Jump to a key chapter
Introduction to Biomechanics in Robotics
The field of biomechanics in robotics bridges the gap between natural biological systems and artificial mechanical systems. By studying biological movements, engineers apply these principles to enhance robotic functions, particularly in areas requiring intricate motion and responsiveness.
What is Biomechanics in Robotics?
Biomechanics in robotics involves analyzing the mechanics of biological systems and transferring these insights to robotic designs. This discipline provides critical insights for developing robots that can mimic, emulate, or improve upon human and animal movements. By considering factors such as muscle contractions, joint movements, and load distribution, engineers can create robots with enhanced agility and flexibility.
In biomechanics, robots are often developed to perform tasks such as walking, grasping, and even mimicking complex human actions like typing or playing musical instruments. The premise behind this approach is that biological systems have evolved over millions of years to move efficiently and effectively. By capturing these systems' fundamentals, robots can be designed to achieve similar, if not superior, efficiencies.
Key areas influenced by biomechanics include:
- Prosthetics: Mimicking limb movements to create functional prosthetic devices
- Exoskeletons: Enhancing human capabilities by supplementing their movement
- Rehabilitation robotics: Assisting in recovery and therapy through precise and adaptive motion
- Soft robotics: Creating flexible and adaptable robots that can navigate dynamic environments
Biomechanics: The study of the structure, function, and motion of the mechanical aspects of biological systems, using the principles of mechanics.
A robot using biomechanics principles might use actuators as artificial muscles, allowing it to simulate the flexibility and resilience of these muscles in its movement patterns.
Consider biomechanics in the context of athletic performance, where understanding forces and motion can optimize training and equipment.
When exploring biomechanics in robotics further, you'll find that this area requires an interdisciplinary approach, combining knowledge from fields such as biology, mechanical engineering, and computer science. Advanced techniques like computational modeling and kinematic analysis are often used to simulate and refine robotic movements. For example, computational modeling can be used to predict how a robot's joint will react to a certain load, allowing engineers to design joints that closely mimick the natural movements of living creatures. Moreover, machine learning algorithms can optimize the robot's movements by analyzing repeated actions and refining the robot's pathway for more natural motion responses.
Importance of Biomechanics in Automation
Biomechanics plays a significant role in automation by providing robots with the ability to perform tasks in a human-like manner. This is crucial in fields such as manufacturing, where precise yet flexible movements are required. These principles enable robots to handle delicate tasks that were once thought only achievable by human hands.
A fundamental aspect of this is the ability to develop actuators and sensors that replicate the feedback mechanisms seen in nature. This can create machines that not only move efficiently but also make real-time adjustments to their environment. For example, in manufacturing lines, a robot arm might use force sensors to adjust its grip based on the size and shape of an object, much like how human fingers work.
- Enhanced Precision: Use of biomechanics leads to increased precision in tasks such as assembly, welding, or painting.
- Adaptability: Robots can adapt dynamically to changes in their environment, a crucial trait for unpredictable tasks.
- Energy Efficiency: Biomimetic designs often lead to more energy-efficient robots, conserving power and reducing operational costs.
Consider a surgical robot that utilizes biomechanics. It is designed to perform incisions with accuracy similar to a human hand, maintaining movements within micrometer precision to ensure patient safety and procedure efficiency.
Incorporating biomechanics can significantly transform industries like agriculture, where robots with dexterous movements can help with delicate tasks such as picking fruits without causing damage.
The future of biomechanics in automation may include further integration with artificial intelligence (AI) to enhance decision-making processes. Imagine robots that can independently learn from their environment by analyzing biomechanical data, adjusting their actions toward more complex, less structured environments. Recent developments in neural networks and AI have shown promising results in this area, potentially paving the way for robots that adapt over time, improving efficiency, performance, and the ability to handle unexpected circumstances. Furthermore, the integration of tactile feedback systems akin to human touch in robots may emerge, providing robots with a ‘sense of touch’ that could revolutionize tasks in both medical fields and manufacturing environments.
Applications of Biomechanics in Robotics
Understanding the applications of biomechanics in robotics helps in developing machines that can replicate or enhance human capabilities. This understanding is crucial for designing robots that can perform complex tasks with precision, efficiency, and adaptability, which are often seen in biological organisms.
Engineering Biomechanics for Enhanced Robotic Design
Incorporating biomechanics into robotic design emphasizes the importance of mimicking biological structures. To achieve this, engineers often analyze the mobility and load distribution in various organisms. By examining these aspects, they can create robotic systems capable of performing tasks in diverse environments with similar ease and adaptability found in nature. This approach proves beneficial in areas such as prosthetics and biomimetic robotics, where the goal is to simulate natural movements as closely as possible.
A critical component of this design is utilizing actuators that function like biological muscles. These actuators need to provide a broad range of motion and power, essential for replicating human-like movement. Accordingly, robotic joint designs are often influenced by human joints, facilitating seamless motion and energy efficiency akin to their biological counterparts.
To optimize these designs, biomechanics also incorporates mathematical modeling. Key equations include:
- Equations of motion: \[F = ma\], where F is the force applied, m is the mass, and a is the acceleration of the robotic component.
- Energy conservation: \[KE + PE = constant\], with KE representing kinetic energy and PE potential energy, illustrating how energy is distributed within the robotic system.
Biomimetic Robotics: A field in robotics where designs are inspired by biological entities, focusing on replicating the mechanics and dynamics of living organisms to enhance robotic function.
An example of engineering biomechanics in robotic design is the creation of a bionic arm that uses actuators to mimic the muscle contractions in a human arm, allowing the wearer to perform a wide range of activities with high dexterity.
It's interesting to note how advancements in sensor technology contribute significantly to enhancing the functionalities of biomechanical robots.
In-depth exploration into biomechanics in robotics often involves applying computational fluid dynamics (CFD) to assess how robots can move through fluids such as air and water efficiently. This is particularly relevant for underwater and aerial robots, which must account for fluid resistance when moving. Furthermore, integrating artificial intelligence (AI) allows robots to optimize their movements in real-time based on the biomechanical feedback they receive. This can lead to further innovations such as teaching a robot to walk over different terrains by using feedback loops to adjust gaits for steep, slippery, or rocky surfaces, similar to how humans and animals adjust their steps.
Techniques in Biomechanics for Robotics Applications
Diverse techniques in biomechanics are utilized to enhance robotic applications. One of the primary methods is kinematic analysis, which involves studying the geometry of motion without considering the forces causing it. This allows engineers to forecast the movement patterns of robotics parts and design components that can adapt to various tasks efficiently.
Another essential technique is the use of mechanical joint optimization. By understanding joint behavior, robots can be designed to replicate not only the motion but also durability and responsiveness similar to biological joints. This results in devices that require less maintenance and can perform consistently under strenuous conditions. Additionally, techniques such as finite element analysis (FEA) are employed to predict how robots will react under different workloads and stress environments, ensuring structural integrity before physical prototypes are manufactured.
Commonly applied equations in biomechanics come from Newtonian mechanics and thermodynamics to understand how these forces interact within a robotic system.
- Torque Calculation: \[\tau = r \times F\], where \tau is torque, r is the distance from the pivot point, and F is the force applied.
- Heat Engine Principle: \[\eta = \frac{W}{Q_h}\], displaying efficiency (\eta) as a ratio of work done (W) to the heat absorbed (Q_h).
A biomechanical technique application can be seen in designing robotic exoskeletons used in rehabilitation. These devices require precise kinematic analysis to ensure they assist the patient’s natural gait without imposing additional strain.
Keep an eye on how biometrics and biomechanics are blending, paving the way for more personalized robotic solutions.
Biomechanics in Robotic Design
Integrating biomechanics into robotic design revolutionizes how robots interact with their environment. By emulating the efficient and complex movements seen in biological organisms, robotic systems achieve higher levels of functionality and adaptability.
Incorporating Biomechanics in Robotic Movement
Incorporating biomechanics into robotic movement involves the precise study and application of biological motion patterns to artificial systems. This integration is crucial for creating robots capable of performing dynamic tasks, such as walking on uneven terrain or manipulating delicate objects. Robotics engineers use sophisticated models and simulations to replicate muscle dynamics, which in turn improve the machine's agility and efficiency.
One foundational principle here is mimicking human joint articulation through robotic joints that provide similar range and control of motion. By analyzing kinematics, engineers can devise motion trajectories that optimize speed and minimize energy consumption. This involves using equations like:
- Newton’s Second Law: \[F = ma\], where F is the net force, m is mass, and a is acceleration.
- Rotational Dynamics: \[I \alpha = \tau\], with I as the moment of inertia, \alpha as angular acceleration, and \tau as torque.
Kinematics: The branch of physics or mechanics that involves the analysis of movement without considering the forces that affect that movement.
An example of biomechanics in robotic movement is the Boston Dynamics robot, which uses dynamic balance and sophisticated joint mechanics to navigate complex environments like staircases, similar to a human.
Understanding muscle-tendon mechanics is crucial because it provides insights into designing actuators that replicate muscular force and elasticity.
Advanced robotics utilize neural networks for sensorimotor control, mimicking the human nervous system to process sensory inputs and execute precise movements. By embedding artificial intelligence within biomechanically inspired frameworks, robots can adapt rapidly to unexpected changes in their environment. For instance, a robot can learn to recalibrate its steps when encountering a new obstacle. Moreover, interdisciplinary approaches merging biomechanics with machine learning foster robots that can improve their task execution over time by learning from their experiences. This dynamic adaptation stems from algorithms analyzing feedback loops, similar to how the human brain processes errors to refine motor skills.
Innovations in Biomechanics for Robotic Design
Recent innovations in biomechanics for robotic design focus on creating more adaptable and responsive robots. These advancements are largely driven by discoveries in the material sciences that allow for the development of more flexible and durable components, closely resembling natural biological tissues.
Materials like smart polymers and shape-memory alloys are widely integrated into modern robotic systems, contributing to systems that can change shape or stiffness dynamically. In applications, one popular method involves tuning a robot's stiffness in response to the forces it encounters, employing principles derived from human muscle structures.
Roboticists also implement biologically-inspired swarm intelligence, drawing parallels to ant and bee colonies, where simple local interactions yield complex global behaviors. This has proven particularly powerful in robotics where decentralized control systems allow for more robust and flexible operations, leading to innovations such as:
- Adaptive locomotion systems
- Self-healing materials
- Energetically autonomous robots, equipped with systems for energy harvesting from environmental sources
An innovative approach is seen in soft robotics, where materials and designs based on octopus tentacles provide highly adaptive movement capabilities that are difficult to achieve with traditional rigid robotics.
Consider how using evolutionary algorithms in biomechanical design can lead to novel and optimized robotic systems through iterative testing and adaptation.
Future Trends in Biomechanics in Robotics
The field of biomechanics in robotics is rapidly evolving, influenced by innovations in technology and the growing understanding of biological systems. This section will discuss future trends, including emerging technologies and the challenges and opportunities faced by integrating biomechanics in robotic design. These advancements aim to push the boundaries of what is achievable with robotic systems, providing enhanced efficiency and versatility.
Emerging Technologies in Biomechanics in Robotics
Emerging technologies in biomechanics present cutting-edge opportunities for developing robots that are more agile, autonomous, and effective in mimicking human and animal movements. Some key technologies include:
- Soft Robotics: Utilizing materials that imitate the flexibility and adaptability of natural musculature to navigate complex terrains.
- Tactile Sensors: Enhancements in sensors resembling a human sense of touch enable robots to perform delicate operations with precision.
- AI and Machine Learning: Implementing these algorithms to advance the cognitive capabilities of robots, allowing them to learn and adapt to their environments more independently.
These technologies are essential for developing robots capable of performing not just routine assembly tasks but also complicated tasks requiring subtlety and precision. The mathematical modeling that informs these technologies is deeply rooted in the principles of biomechanics. For example:
- Calculating force in soft robotics: \[F = \text{{Integral}} \frac{{E \times \text{{strain}}}}{{\text{{Area}}}} \], where E is the modulus of elasticity.
- Sensors calibration formulas implementing thermal law as \[Q = mCT \], where Q is the heat added, m is mass, C is specific heat, and T is temperature.
Consider a robotic gripper enhanced by tactile sensors and soft material technology, capable of adjusting its grip based on the object's shape and delicacy, much like a human hand handling a ripe fruit.
Exploring further, the convergence of biomechanics and biotechnology may lead to breakthroughs in cyborg robotics. These robots would augment human capabilities beyond natural physical limits, through interfaces embedded directly within the human body. Developments in advanced nanotechnology might enable real-time data transmission between biological and mechanical systems, opening doors for enhanced sensory feedback and faster cognitive responses by integrating neural prosthetics. These advancements are at the crossroads of AI, biotechnology, and biomechanics, stimulating research and development of next-generation robotics that seamlessly blend with human users.
Biomechanics in Robotics: Future Challenges and Opportunities
Biomechanics in robotics holds immense potential, but there are hurdles to overcome to fully realize its benefits. Engineers face numerous challenges, such as:
- Developing cost-effective and high-performance materials suitable for long-term use.
- Ensuring safety and compliance with ethical standards, particularly for humanoid robots that bridge closely with human interaction.
- Tackling the compute and power demands of sophisticated AI algorithms necessary for autonomous decision-making.
Opportunities abound when these challenges are met, including the potential for robots to revolutionize industries like healthcare, agriculture, and logistics. Robots enabled by biomechanical advancements could undertake more diverse tasks with augmented accuracy and reliability.
Mathematical challenges include solving complex nonlinear equations to model robotic movement accurately. For example:
- Inverse Dynamics: \[\tau = M(q)q'' + C(q,q')q' + g(q)\], where τ is torque, q is the joint coordinate vector, M represents the mass matrix, C is the Coriolis and centrifugal terms, and g is the gravitational forces vector.
Keep an eye on regulatory frameworks that play a crucial role in shaping the ethical implications and guidelines for robotic research and deployment.
The intersection of biomechanics with other technological advancements, such as quantum computing, could redefine computational approaches in optimizing robotic design and operation. Quantum algorithms, by solving problems exponentially faster than classical algorithms, could potentially facilitate real-time adjustments in robotic behaviors under complex and changing environmental conditions. This capacity, though in the realm of theoretical exploration currently, signals a transformative trajectory for robotics, making them smarter and more efficient in ever-evolving ecosystems.
biomechanics in robotics - Key takeaways
- Biomechanics in robotics bridges biological systems and mechanical systems, enhancing robotic functions through principles of biological movement.
- Engineering biomechanics involves analyzing biological mechanics for robotic design, improving agility and flexibility by mimicking human and animal movements.
- Key applications include prosthetics, exoskeletons, rehabilitation robotics, and soft robotics for dynamic environments.
- Advanced techniques like kinematic analysis and computational modeling help refine robotic movements by simulating biological joint mechanics.
- Biomechanics enhances automation, allowing robots to handle tasks with human-like precision and adaptability in manufacturing.
- Incorporating biomechanics into robotic design improves functionality and adaptability, using intelligent materials and AI integration for efficient motion.
Learn faster with the 12 flashcards about biomechanics in robotics
Sign up for free to gain access to all our flashcards.
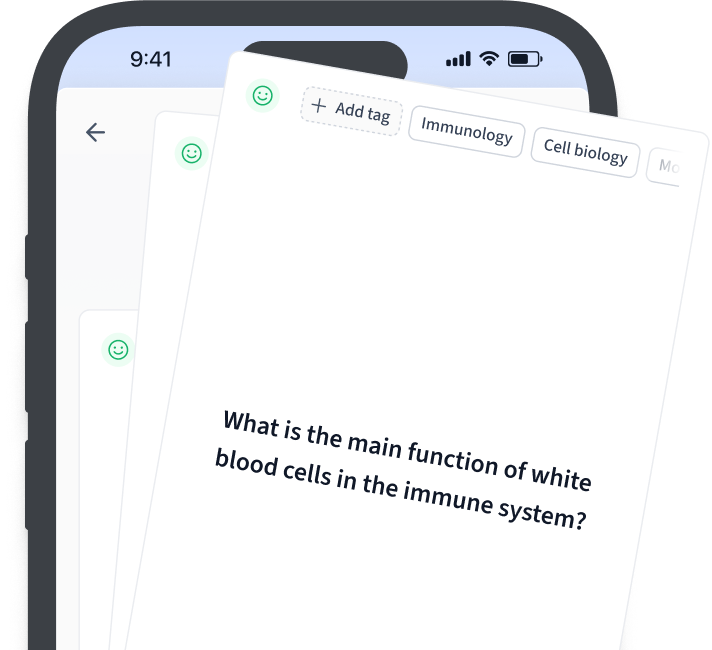
Frequently Asked Questions about biomechanics in robotics
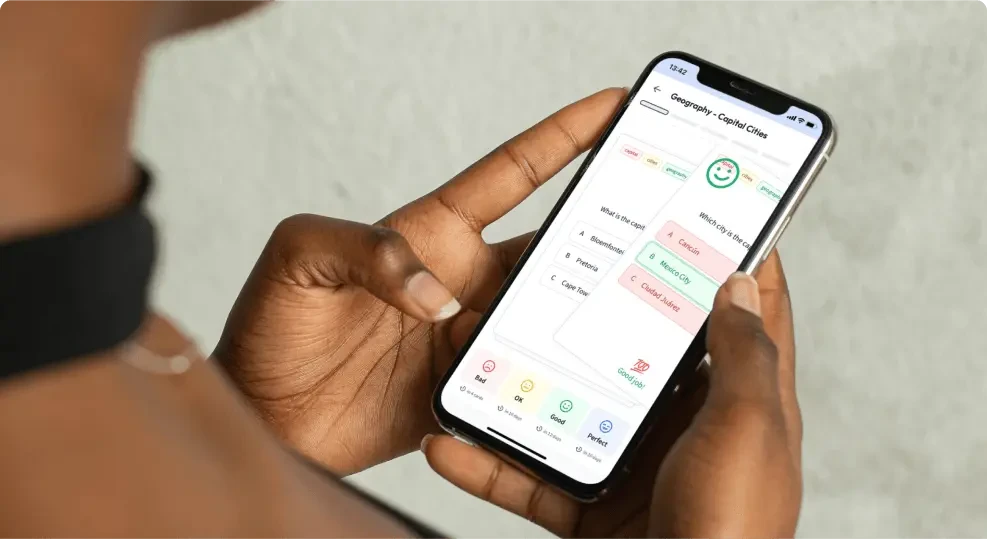
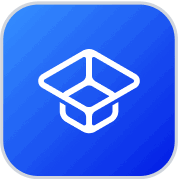
About StudySmarter
StudySmarter is a globally recognized educational technology company, offering a holistic learning platform designed for students of all ages and educational levels. Our platform provides learning support for a wide range of subjects, including STEM, Social Sciences, and Languages and also helps students to successfully master various tests and exams worldwide, such as GCSE, A Level, SAT, ACT, Abitur, and more. We offer an extensive library of learning materials, including interactive flashcards, comprehensive textbook solutions, and detailed explanations. The cutting-edge technology and tools we provide help students create their own learning materials. StudySmarter’s content is not only expert-verified but also regularly updated to ensure accuracy and relevance.
Learn more