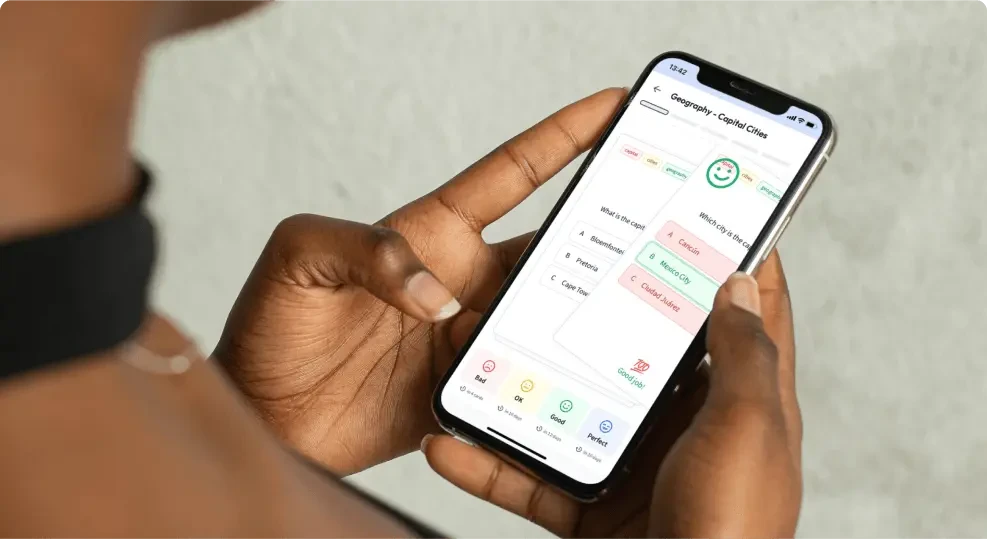
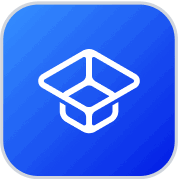
About StudySmarter
StudySmarter is a globally recognized educational technology company, offering a holistic learning platform designed for students of all ages and educational levels. Our platform provides learning support for a wide range of subjects, including STEM, Social Sciences, and Languages and also helps students to successfully master various tests and exams worldwide, such as GCSE, A Level, SAT, ACT, Abitur, and more. We offer an extensive library of learning materials, including interactive flashcards, comprehensive textbook solutions, and detailed explanations. The cutting-edge technology and tools we provide help students create their own learning materials. StudySmarter’s content is not only expert-verified but also regularly updated to ensure accuracy and relevance.
Learn more