Jump to a key chapter
Definition of Myoelectric Control is based on the utilization of electrical signals produced by muscles during contraction. These signals can be harnessed to control devices such as prosthetics, providing users with a way to regain functions.
Basics of Myoelectric Control
Myoelectric control is a fascinating technology that bridges biology and electronics. This technology essentially uses the electrical potential generated by muscles when they contract to control devices. These signals, known as myoelectric signals, are measured on the skin's surface over the muscles.
The process can be broken down into several key steps:
- Detecting muscle signals using electrodes
- Processing and amplifying these signals for clarity
- Decoding the signals to understand intended actions
- Linking the decoded signals to a mechanical output
One of the primary uses of myoelectric control is in prosthetic limbs. By placing electrodes on specific muscles, signals generated by contracting muscles can replace missing limb functions.
Myoelectric signals represent the electrical activity produced by muscles. These signals are crucial in translating intention into practical actions.
Consider a prosthetic hand operated through myoelectric control. By flexing the forearm muscle, a corresponding signal is sent to the prosthetic, causing it to close in a gripping motion.
Myoelectric Signals and Their Role
Myoelectric signals are pivotal in connecting human intention with mechanical execution. These signals are essentially the body's natural electrical activity emanating from muscles, typically in the range of 0 to 20 mV. To make use of these signals, it is crucial to understand their nature and the factors affecting them.
Key Factors influencing myoelectric signals:
- Muscle mass and size which affect signal strength
- Electrode placement and contact quality
- Electromagnetic interference from external sources
When leveraging myoelectric signals in control systems, it is important to transform them into a readable and actionable format. This often involves:
- Amplification: Enhancing the weak myoelectric signals to a usable level
- Filtering: Removing noise and irrelevant information
- Pattern Recognition: Analyzing the signals to decipher the user's intended movement
In prosthetic applications, algorithms interpret these signals to execute precise mechanical movements. The mathematical models used might involve linear and non-linear computations. For instance, the relationship between signal amplitude and muscle force can be represented as:
\[F = k \times \text{Signal Amplitude}\]Where \(F\) is the force and \(k\) is a constant determined through calibration.
Electrode positioning can dramatically affect signal quality in myoelectric systems.
Myoelectric Control is a pivotal subject in the engineering domain, leveraging the innate capability of muscle-generated signals to control external devices. By understanding the underlying principles, one can appreciate how technology interprets our body's language into mechanical actions.
Electrical Signals in Myoelectric Control Systems
Electrical signals generated by muscles form the backbone of myoelectric control systems. These signals, measured in millivolts, provide insights into muscular function and intention. The human body naturally produces these signals during muscle contractions, which can then be detected using surface electrodes.
Here's how these signals play a role in myoelectric control:
- When you decide to move a limb, muscles contract, generating electrical signals.
- These signals are captured non-invasively using electrodes placed on the skin.
- The captured signals undergo processing to amplify and filter out noise.
An understanding of the electrical properties involved is essential. For example, electrode impedance can impact signal clarity and must be minimized for effective signal capture. Additionally, factors like skin condition and placement sensitivity play a crucial role in the quality of the myoelectric signal.
Mathematical Representation:
Signal strength can be expressed as \(V = IR\) where:
- \(V\) is the voltage (myoelectric signal amplitude)
- \(I\) is the current produced by muscle activity
- \(R\) is the resistance which might vary depending on skin conditions
Analyzing the spectral content of myoelectric signals provides insights into the muscle's health and fatigue levels. Techniques such as Fourier Transform are used in this analysis. The frequency range of interest is typically between 20-500 Hz.
Myoelectric Control Techniques
Myoelectric control techniques involve sophisticated methods to interpret muscle signals. These techniques enable the seamless operation of prosthetic devices and other systems by translating myoelectric signals into actionable commands.
Some popular myoelectric control techniques include:
- Pattern Recognition: This technique employs machine learning algorithms to analyze signal patterns and predict intended movements.
- Threshold-based Control: Signals are monitored, and actions are triggered when they exceed a certain threshold.
- Contraction Phase Analysis: Distinguishing between signals during different contraction phases allows for more refined control.
Each technique has its advantages and challenges. For example, pattern recognition requires extensive training data but offers high accuracy by identifying complex signal patterns.
Example:
Consider a scenario where a person with an upper limb prosthesis uses pattern recognition to operate their artificial arm. Sensors on their residual limb capture the muscle signals, which are then processed to recognize whether the intention is to grasp, rotate, or release an object.
Myoelectric Control Applications in Engineering have revolutionized numerous fields by offering sophisticated methods of translating muscular intentions into precise mechanical actions. These applications range from prosthetics to advanced robotics, illustrating the diverse potential of myoelectric technology.
Innovations and Advancements
The field of myoelectric control is continuously evolving with remarkable innovations. These advancements significantly enhance the efficiency and capability of systems utilizing myoelectric control, making them more adaptable to users' needs.
Some exciting innovations include:
- Multi-channel Electrode Arrays: These capture a broader range of signals, improving the resolution and responsiveness of myoelectric devices.
- Machine Learning Integration: By implementing machine learning, signal interpretation becomes more accurate, reducing error rates in control systems.
- Wireless Communication: Eliminating wires increases user comfort and device functionality, providing more freedom of movement.
A key development in myoelectric control technology is hybrid systems that combine myoelectric signals with other biosignals, enhancing the flexibility and adaptability of devices. For example, combining electromyography with electroencephalography can enable more comprehensive control over prosthetic limbs.
Example:
An innovative prosthetic hand uses machine learning algorithms to interpret myoelectric signals. With pattern recognition, the system identifies specific hand gestures, enabling the user to perform complex tasks with greater precision.
The use of graphene-based electrodes can improve signal quality and durability in myoelectric systems.
Myoelectric control systems sometimes incorporate real-time feedback loops. These systems enable immediate adjustments by analyzing signal deviations, thus increasing the accuracy of the devices. Feedback can be visual, auditory, or tactile, providing the user with a comprehensive interaction with the device.
Case Studies in Myoelectric Control
Case studies provide valuable insights into the effectiveness and practical application of myoelectric control. By examining real-world scenarios, you can understand the challenges and triumphs associated with implementing these systems.
Case Study 1: Prosthetic Limb Development
An engineering team developed a myoelectric prosthetic arm for a teenage amputee. Through extensive training and calibration, the prosthetic replicated various hand positions. The project highlighted the critical role of signal calibration and machine learning in aligning device responses with user intentions.
Case Study 2: Rehabilitation Robotics
In a rehabilitation center, therapists used myoelectric control in robotic exoskeletons to enhance patient recovery. By mapping body movements through muscle signals, the technology provided patients with an intuitive control mechanism, boosting recovery rates.
Both case studies emphasize the significance of personalization in myoelectric applications. Each user's biological signals are unique, and tailoring devices to these individual profiles is key for success. Thus, engineers are continually striving to optimize myoelectric systems for broader applicability and user satisfaction.
Proportional Myoelectric Control offers a more nuanced approach compared to binary systems by allowing a proportional response based on the intensity of myoelectric signals. This control method significantly enhances the capability and precision of devices, especially in prosthetics.
Understanding Proportional Myoelectric Control
Proportional myoelectric control systems allow devices to move in a manner proportional to the strength of the muscle signals generated. This can be highly beneficial in applications where the precise control of movement is necessary.
The main components of a proportional myoelectric control system include:
- Signal Detection: Using electrodes to capture muscle-generated electrical signals.
- Signal Amplification: Enhancing signal strength to make it readable by the control system.
- Control Algorithms: Employing mathematical models to translate signal strength into proportional movement. An example formula used might be:
\[F_{\text{output}} = k \times A_{\text{signal}}\]
where \( F_{\text{output}} \) is the force or speed exerted by the device, \( k \) is a constant, and \( A_{\text{signal}} \) is the amplitude of the myoelectric signal.
This control system provides smoother transitions and more natural movements compared to traditional on-off control systems. It requires precise calibration to align the hardware response with user expectations.
A prosthetic arm equipped with proportional myoelectric control can adjust its grip strength based on the intensity of the user's muscle contractions, allowing delicate actions like holding a fragile object or exerting greater force for heavier tasks.
Calibration is crucial for ensuring the proportional response aligns accurately with the user's intentions.
The mathematical modeling of proportional myoelectric control can involve advanced techniques such as machine learning for real-time adaptation. Algorithms continuously learn from user inputs, adjusting the constant \(k\) to refine responsiveness and improve the system over time.
Benefits in Robotics Engineering
Proportional myoelectric control is transforming robotics engineering by offering precise and scalable control options. Such advancements bridge the gap between human intentions and mechanical execution, leading to more efficient and responsive systems.
Benefits in robotics include:
- Enhanced Precision: Devices can perform tasks with varying intensities, improving dexterity and control.
- Increased Efficiency: Robots can adapt their force application based on the requirements of different tasks, optimizing energy use.
- Natural Interaction: The proportional control provides a more intuitive interface for users, mimicking natural human movements.
For instance, in assembly line robots, proportional control allows the application of the right amount of pressure for assembling delicate parts, minimizing damage and errors. This adaptability is crucial in robotics fields such as manufacturing, healthcare, and even service robots.
myoelectric control - Key takeaways
- Myoelectric Control: Utilizes electrical signals produced by muscles during contraction to control devices like prosthetics.
- Myoelectric Signals: Electrical activity from muscles measured on the skin, critical for translating intentions into actions.
- Myoelectric Control System: Involves detecting, processing, decoding muscle signals, and linking them to mechanical output.
- Myoelectric Control Techniques: Include pattern recognition, threshold-based control, and contraction phase analysis for interpreting muscle signals.
- Proportional Myoelectric Control: Responds proportionally to signal intensity, enhancing precision in applications like prosthetics and robotics.
- Myoelectric Control Applications in Engineering: Revolutionizes fields by translating muscular intentions into precise mechanical actions, such as in robotics and prosthetics.
Learn faster with the 12 flashcards about myoelectric control
Sign up for free to gain access to all our flashcards.
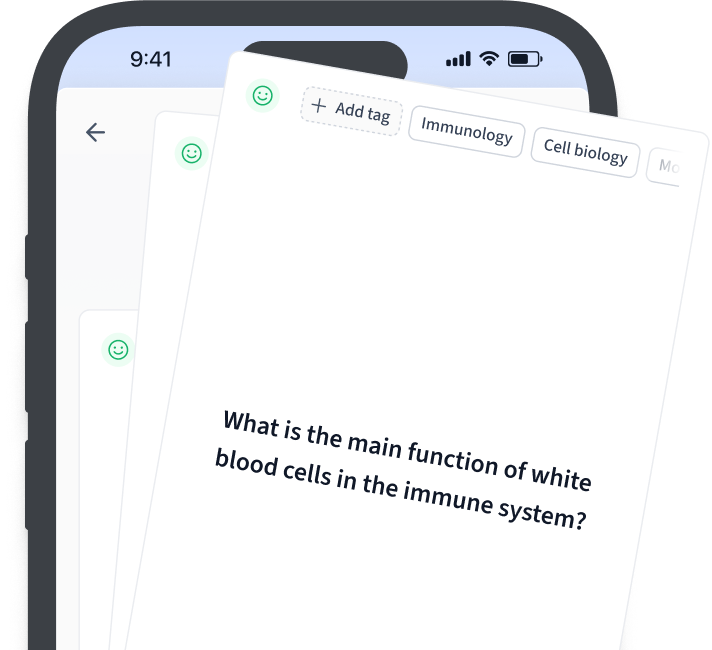
Frequently Asked Questions about myoelectric control
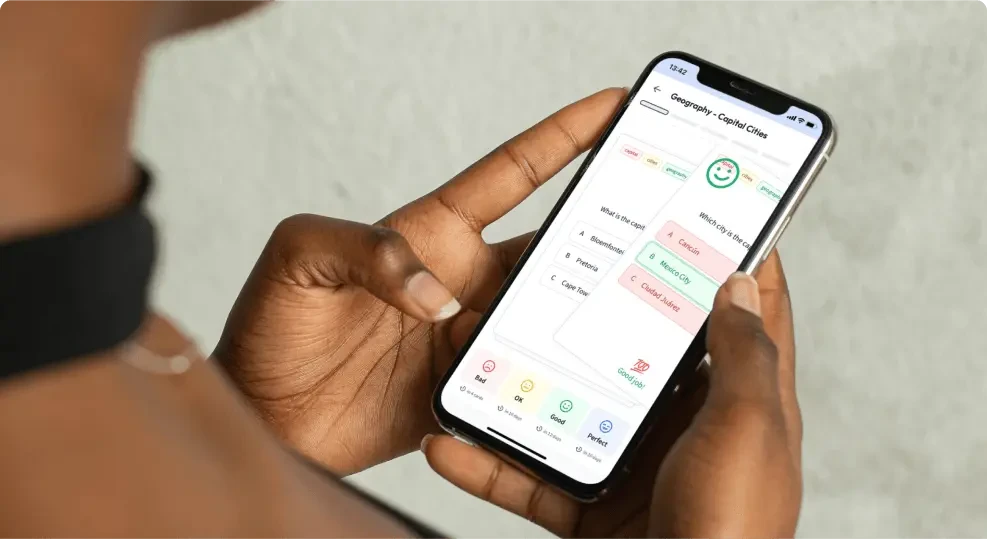
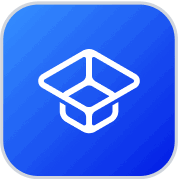
About StudySmarter
StudySmarter is a globally recognized educational technology company, offering a holistic learning platform designed for students of all ages and educational levels. Our platform provides learning support for a wide range of subjects, including STEM, Social Sciences, and Languages and also helps students to successfully master various tests and exams worldwide, such as GCSE, A Level, SAT, ACT, Abitur, and more. We offer an extensive library of learning materials, including interactive flashcards, comprehensive textbook solutions, and detailed explanations. The cutting-edge technology and tools we provide help students create their own learning materials. StudySmarter’s content is not only expert-verified but also regularly updated to ensure accuracy and relevance.
Learn more