Jump to a key chapter
Respiratory Biomechanics Definition
Understand the fundamental concept of Respiratory Biomechanics, a branch of biomechanics focusing on how biological and mechanical processes work together during respiration. It provides insights into how the respiratory system operates efficiently.
What is Respiratory Biomechanics?
Respiratory Biomechanics involves the study of forces exerted by respiratory muscles and the resultant motion of lung structures. This field examines:
- Muscle contractions involved in inhalation and exhalation
- Elastic properties of the lungs and thoracic cavity
- Airflow dynamics within the respiratory tract
- Pressure changes facilitating gas exchange
Definition: Respiratory Biomechanics explores the mechanical functions within the respiratory system, detailing how force and motion contribute to breathing.
Consider the process of inhalation. During this action, the diaphragm contracts, pulling downward and expanding the chest cavity.This expansion results in:
- A decrease in intrapleural pressure
- Increased lung volume
- Air influx into the lungs
Beyond the basics, delve into the role of surfactants, which are substances that reduce surface tension in the lungs. Surfactants are crucial during respiration as they:
- Prevent alveolar collapse
- Ensure easier breathing by reducing work of breathing
- Facilitate uniform inflation of alveoli
Biomechanics of Respiratory System
The study of Biomechanics of the Respiratory System reveals how mechanical principles govern the functioning of our breathing processes. By examining the physical aspects, you get a comprehensive look at how different components of the respiratory system synchronize to facilitate breathing.
Mechanical Components of Breathing
Breathing involves several mechanical components, each playing a crucial role.
- Diaphragm: The primary muscle responsible for inhalation, moving downward to expand the chest cavity.
- Intercostal Muscles: These muscles assist in elevating the ribs, increasing thoracic volume.
- Pleura: A double-layered membrane surrounding the lungs, providing a frictionless surface.
The diaphragm's movement during inhalation causes a chain reaction leading to a pressure difference following Boyle’s Law. The relationship between pressure and volume in a closed system can be expressed as:\[ P_1V_1 = P_2V_2 \]This equation shows how an increase in lung volume results in decreased pressure, drawing air into the lungs.
Imagine the lungs as balloons. When you breathe in, the diaphragm and intercostal muscles expand the chest cavity, similar to pulling on the stem of a balloon. This expansion decreases pressure inside the lungs, and air naturally flows in to equalize the pressure.
Boyle’s Law: A principle stating the inverse relationship between the volume and pressure of a gas in a closed system at constant temperature.
The biomechanics of respiration is not only limited to muscle action and pressure changes. It also involves the concept of lung compliance, a measure of the lungs' ability to stretch and expand.High compliance indicates an easy expansion of the lungs, while low compliance means more effort leads to the same expansion. The equation for lung compliance \( C_L \) is:\[ C_L = \frac{\Delta V}{\Delta P} \]where \( \Delta V \) is the change in lung volume and \( \Delta P \) is the change in pleural pressure. Understanding compliance helps in diagnosing and treating respiratory conditions.
Remember that while the diaphragm does most of the work during quiet breathing, other muscles play a larger role during deep or forceful inhalation.
Engineering Principles in Respiratory Biomechanics
Engineering principles apply significantly in Respiratory Biomechanics, highlighting the interaction between biological structures and mechanical forces. Understanding these principles offers insights into the efficient functioning and resilience of the respiratory system.
Engineering Concepts in Breathing
Breathing mechanics incorporate several engineering concepts that mimic mechanical systems.
- Elasticity: Lungs possess elastic properties that allow stretching and recoiling.
- Fluid Dynamics: The study of airflow through the respiratory tract is akin to fluid flow in engineering systems.
- Pressure Systems: The thoracic cavity operates as a pressure system, driving air movement based on pressure differences.
Elasticity in respiratory biomechanics refers to the lung tissue's capacity to return to its original shape after being stretched, a critical property for effective breathing.
Consider the heart-lung machine used in surgeries. This device illustrates the principle of fluid dynamics by replacing the lung's function, channeling blood in a manner similar to air moving through the bronchial passages.When analyzing fluid flow, Bernoulli’s equation can be useful:\[ P + \frac{1}{2} \rho v^2 + \rho gh = \text{constant} \]This equation demonstrates the relationship between pressure, velocity, and height in fluid dynamics.
In understanding pressure systems, consider that breathing in (inspiration) and breathing out (expiration) are driven by pressure changes represented by:\[ P_\text{atm} = P_\text{alv} + P_\text{pl} \]where \( P_\text{atm} \) is atmospheric pressure, \( P_\text{alv} \) is alveolar pressure, and \( P_\text{pl} \) is pleural pressure. Effective respiration relies on the precise regulation and interaction of these pressures.
Delving deeper into the concept of elasticity, Hooke’s Law plays a vital role in depicting how tissues respond to stress. The equation for Hooke’s Law is:\[ F = -kx \]where \( F \) is the force applied, \( k \) is the stiffness of the tissue, and \( x \) is the extension or compression. This relationship describes how lung tissue accommodates varying volumes of air, demonstrating its elastic limits and capabilities.
Think of lung elasticity like a spring — it stretches when you inhale and returns to its initial state when you exhale, ensuring consistent airflow.
Respiratory Biomechanics Applications
Applications of Respiratory Biomechanics are pivotal in medical and engineering fields. They provide innovative approaches to analyze and enhance respiratory functions, leading to advancements in healthcare and technology.
Respiratory Biomechanics Analysis Techniques
Analyzing the respiratory system through biomechanics involves several sophisticated techniques that reveal detailed insights into how breathing mechanics function:
- Computational Modeling: Uses computer-based simulations to predict respiratory mechanics under various conditions.
- Imaging Technologies: Includes MRI and CT scans, offering detailed views of lung structures and functions.
- Spirometry: Measures lung function by recording the volume and speed of air inhaled and exhaled.
In clinical settings, computational modeling can predict how alterations in airway geometry affect airflow. For instance, a reduction in bronchial diameter can increase resistance, represented mathematically as:\[ R = \frac{8 \eta L}{\pi r^4} \]where \( R \) is the resistance, \( \eta \) is the viscosity of the air, \( L \) is the length of the airway, and \( r \) is the radius. A small decrease in \( r \) significantly increases \( R \), illustrating the impact of airway constriction.
A deeper understanding of biomechanical analysis reveals the importance of analyzing tissue engineering in respiratory biomechanics. Tissue engineering focuses on manipulating the extracellular matrix (ECM) to enhance lung tissue regeneration. Recent advances show how bioengineering scaffolds can mimic natural ECM, leading to better outcomes in lung repair.
- Scaffolds provide structural support for tissue growth.
- They aid in cell adhesion and differentiation.
- Scaffolds degrade over time, integrating with host tissue.
Techniques in Respiratory Biomechanics
Various techniques in the field of Respiratory Biomechanics are employed to enhance understanding and application in both medical and research fields:
- Finite Element Analysis (FEA): Evaluates stress and strain distribution in lung tissues.
- Mechanical Ventilation Modeling: Simulates the mechanical aspects of assisted breathing devices.
- Acoustic Analysis: Studies sound waves to assess airway obstructions or abnormalities.
Acoustic analysis offers a non-invasive technique to detect early signs of airway obstructions, making it an important diagnostic tool.
Finite Element Analysis (FEA): A computational technique that divides a large system into smaller finite elements, which are easier to calculate, allowing detailed analysis of complex structures like lung tissues.
Using FEA, you can model how stress spreads throughout lung tissue during different breathing phases, predicting potential points of failure or overload. These insights help in improving devices like mechanical ventilators, ensuring optimal assistance to patients without risking tissue damage.
Consider a mechanical ventilator delivering air at a constant flow rate. By adjusting variables like flow rate and pressure, you can use mechanical ventilation modeling to assess how changes affect the patient’s lungs. This can be mathematically represented as:\[ Q = A \times V \]where \( Q \) is the flow rate, \( A \) is the cross-sectional area, and \( V \) is the velocity of airflow.
respiratory biomechanics - Key takeaways
- Respiratory Biomechanics Definition: It explores how mechanical functions within the respiratory system, detailing force and motion, contribute to breathing.
- Engineering Principles: Includes elasticity, fluid dynamics, and pressure systems demonstrating the application of mechanical laws to the respiratory system.
- Applications in Medical Field: Respiratory biomechanics plays a pivotal role in medical advancements, enhancing respiratory function analysis and healthcare technology.
- Analysis Techniques: Techniques such as computational modeling, imaging technologies, and spirometry provide insights into respiratory mechanics.
- Finite Element Analysis (FEA): A computational technique used to evaluate stress and strain distribution in lung tissues.
- Mechanics of Breathing: Involves components like the diaphragm and intercostal muscles following mechanical principles for efficient gas exchange.
Learn faster with the 12 flashcards about respiratory biomechanics
Sign up for free to gain access to all our flashcards.
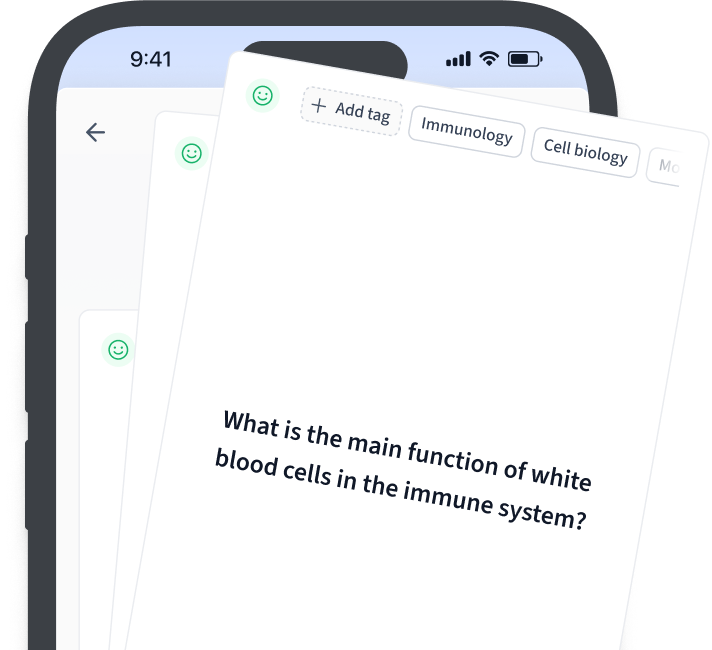
Frequently Asked Questions about respiratory biomechanics
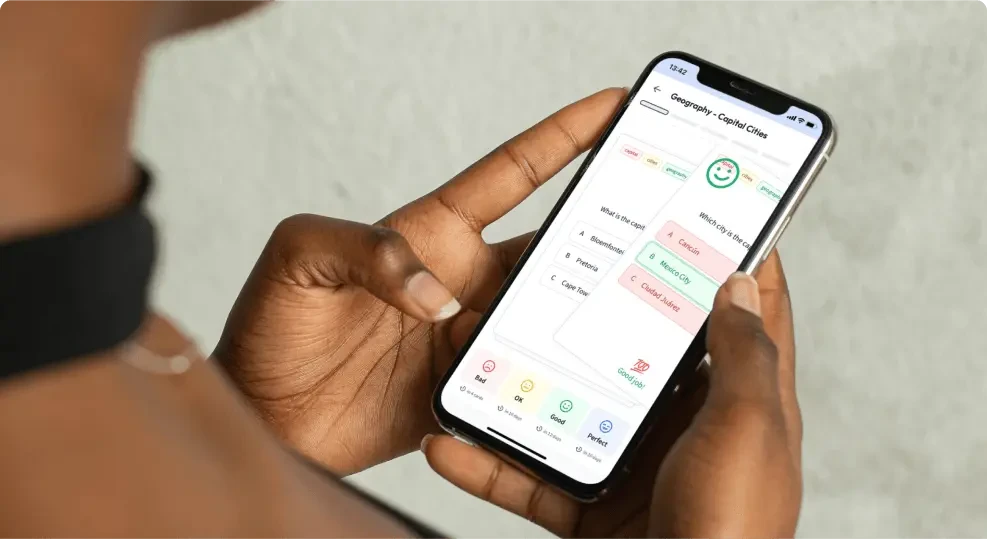
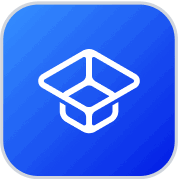
About StudySmarter
StudySmarter is a globally recognized educational technology company, offering a holistic learning platform designed for students of all ages and educational levels. Our platform provides learning support for a wide range of subjects, including STEM, Social Sciences, and Languages and also helps students to successfully master various tests and exams worldwide, such as GCSE, A Level, SAT, ACT, Abitur, and more. We offer an extensive library of learning materials, including interactive flashcards, comprehensive textbook solutions, and detailed explanations. The cutting-edge technology and tools we provide help students create their own learning materials. StudySmarter’s content is not only expert-verified but also regularly updated to ensure accuracy and relevance.
Learn more