Jump to a key chapter
Waveguides and Their Importance in Mechanical Engineering
In mechanical engineering, waveguides are channels or pathways that direct waves, such as electromagnetic waves or sound waves, from one point to another. They are fundamental elements in various applications, playing a crucial role in optimizing signal transmission and minimizing energy loss. Understanding the workings of waveguides allows engineers to design more efficient and effective mechanical systems.
Basic Functionality of Waveguides
Waveguides are designed to confine and direct wave energy efficiently. This is achieved through their unique structure, typically characterized by hollow metallic tubes. Electromagnetic waves, like those used in microwave communication, are effectively controlled within these pathways, ensuring minimal signal degradation.
The cross-sectional shape and size of a waveguide significantly influence its capability to transport waves. The cut-off frequency is a key concept here, defining the minimum frequency required for wave propagation. This frequency is given by the equation:
\[ f_c = \frac{c}{2a} \sqrt{\left(m\right)^2 + \left(n\right)^2} \]
This formula involves:
- \(f_c\): cut-off frequency
- \(c\): speed of light in a vacuum
- \(a\): width of the waveguide
- \(m\) and \(n\): mode indices
Definition: The cut-off frequency is the lowest frequency at which a waveguide mode can propagate.
Example: Consider a rectangular metal waveguide used in a radar system. If the width a is 5 cm, calculate the cut-off frequency for the fundamental mode (\(m,n\) = 1,0).
Solution:\[ f_c = \frac{3 \times 10^8}{2 \times 0.05} \approx 3 \times 10^9\ Hz \]
Applications of Waveguides in Mechanical Engineering
Waveguides are utilized in multiple engineering domains. Their applications include:
- Microwave Engineering: Used in microwave ovens and communications, waveguides efficiently transport microwave energy.
- Optical Engineering: Optical fibers are a type of waveguide that transmit light waves.
- Acoustics: Direct sound waves in musical instruments and architectural acoustics.
Efficiency depends on proper design and material choice, which minimize wave energy dispersion.
Hint: Mechanical engineers often work collaboratively with electrical engineers to optimize waveguide designs for electromagnetic wave applications.
Design Considerations
A waveguide's design is pivotal to its performance. Engineers must consider:
- Material properties: such as conductivity and magnetic permeability.
- Geometric parameters: which influence the cut-off frequency.
- Separation distance: to prevent mode interference.
Mathematical modeling helps in predicting behavior under various conditions.
Deep Dive: A key challenge in waveguide design is the trade-off between size and bandwidth. For higher frequency waves, the waveguide dimensions approach those of the wavelength itself, presenting both design and material challenges. Advanced materials like metamaterials are being researched to mitigate these issues. Metamaterials have unique properties - like a negative index of refraction - which can be leveraged to create compact waveguides with enhanced performance parameters.
Basics of Waveguide Theory
A waveguide is a structure that guides waves, such as electromagnetic waves or sound, with minimal loss of energy by diffraction. Typically, waveguides are hollow metallic or dielectric tubes through which waves are transmitted.
Understanding Waveguide Propagation
Wave propagation within a waveguide primarily depends on the balance between reflection and transmission. A wave reflects off the walls of the waveguide, confining it within a specific path. This is described through the angle of incidence and reflection based on the wave's wavelength and the waveguide's dimensions. By adhering to the principles of total internal reflection, waves travel efficiently over long distances.
Modes of propagation are based on the solution of Maxwell's equations under boundary conditions imposed by the waveguide. Each mode, defined by different field pattern distributions, operates at distinct frequencies. The total number of modes is determined by the waveguide's geometry and the frequency of operation.
Definition: Mode in waveguides refers to the distribution pattern of electromagnetic fields that can propagate through the waveguide. Each mode corresponds to a specific frequency and energy state.
Example: Consider a rectangular waveguide with dimensions a and b. The fundamental transverse electric (TE) mode (TE10) occurs when there is one-half wavelength variation across the larger dimension (a) and no variation along the smaller dimension (b). The cut-off frequency for this mode is given by:
\[ f_c = \frac{c}{2a} \]
Hint: The larger the waveguide, the more possible modes can propagate.
Exploring Waveguide Modes
The modes in a waveguide determine how electromagnetic fields distribute and travel within it. There are two primary mode types: Transverse Electric (TE) and Transverse Magnetic (TM). In TE modes, the electric field is perpendicular to the direction of propagation, whereas in TM modes, the magnetic field is perpendicular.
Each mode has a cutoff frequency, below which it cannot propagate. This cutoff frequency depends on the waveguide's dimensions and can be calculated as:
\[ f_{cmn} = \frac{c}{2} \sqrt{\left(\frac{m}{a}\right)^2 + \left(\frac{n}{b}\right)^2} \]
Mode | Description |
TEmn | Electric field is perpendicular. |
TMmn | Magnetic field is perpendicular. |
Understanding these modes is crucial for designing efficient waveguides tailored to specific applications, such as in radar and satellite communication systems.
Deep Dive: Waveguides can support hybrid modes, such as Transverse Electric and Magnetic (TEM) modes. Unlike TE and TM modes, TEM modes have both electric and magnetic fields perpendicular to the direction of wave propagation. This occurs mainly in coaxial cables where both conductive walls and dielectric mediums are used. The analytical derivation of hybrid modes requires solving modified Maxwell’s equations, leading to complex mathematical models. These modes are not just theoretical but have practical applications in RF and microwave engineering where specific propagation characteristics are desired. Exploring hybrid modes allows engineers to innovate in areas like wireless power transmission and stealth technology.
Optical Waveguide in Communication Systems
In modern communication systems, optical waveguides play a pivotal role in the transmission of light signals over long distances with minimal loss. They are essential components in optical fibers, which are used in telecommunications to send data efficiently and accurately. By guiding light waves, these waveguides help maintain signal integrity and enhance communication speed.
Working Principles of Optical Waveguides
Optical waveguides operate on the principle of total internal reflection. Light travels through a core with a high refractive index, surrounded by a cladding with a lower refractive index. This difference in refractive indices ensures that light is reflected within the core, allowing it to travel efficiently along the waveguide.
The performance of an optical waveguide is described by its mode, which is the pattern in which light travels. These modes are determined by the waveguide's dimensions and the refractive indices of the core and cladding.
The normalized frequency parameter \( V \) is used to determine the number of modes that can propagate through the waveguide, given by the equation:
\[ V = \frac{2\pi a}{\lambda} \sqrt{n_1^2 - n_2^2} \]
- {\lambda}: Wavelength of light
- {a}: Core radius
- {n_1}: Refractive index of core
- {n_2}: Refractive index of cladding
Definition: The normalized frequency parameter \( V \) is a dimensionless quantity that determines the number of modes an optical fiber can support.
Example: For a single-mode fiber operating at 1550 nm with a core radius of 4.5 micrometers, and refractive indices \( n_1 = 1.48 \) and \( n_2 = 1.46 \), calculate the normalized frequency \( V \).
Solution:\[ V = \frac{2\pi (4.5 \times 10^{-6})}{1550 \times 10^{-9}} \sqrt{1.48^2 - 1.46^2} \]\[ V \approx 2.405 \]
Hint: Single-mode fibers typically have a V-number less than or equal to 2.405, ensuring light travels in one mode to reduce interference.
Applications and Benefits in Communication Systems
Optical waveguides are invaluable in telecommunications owing to their ability to transmit data at high speeds and over long distances without significant signal loss. Their applications include:
- Physically lightweight networks with vast bandwidth capabilities.
- Efficient connection methods in metropolitan area networks (MANs).
- Support for internet systems transmitting large quantities of data packets.
By minimizing attenuation, optical waveguides reduce the need for signal amplifiers, thereby lowering operational costs.
Deep Dive: One emerging area of research in optical waveguides is exploring photonic crystal fibers (PCFs). PCFs employ a microstructured air-hole matrix within the core, altering light propagation characteristics compared to conventional fibers. This allows precise control over dispersion properties, enabling advancements in applications like supercontinuum light generation and high-power laser delivery. These novel fibers can potentially revolutionize fields requiring precise control over light-matter interactions, contributing significantly to telecommunications, medical diagnostics, and high-resolution imaging.
Types of Waveguides
Waveguides are specialized structures designed to guide waves such as electromagnetic or acoustic waves. Their ability to direct the wave energy with minimal signal loss makes them crucial in various engineering applications. Different types of waveguides suit different applications based on their structure, design, and material composition.
Features of Rectangular Waveguide
Rectangular waveguides are the most common type used in microwave applications. They are characterized by their rectangular cross-section, guiding waves primarily in the Transverse Electric (TE) and Transverse Magnetic (TM) modes. These waveguides offer advantages in handling high powers and are instrumental in military and telecommunication systems.
The key features include:
- Low loss of energy due to the rigid metallic structure.
- Ability to support multiple modes of propagation.
- Efficient in high-frequency applications.
The cutoff frequency for the fundamental TE mode in a rectangular waveguide is determined by:
\[ f_{c} = \frac{c}{2a} \]
where:
- \(c\): Speed of light in a vacuum
- \(a\): Width of the waveguide
Hint: The larger the dimension of the waveguide, the lower the cutoff frequency, allowing wider frequency bands to be propagated.
Deep Dive: Rectangular waveguides can be combined with dielectric materials to modify their propagation characteristics. For instance, loading the waveguide with a dielectric material can lower the cutoff frequency, enabling it to operate at lower frequencies while maintaining compact dimensions. This principle is highly beneficial in creating compact and efficient antennas used in radar and communication systems. Engineers are constantly exploring new materials, like metamaterials, to enhance waveguide performance and create more versatile designs.
CPW Coplanar Waveguide Characteristics
Coplanar Waveguides (CPW) are another common type of waveguide, used extensively in modern electronics due to their ease of integration with other planar-based components like microstrip circuits. CPWs have a single metallized plane and conductors patterned on the same plane, offering unique advantages.
Key characteristics include:
- Low radiation loss, making them ideal for compact devices.
- Simple fabrication process, which lowers production costs.
- Flexibility to integrate with other RF components on pcb (printed circuit boards).
The characteristic impedance \(Z_0\) of a coplanar waveguide can be calculated using the following formula:
\[ Z_0 = \frac{60}{\sqrt{\varepsilon_{eff}}} \ln \left( 1 + \frac{4h}{W} \right) \]
where:
- \(\varepsilon_{eff}\): Effective dielectric constant
- \(h\): Substrate thickness
- \(W\): Width of the center conductor
Example: Calculate the characteristic impedance of a CPW with \(h = 1.5 \text{mm}\), \(W = 0.5 \text{mm}\), and \(\varepsilon_{eff} = 9.8\).
Solution:\[ Z_0 = \frac{60}{\sqrt{9.8}} \ln \left( 1 + \frac{4 \times 1.5}{0.5} \right) \]\[ Z_0 \approx 31.7 \Omega \]
Practical Applications of Waveguides in Various Industries
Waveguides are essential components in numerous industries due to their ability to efficiently transmit electromagnetic waves over distances with minimal energy loss.
Telecommunications Industry
In the telecommunications sector, waveguides are crucial for high-frequency signal transmission. They act as conduits for microwave signals, essential for satellite communications, mobile networks, and broadcasting systems. Engineers use waveguides to maintain signal integrity across vast distances, ensuring clear and reliable communication.
The efficiency of waveguides reduces the need for signal amplification, lowering operational costs.
- Waveguides are used in antennas to transmit and receive high-frequency signals.
- They are integral to the function of satellite dishes.
- Essential in filtering out unwanted signals in communication devices.
Example: Consider a waveguide used in a microwave communication system that operates at frequencies of 10 GHz. The mode of operation is characterized by the waveguide's dimensions, selecting for optimal transmission and minimal attenuation.
Calculating the wavelength in a typical rectangular waveguide gives:
\[ \lambda_g = \frac{c}{f} \sqrt{1 - \left(\frac{f_c}{f}\right)^2} \]where \( \lambda_g \) is the guided wavelength, \( c \) is the speed of light, \( f \) is the frequency, and \( f_c \) is the cut-off frequency.Hint: Infrequent signal amplification along waveguides helps in reducing energy consumption in long-distance telecommunications applications.
Medical Industry
The medical field uses waveguides for various applications, notably in diagnostic imaging equipment like Magnetic Resonance Imaging (MRI) and therapeutic devices. In these machines, waveguides help in directing radiofrequency waves or light waves, which are essential for diagnosing and treating patients.
- Utilized in MRI for transmitting radiofrequency signals.
- Essential in laser surgery equipment to guide light with precision.
- Applied in microwave ablation techniques.
Definition: Microwave Ablation is a technique used in cancer treatment where microwave energy is emitted through waveguides to destroy tumor tissues.
Automotive Industry
Waveguides find innovative applications in the automotive industry for radar and sensor systems. These systems rely on transmitting and receiving radiofrequency signals to detect obstacles and assist in navigation.
- Radar systems use waveguides to direct microwaves for adaptive cruise control.
- Used in collision avoidance sensors to ensure vehicle safety.
- Integral to blind-spot monitoring systems.
Waveguides in the automotive industry help improve safety and efficiency, indicating upcoming trends such as autonomous vehicles.
Deep Dive: As the automotive industry advances towards fully autonomous vehicles, waveguides are expected to become even more integral. They provide a means to transmit and receive high-frequency signals necessary for 360-degree sensing. This technology enables vehicles to process real-time data and make autonomous driving decisions, transforming waveguides into a cornerstone of modern vehicular design. Engineers focus on developing lighter, more efficient waveguide designs to integrate seamlessly with autonomous vehicle architecture. Further research into meta-materials could also pave the way for novel waveguide applications in future autonomous vehicle systems.
waveguides - Key takeaways
- Waveguides: Structures that guide waves such as electromagnetic or sound with minimal energy loss, crucial for signal transmission.
- Waveguide Propagation: Involves confining and directing wave energy efficiently through reflection and transmission within the waveguide's structure.
- Waveguide Modes: Determines how electromagnetic fields distribute in the waveguide, including Transverse Electric (TE) and Transverse Magnetic (TM) modes.
- Optical Waveguide: Guides light signals in telecommunication systems, operating on the principle of total internal reflection to minimize loss.
- Rectangular Waveguide: A common type used in microwave applications with a rectangular cross-section, allowing multiple modes of propagation.
- CPW Coplanar Waveguide: Used in electronics, offering low radiation loss and simplified integration with other components, calculated with effective dielectric constant and substrate thickness.
Learn faster with the 10 flashcards about waveguides
Sign up for free to gain access to all our flashcards.
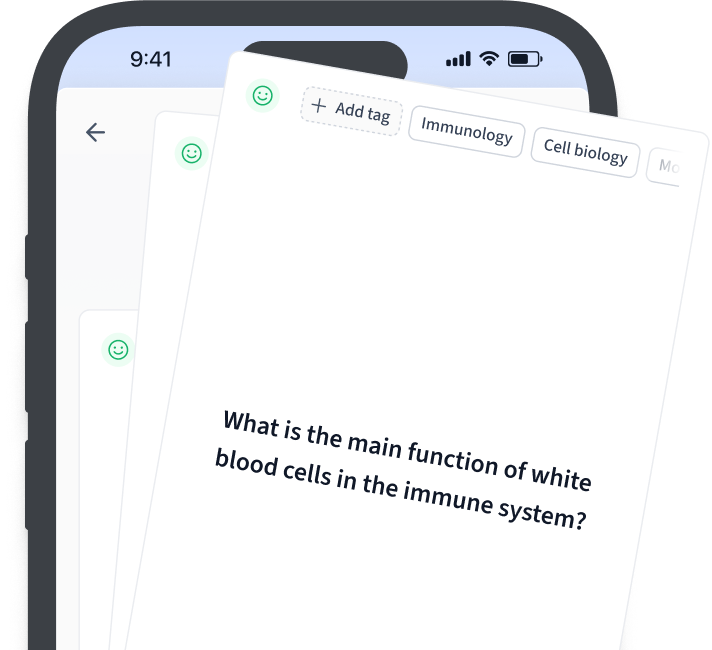
Frequently Asked Questions about waveguides
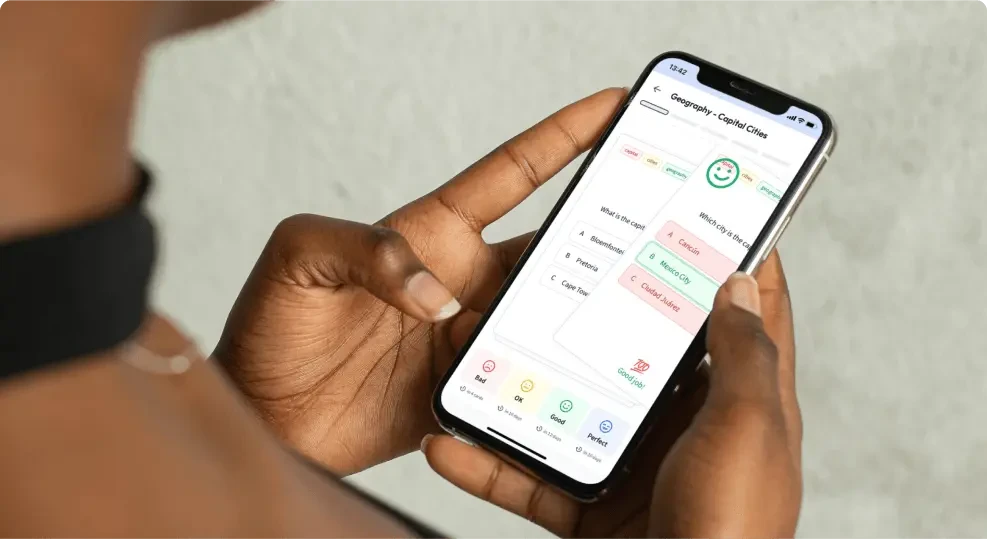
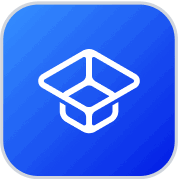
About StudySmarter
StudySmarter is a globally recognized educational technology company, offering a holistic learning platform designed for students of all ages and educational levels. Our platform provides learning support for a wide range of subjects, including STEM, Social Sciences, and Languages and also helps students to successfully master various tests and exams worldwide, such as GCSE, A Level, SAT, ACT, Abitur, and more. We offer an extensive library of learning materials, including interactive flashcards, comprehensive textbook solutions, and detailed explanations. The cutting-edge technology and tools we provide help students create their own learning materials. StudySmarter’s content is not only expert-verified but also regularly updated to ensure accuracy and relevance.
Learn more