Jump to a key chapter
Rotordynamics Definition
In engineering, the study of rotordynamics explores the behavior and dynamics of rotating machinery. This includes turbines, engines, and compressors among other systems. Understanding rotordynamics is essential for designing machinery that operates reliably and efficiently. Machinery that experiences rotational forces often requires precise calculations and considerations within rotordynamics to ensure performance and safety.
What is Rotordynamics?
Rotordynamics is a specialized branch of mechanical engineering focused on analyzing the dynamic behavior of rotating systems or rotors. These systems are crucial for various applications, including turbines in power plants and aircraft engines. Rotordynamics examines how different forces, such as imbalance, gyroscopic effects, and damping, affect a rotor during operation. A fundamental goal in this field is to predict and mitigate undesired phenomena like vibrations and resonances, which can lead to mechanical failure. By applying the principles of rotordynamics, engineers can optimize the design and operation of rotating machinery to enhance longevity and efficiency.
Rotordynamics refers to the study of the behavior of rotating parts, known as rotors, influenced by forces like centrifugal and gyroscopic forces, damping, and stiffness.
Consider a jet engine, a sophisticated piece of machinery where rotordynamics is crucial. Engineers must analyze the rotor's vibration modes and critical speeds to avoid resonance, which could lead to catastrophic failure if unaddressed. For example, if a rotor operates at a speed where its natural frequency matches the operational frequency, known as the critical speed, the resulting resonance could amplify vibrations and pose significant risks.
The critical speed is a pivotal concept in rotordynamics, representing the speed at which a rotor's natural frequency aligns with its operational speed.
Key Concepts in Rotordynamics
Understanding core concepts in rotordynamics is essential for engineers dealing with rotating machinery. These concepts ensure the assessment and optimization of these systems' performance as follows:
- Critical Speed: The speed at which resonance occurs, which is avoided in design.
- Stiffness and Damping: Affects how vibrations are absorbed and mitigated in a system.
- Imbalance: A condition where the rotor's mass is unevenly distributed, causing vibrations.
A significant aspect of rotordynamics involves the study of vibrational modes and how they interact within a system. Of particular interest is the role of gyroscopic effects, which becomes prominent when a rotor's axis is subjected to precession. Gyroscopic effects ensure stability in directional control devices like gyroscopes. When analyzing rotor-bearing systems, engineers must account for these effects, often using advanced computational models. In turbomachinery, this entails using finite element methods to predict potential vibratory behavior under various operational conditions. Another concept is the nonlinear phenomena in rotordynamics. Nonlinearity occurs in rotor systems due to factors like large amplitudes of motion, which standard linear analysis may not appropriately address. By employing more complex mathematical approaches, such as perturbation methods or bifurcation analysis, engineers can accurately predict and mitigate these nonlinear responses. Understanding these intricate interactions helps in ensuring design robustness and operational integrity, especially for rotating systems facing extreme conditions.
Rotordynamics Explained
In understanding rotating machinery, rotordynamics is a critical branch of mechanical engineering that focuses on the dynamic behavior of rotors. This knowledge is vital for ensuring machinery runs smoothly, safely, and efficiently. Missteps in rotordynamics can lead to equipment failure, underscoring its importance in design and maintenance. The discipline encompasses various principles such as vibration analysis, dampening techniques, and critical speed determination. These concepts help in preempting mechanical issues associated with forces that act on rotors.
Importance of Rotordynamics in Mechanical Engineering
The significance of rotordynamics in mechanical engineering cannot be overstated, especially considering its applications in various mechanical systems. At its core, rotordynamics helps in:
- Vibration Reduction: By understanding rotor dynamics, engineers can design systems that minimize harmful vibrations.
- Operational Integrity: Ensures that machinery operates within safe and optimal parameters.
- Wear and Tear Minimization: Reducing vibrations leads to less mechanical wear over time.
Consider a steam turbine in a power plant. Engineers must analyze the rotational dynamics carefully to ensure that operational conditions do not coincide with the turbine's critical speed. Operations at this speed can lead to resonance, resulting in excessive vibrations and potential failure. By calculating the critical speed ahead of time, precise measures can be implemented to avoid such issues.
Remember, the avoidance of critical speeds is paramount in rotordynamics to maintain the durability and reliability of the system.
While fundamental principles of rotordynamics are essential, advanced applications involve studying phenomena such as stability analysis and transient responses. For instance, in aircraft engines, gyroscopic effects and complex rotor interactions become significant. Advanced computational models, such as finite element analysis, are often employed to predict these intricate interactions. In addition, the study of nonlinear behavior in rotordynamics offers more depth. It considers conditions where the assumptions of linear elasticity and small deformations no longer hold. Nonlinearities may arise from material behavior, large deformations, or multiphysics interactions, requiring sophisticated techniques, such as bifurcation theory, for a thorough understanding.
Applications of Rotordynamics
Rotordynamics extends far beyond theoretical concepts, proving crucial in practical engineering applications. The study is used in the design, analysis, and operational optimization of many systems. Here are a few areas where rotordynamics plays a pivotal role:
- Power Generation: Turbines and generators rely on sound rotordynamic principles to ensure efficiency and prevent operational downtime.
- Aerospace: In aircraft engines, understanding rotor dynamics is essential to avoid destructive vibrations at high speeds.
- Automotive Industry: Applications include balancing and analyzing crankshafts and gears to enhance performance and longevity.
Rotordynamic Analysis
The study of rotordynamic analysis is crucial for understanding the performance and safety in machinery featuring rotating parts. By examining vibrations, interactive forces, and operating conditions, engineers can predict potential issues and optimize designs for efficiency.
Tools for Rotordynamic Analysis
In the realm of rotordynamic analysis, several tools facilitate the comprehensive study of rotating systems. These tools help engineers visualize and predict the behavior of rotors under various conditions. Key tools include:
- Finite Element Analysis (FEA): A computational tool that breaks down complex systems into smaller parts or elements, allowing for detailed evaluation of vibrations and stresses.
- Modal Analysis: Used to determine the vibration modes of a system, identifying natural frequencies and potential resonance issues.
- Balance Quality Tools: Instruments that measure and correct mass imbalance in rotating machinery to minimize vibrations.
Tool | Function |
FEA | Analyzes complex systems through simulation |
Modal Analysis | Identifies vibration modes |
Balance Quality | Corrects mass imbalances |
Finite Element Analysis (FEA) is a simulation method that breaks down complicated models into smaller, simpler parts to study internal dynamics accurately.
Imagine you are examining a rotor within a car engine. Using FEA, you can simulate the effects of imbalance at high speeds, allowing you to adjust design parameters and avoid efficiency loss or parts damage.
Tools like FEA and Modal Analysis save engineers significant time and resources by precisely predicting how equipment will perform under stress and operational pressures.
Process of Rotordynamic Analysis
Conducting a comprehensive rotordynamic analysis involves several key processes, each crucial in evaluating the dynamics of a rotor:
- Data Collection: Gather necessary data on rotor dimensions, material properties, and operational parameters.
- Modeling: Develop mathematical models representing the rotational system using formulas such as \( M\ddot{x} + C\dot{x} + Kx = F(t) \).
- Simulation: Utilize computational tools to simulate the rotor's behavior under different conditions.
- Analysis: Examine simulation results to identify critical speeds and areas of potential resonance.
- Implementation: Apply findings to improve the design, increase efficiency, and mitigate risks.
A deeper exploration into rotordynamic analysis introduces the concept of transient analysis, which examines non-steady-state situations such as start-up and shutdown conditions. These phases are critical because, during transient moments, the rotor might cross multiple critical speeds, leading to amplified vibrations. Engineers often apply complex mathematical techniques like the Runge-Kutta methods in transient simulations to precisely determine potential risks and stable operating ranges. By incorporating such in-depth analysis, engineers can tailor components to safely withstand dynamic stresses during these transitional moments.
Machinery Vibration and Rotordynamics
Rotordynamics is a vital field in understanding machinery vibration. Vibration in rotating machinery can lead to damage and inefficiencies. The principles of rotordynamics help engineers analyze and reduce these vibrations to improve machinery longevity and performance. By focusing on rotordynamic principles, you can effectively manage and design rotating systems that operate seamlessly.
Relationship Between Vibration and Rotordynamics
The relationship between vibration and rotordynamics is fundamental in understanding the behavior of rotating machinery. Here are key aspects of this relationship:
- Rotordynamics provides the framework for predicting vibrational behavior in machinery like turbines, engines, and compressors.
- It focuses on analyzing forces such as imbalance and resonance which cause vibrations.
- Through mathematical modeling, engineers can anticipate critical speeds that might amplify vibrations.
- M represents the mass of the rotor.
- C is the damping coefficient.
- K stands for stiffness.
- F(t) depicts external forces acting on the system.
Vibration in the context of rotordynamics refers to the oscillatory motion of rotating components, which can lead to excessive wear and operational failure if not properly managed.
Consider an industrial fan. This fan experiences high levels of vibration due to its rotation. By applying rotordynamic principles and ensuring proper balance, engineers can reduce these vibrations significantly, leading to quieter operation and prolonged equipment life.
Managing vibration through rotordynamics not only extends the life of machinery but also increases efficiency and safety.
Rotordynamics Techniques to Manage Machinery Vibration
There are several techniques rooted in rotordynamics used to manage and mitigate machinery vibration effectively. These techniques include:
- Dynamic Balancing: This technique involves adjusting the distribution of mass around the rotor to eliminate imbalances causing vibration.
- Damping Control: Implementing components or materials designed to absorb and diminish vibratory energy.
- Tuning Critical Speeds: Adjusting design parameters to shift the operational speed range away from critical (resonant) speeds.
Advanced computational techniques like finite element analysis (FEA) play a significant role in rotordynamic simulations, allowing engineers to visualize and predict vibratory behaviors in complex machinery. With FEA, engineers can experiment with different design possibilities, observing how changes affect vibrations without actual physical prototypes. The application of active control systems also represents a modern approach. These systems use sensors and actuators to counteract vibrations in real-time, adjusting operational parameters dynamically to maintain stability and efficiency.
Rotordynamics Theory
The rotordynamics theory is essential in the design and operation of rotating machinery, focusing on various factors that influence rotor behavior. This includes analyzing forces like imbalance, gyroscopic effects, and dynamic stability. Understanding these elements is critical for optimizing machinery performance and preventing failures.
Fundamental Theories in Rotordynamics
The foundation of rotordynamics consists of several key theories and principles that guide engineers in the design and analysis of rotating systems. Some of these fundamental concepts include:
- Critical Speed: The speed at which the rotor's natural frequency coincides with the operational frequency, potentially causing resonant vibrations.
- Gyroscopic Effects: These effects arise due to the precession of rotational axes, impacting the dynamic behavior of the rotor.
- Imbalance Forces: Occur when the rotor's mass distribution is not symmetrical, leading to lateral vibrations.
Consider the case of an industrial gas turbine. If its operational speed crosses the critical speed, the rotor may experience severe vibration due to resonance. Using rotordynamic principles, engineers can predict these speeds and design systems to avoid them, thereby preventing potential damage and enhancing safety.
A deeper understanding of rotordynamics also involves studying the effects of different types of damping in a rotor system. Damping can be linear or nonlinear, with each type playing a distinct role in system behavior. Linear damping is often represented as a constant in the equations of motion, whereas nonlinear damping may include complex components that relate to velocity squared or higher orders. Understanding how these components interact is vital when simulating real-world rotor behaviors, especially in systems subject to variable loading conditions.
Advanced Concepts in Rotordynamics Theory
Building upon fundamental theories, advanced concepts in rotordynamics delve into complex phenomena that can affect more sophisticated machinery systems. These advanced concepts include:
- Nonlinear Dynamics: Involves the study of rotor systems that do not behave linearly, requiring advanced mathematical approaches for accurate modeling.
- Stability Analysis: Assessing the rotor's ability to maintain its motion without diverging over time.
- Transient Analysis: Focuses on the rotor's behavior during changes in speed, including start-up and shut-down phases.
Advanced rotordynamic techniques often use finite element methods to accurately simulate and predict vibrational behaviors in complex systems.
rotordynamics - Key takeaways
- Rotordynamics Definition: The study of rotating machinery behavior and dynamics, crucial for design and safety.
- Key Concepts in Rotordynamics: Critical Speed, Stiffness and Damping, and Imbalance are core concepts affecting rotor behavior.
- Rotordynamic Analysis: Involves using tools like Finite Element Analysis and Modal Analysis to study rotor dynamics.
- Machinery Vibration and Rotordynamics: Understanding vibrations through rotordynamics is vital for reducing damage and enhancing efficiency.
- Rotordynamics Techniques: Dynamic Balancing, Damping Control, and Tuning Critical Speeds are methods to manage machinery vibrations.
- Rotordynamics Theory: Encompasses theories of Critical Speed, Gyroscopic Effects, and Imbalance Forces essential in designing rotating systems.
Learn faster with the 10 flashcards about rotordynamics
Sign up for free to gain access to all our flashcards.
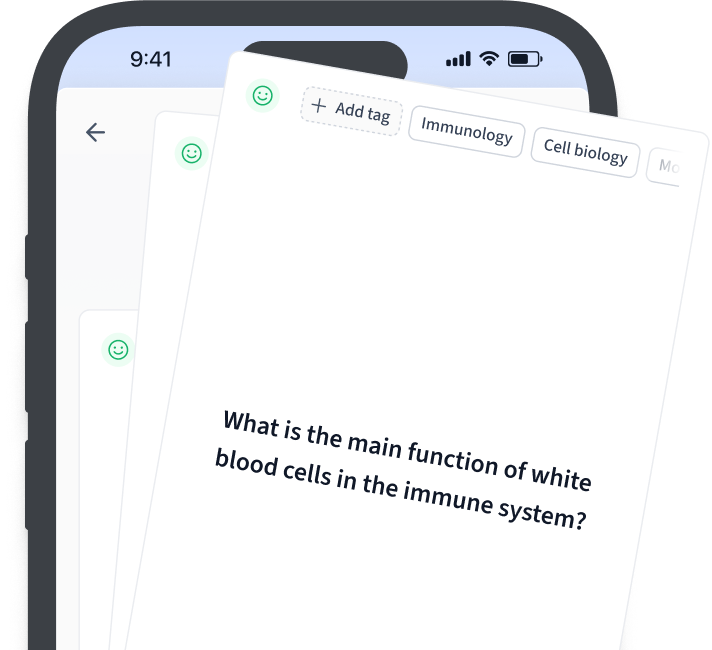
Frequently Asked Questions about rotordynamics
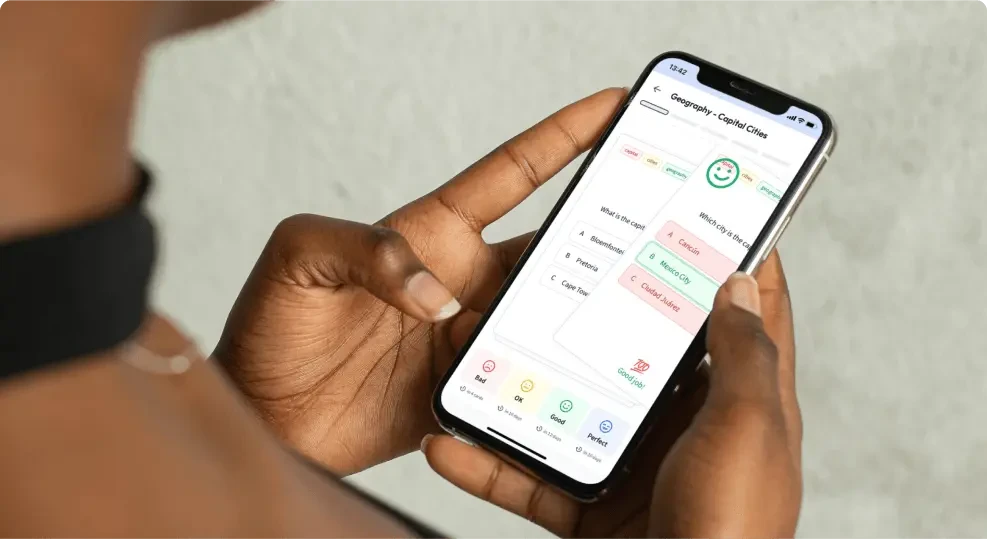
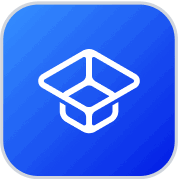
About StudySmarter
StudySmarter is a globally recognized educational technology company, offering a holistic learning platform designed for students of all ages and educational levels. Our platform provides learning support for a wide range of subjects, including STEM, Social Sciences, and Languages and also helps students to successfully master various tests and exams worldwide, such as GCSE, A Level, SAT, ACT, Abitur, and more. We offer an extensive library of learning materials, including interactive flashcards, comprehensive textbook solutions, and detailed explanations. The cutting-edge technology and tools we provide help students create their own learning materials. StudySmarter’s content is not only expert-verified but also regularly updated to ensure accuracy and relevance.
Learn more