Jump to a key chapter
Thermodynamics in Manufacturing Overview
Understanding the principles of thermodynamics is crucial in the field of manufacturing. It offers insights into how energy is converted and used during production processes. As you delve deeper into this subject, you'll learn about the key laws that govern energy transformations and explore the real-world applications of these concepts in various manufacturing contexts.
Thermodynamic Definitions in Manufacturing
Thermodynamics in manufacturing involves the study of energy transformations and the relationship between heat, work, and internal energy. This area is key in optimizing processes, reducing waste, and improving efficiency. Below are some core terms you need to know:
- System: In thermodynamics, a system is a defined portion of the universe that is being studied. It can be open, closed, or isolated.
- Surroundings: Everything external to the system is considered the surroundings.
- Internal Energy (U): The total energy contained within a system.
- Enthalpy (H): Represented as the sum of the internal energy and the product of pressure and volume, it is given by the formula: H = U + PV
- Entropy (S): A measure of disorder or randomness in a system, often linked with the second law of thermodynamics.
Consider a steam engine, a classic example of an open system in manufacturing. It takes in water and fuel, converts chemical energy into mechanical work, and releases exhaust. During this process, the engine's internal energy changes, and calculations involving enthalpy and entropy can help optimize its efficiency.
When studying thermodynamics, remember that laws and definitions are interconnected. Mastering the basics will help you tackle more complex topics in manufacturing.
The concept of entropy can be particularly fascinating in manufacturing. In an ideal process, entropy might remain constant, but in the real world, entropy tends to increase due to various inefficiencies. This is represented by the inequality: ΔS ≥ 0 This principle guides engineers to design manufacturing processes that minimize entropy increases, thereby improving energy efficiency. For example, in refrigeration cycles, energy transfer and entropy changes are calculated to maximize the efficiency of cooling processes. By understanding the entropy changes in these processes, you can devise strategies to reduce unnecessary energy losses and enhance overall performance.
Key Thermodynamic Principles in Manufacturing
Thermodynamic principles play a vital role in manufacturing. They help optimize energy usage, which can lead to cost savings and sustainability. This section will address the fundamental laws of thermodynamics and their implications in the manufacturing sector.
First Law of Thermodynamics in Manufacturing
The First Law of Thermodynamics, also known as the law of energy conservation, states that energy cannot be created or destroyed; it can only change from one form to another. This principle is critical in ensuring that energy inputs in manufacturing processes are efficiently converted to useful work.In manufacturing, this law can be represented as: \(\text{{Change in Internal Energy}} (\triangle U) = \text{{Heat added to the system}} (Q) - \text{{Work done by the system}} (W)\)Key applications include:
- Calculating energy efficiency: By measuring the work done and comparing it to the energy input, you can determine system efficiency.
- Designing energy-efficient processes: Minimizing energy loss through effective insulation and waste heat recovery.
Internal Energy (\text{U}): The total energy contained within a system, influenced by temperature, pressure, and volume.
Consider a gas turbine used in power plants. According to the first law, the heat energy supplied to the turbine is converted into mechanical energy with some losses as heat, which must be accounted for. If \text{Q} = 250 \text{Joules} and \text{W} = 200 \text{Joules}, The change in internal energy is \(\triangle U = 250 \text{Joules} - 200 \text{Joules} = 50 \text{Joules}\)
Remember, the First Law is about balancing energy, much like balancing your checkbook!
Second Law of Thermodynamics in Manufacturing
The Second Law of Thermodynamics introduces the concept of entropy. It states that in any energy exchange, if no energy enters or leaves the system, the potential energy of the state will always be less than that of the initial state. This law emphasizes the inefficiencies and losses that occur during energy transformations in manufacturing. In manufacturing processes, this can be applied by understanding that not all supplied energy is converted into useful work, and some is dissipated as waste heat. Thus, the formula becomes:\(\triangle S = \frac{Q}{T}\)where \(\triangle S\) is the change in entropy, \(Q\) is the heat transferred, and \(T\) is the temperature.Implications include:
- Recognizing inevitable energy degradation: Every manufacturing process has some degree of inefficiency, resulting in increased entropy.
- Improving process efficiencies: By reducing waste and designing processes that minimize entropy production.
- Designing Energy Systems: Engineers use thermodynamic principles to design systems that efficiently convert energy from one form to another.
- Optimizing Performance: By applying the laws of thermodynamics, you can optimize the performance of engines, refrigeration units, and other systems.
- Improving Sustainability: Thermodynamics enables the development of processes that reduce energy consumption and minimize environmental impacts.
- Process Heating: Thermodynamics is used to design systems that provide optimal heating solutions, minimizing energy loss.
- Refrigeration and Cooling: Essential for processes that require precise thermal control, such as in the food industry.
- Material Processing: Ensures processes such as smelting or annealing are energy-efficient, improving product quality and consistency.
- Heat Treatment: Utilized in metals and alloys to alter physical properties, improve hardness, or relieve internal stresses, relying heavily on thermodynamic calculations.
- Refrigeration Systems: Essential for both preservative processes in the food industry and chemical manufacturing, these systems depend on refrigeration cycles like vapor-compression.
- Combustion Engines: Thermodynamics helps optimize the fuel consumption and efficiency of engines in the automotive sector.
- Glass Manufacturing: Thermodynamics is used to control furnace temperatures critically, ensuring the finished products meet quality criteria with minimal energy waste.
- Petroleum Refining: Fractional distillation and cracking processes are governed by thermodynamic principles to maximize yield and efficiency.
- Electronics Cooling: Semiconductor production requires precise thermal management to prevent failures and extend device lifespans.
- Thermodynamics in Manufacturing: Study of energy transformations in manufacturing to optimize processes, reduce waste, and improve efficiency.
- Thermodynamic Principles: In manufacturing, these principles help optimize energy usage, leading to cost savings and sustainability.
- Key Thermodynamic Definitions: System, surroundings, internal energy, enthalpy, and entropy are crucial terms in manufacturing thermodynamics.
- First and Second Laws of Thermodynamics: Essential for ensuring energy balance and recognizing inefficiencies in manufacturing processes.
- Engineering Thermodynamics: Involves analyzing energy systems to design effective and efficient engineering solutions.
- Examples and Applications: Include heat treatment, refrigeration systems, and combustion engines, crucial for process efficiency and cost reduction.
An intriguing implication of the Second Law is in heat engines, such as refrigerators and air conditioners. The second law predicts the maximum possible efficiency of these engines, called the Carnot efficiency. Given by \(1 - \frac{T_c}{T_h}\), where \(T_c\) is the cold reservoir temperature and \(T_h\) is the hot reservoir temperature, this relationship helps in understanding and designing cooling systems that consume less power while maximizing performance. By redesigning manufacturing systems with these principles in mind, significant energy savings can be achieved. This ultimately contributes to more sustainable industrial practices and reduced environmental impacts.
Applied Thermodynamics in Engineering Context
Thermodynamics is essential in engineering as it provides the foundation for analyzing energy systems across various applications. Understanding it empowers you to design effective and efficient engineering systems that meet the demands of modern industry. Let's explore how engineering thermodynamics plays a critical role within this context.
Role of Engineering Thermodynamics
Engineering Thermodynamics helps in understanding the transformation and distribution of energy and its implications in engineering systems. Here’s how it plays a crucial role:
Entropy (S): A measure of the disorder or randomness in a system. It increases with energy dissipation in inefficient processes.
Consider a solar panel system. Thermodynamic principles help determine how efficiently solar energy can be converted into electrical energy by calculating the system’s energy conversion efficiency \(E_{eff}\), using the formula: \[ E_{eff} = \frac{P_{out}}{E_{in}} \] where \(P_{out}\) is the power output and \(E_{in}\) is the energy input.
Thermodynamics is deeply intertwined with material science. The study of the thermodynamic properties of materials dictates their behavior in different conditions, such as stress or high temperatures. In manufacturing, for instance, selecting materials with appropriate thermal properties is crucial for fabricating components that can withstand operational conditions without degrading.
Practical Applications of Thermodynamics in Manufacturing
Thermodynamics influences numerous manufacturing processes where understanding heat and energy transfer can lead to innovation and efficiency. Here are some real-world applications:
First Law of Thermodynamics: This law states that energy within a closed system is conserved. It is fundamental in calculating the energy inputs and outputs in manufacturing processes.
In a chemical manufacturing plant, applying the first law can help calculate the required heat input for reactors. For instance, in endothermic reactions, heat must be provided as input, calculated using \(Q = m \cdot c \cdot \Delta T\), where \(m\) is mass, \(c\) is specific heat capacity, and \(\Delta T\) is the temperature change.
Advanced thermodynamic concepts are applied in cutting-edge manufacturing technologies such as additive manufacturing. Understanding how materials respond at molecular levels during processes like 3D printing is essential for optimizing energy use and ensuring structural integrity.
Manufacturing innovations often stem from applying traditional thermodynamics in novel ways, like using waste heat recovery to cut costs and energy use.
Examples of Thermodynamics in Manufacturing
Thermodynamics is a pivotal component in manufacturing, facilitating energy optimization and efficient process engineering. By leveraging its principles, manufacturing professionals can enhance energy efficiency and reduce operational costs. Let’s explore real-world applications and case studies in thermodynamics within the manufacturing industry.
Real-World Thermodynamics Applications in Manufacturing
Thermodynamics finds extensive use in manufacturing, enabling businesses to streamline processes and save energy. Below are some key applications:
Thermodynamic Cycle: A sequence of processes that returns a system to its initial state, crucial in engines where efficiency is calculated using cycles like the Carnot or Rankine cycle.
Consider a blast furnace used in steel production. Thermodynamics allows you to determine the amount of heat required to reduce ores into metal, especially through chemical reactions. By applying the formula: \[ \Delta H = \int_{T_1}^{T_2} C_p \, dT \] where \(C_p\) is the specific heat at constant pressure, you can calculate the necessary heat input over a temperature range.
In the chemical manufacturing industry, exothermic and endothermic reactions are prominent. Thermodynamics aids in determining reaction feasibility and energy profiles. For example, the Haber process synthesizes ammonia; understanding its thermodynamic efficiency can lead to optimized energy use.By balancing reaction enthalpy \( (\Delta H) \) and entropy \( (\Delta S) \) using the Gibbs free energy formula: \[ \Delta G = \Delta H - T \Delta S \] where \( \Delta G \) indicates process spontaneity, manufacturers can enhance system performance.
Thermodynamics not only optimizes energy use but also contributes to a reduction in waste, complying with stringent environmental standards.
Case Studies of Thermodynamics in Manufacturing
Let’s examine specific case studies where thermodynamics has greatly impacted manufacturing outcomes. These examples reveal how theoretical principles translate to practical improvements:
In the production of photovoltaic panels, thermodynamic assessments determine the optimal temperatures for silicon melting and crystallization. By applying principles like the conservation of energy and entropy calculations, manufacturers increase panel efficiency.Efficiency \[ \eta = \frac{E_{useful}}{E_{input}} \]ensures most energy transitions into electricity with minimal losses.
A remarkable case within eco-friendly manufacturing is waste heat recovery units. Through innovative design, these units capture and repurpose heat otherwise expelled as waste. Utilizing tools like the pinch analysis, engineers optimize heat exchanges across processes, turning potential losses into gains.Such interventions dramatically improve energy profiles and align manufacturing operations with sustainability goals. This approach not only enhances profitability but also reduces environmental footprints, paving the way for future advancements in sustainable manufacturing.
thermodynamics in manufacturing - Key takeaways
Learn faster with the 12 flashcards about thermodynamics in manufacturing
Sign up for free to gain access to all our flashcards.
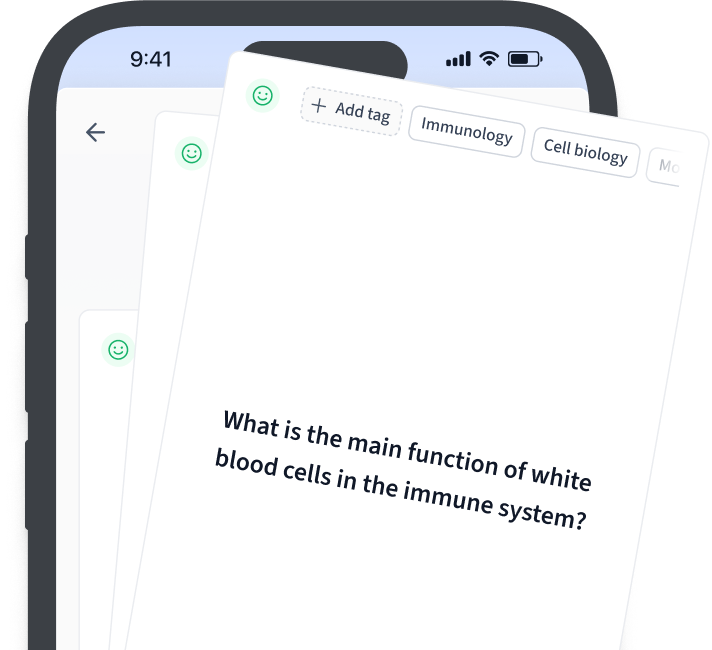
Frequently Asked Questions about thermodynamics in manufacturing
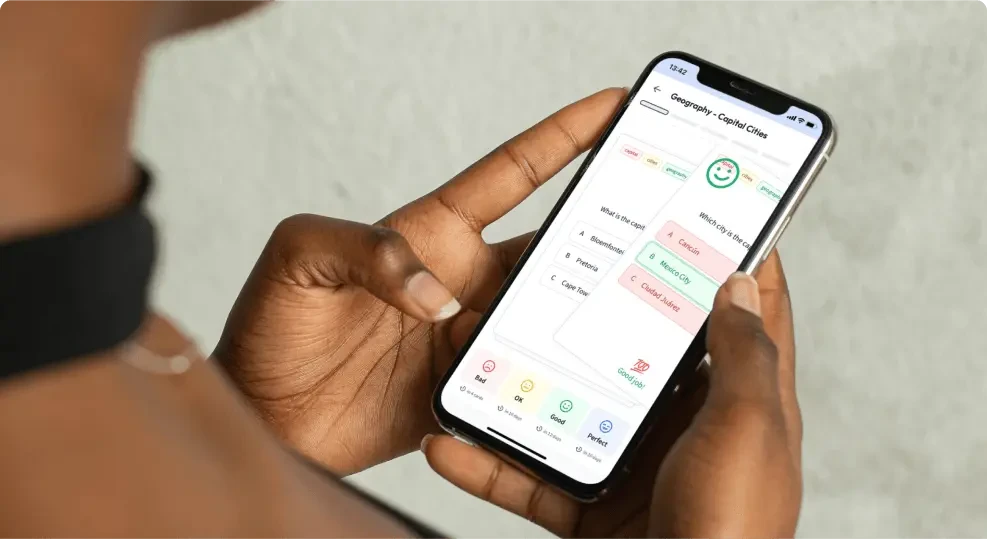
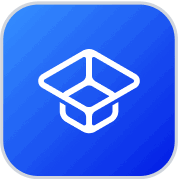
About StudySmarter
StudySmarter is a globally recognized educational technology company, offering a holistic learning platform designed for students of all ages and educational levels. Our platform provides learning support for a wide range of subjects, including STEM, Social Sciences, and Languages and also helps students to successfully master various tests and exams worldwide, such as GCSE, A Level, SAT, ACT, Abitur, and more. We offer an extensive library of learning materials, including interactive flashcards, comprehensive textbook solutions, and detailed explanations. The cutting-edge technology and tools we provide help students create their own learning materials. StudySmarter’s content is not only expert-verified but also regularly updated to ensure accuracy and relevance.
Learn more