Jump to a key chapter
Prosthetics Design Overview
The design of prosthetic limbs is an intricate field that merges engineering, healthcare, and technology. Prosthetics are artificial devices that replace missing limbs and provide crucial functionality to individuals. Understanding the basics of prosthetics design can unlock the door to innovative solutions in mobility and rehabilitation.Advancements in this field not only improve the life quality of users but also push the boundaries of engineering creativity. From material selection to biomechanics, each component demands careful consideration.
Materials Used in Prosthetics Design
When designing prosthetics, the choice of materials is paramount. The materials must be lightweight yet strong, durable yet flexible. Common materials used include:
- Titanium: Known for its strength and corrosion resistance.
- Carbon fiber: Offers a high strength-to-weight ratio.
- Plastic polymers: Used for their flexibility and lightness.
For instance, a carbon fiber prosthetic leg may weigh only a few kilograms yet support dynamic movements like running and jumping. This is due to the anatomical mimicry crucial in creating functional replacements.
Biomechanics plays a pivotal role in prosthetics design. Engineers study how the human body moves to replicate these movements in artificial limbs. They often use concepts like:
- Joint mechanics: Ensuring accurate hinge points for natural movement.
- Load distribution: Calculating the force and stress on each component.
- Kinematics: Analyzing time and space involved in limb movement.
Technological Integration in Prosthetics
Modern prosthetics incorporate technology to enhance functionality. Microprocessors and sensors are embedded to adjust the prosthetic's response to different terrains and movements. Technologies such as:
- Pressure sensors: Measure the load and adjust electrical signals accordingly.
- Actuators: Provide motorized assistance to mimic muscle function.
- Feedback systems: Allow users to sense the environment through vibrations or electrical signals.
Prosthetics can be custom-tailored to fit an individual, ensuring comfort and efficiency, often with the help of 3D scanning and printing technology.
Engineering Principles in Prosthetics
The engineering principles embedded in prosthetics design focus on creating functional, reliable, and efficient artificial limbs. These principles are shaped by understanding the complexities of human motion and translating them into mechanical operations. By comprehensively applying biomechanics, materials science, and sensor technology, prosthetics designers can innovate solutions that significantly improve user mobility and comfort.
Biomechanics and Prosthetics
Biomechanics is the study of the mechanics of living organisms, particularly the forces exerted by muscles and gravity on the skeletal structure. In prosthetics, applying biomechanics means replicating the natural movements of a human limb.Key concepts in biomechanics include:
- Joint torque: Engineers need to calculate the rotational force needed for limb movement, using the formula \( \tau = I \cdot \alpha \), where \( \tau \) is torque, \( I \) is the moment of inertia, and \( \alpha \) is angular acceleration.
- Load-bearing capacity: Ensures that the prosthetic can support the user's weight during activities like walking or running.
Consider a user walking on uneven ground with a prosthetic leg. The leg's knee joint must adapt to different angles and distribute weight evenly. Calculating the forces involved requires an understanding of kinematics, represented by the formula \( v = u + at \), where \( v \) is the final velocity, \( u \) is the initial velocity, and \( a \) is acceleration over time \( t \).
Material Selection in Prosthetics
The choice of materials in prosthetics is crucial for performance and user comfort. Engineers must select materials that are lightweight yet offer strong tensile strength. Common materials include titanium and carbon fiber, each with its unique advantages.Material characteristics:
- Titanium: Known for its biocompatibility and strength, it is ideal for weight-bearing components.
- Carbon fiber: Combines light weight with strong tensile properties, appropriate for dynamic movements.
Carbon fiber prosthetics can be custom-designed for athletes to minimize weight while maximizing speed and flexibility.
In advanced prosthetics, material science plays a vital role. For instance, shape memory alloys (SMAs) have memory properties that allow them to return to an original shape when heated. This can be articulated through the equation for transformation temperature: \( T = T_0 + \frac{\beta}{n} \), where \( T_0 \) is the reference temperature, \( \beta \) is a constant, and \( n \) is a phase transformation indicator. These materials allow engineers to incorporate automatic contraction and relaxation in prosthetics, providing a more natural limb function.
Sensor Technology in Prosthetics
Integrating sensor technology in prosthetics enhances the functional capabilities. Sensors collect and transmit data to adapt prosthetic behavior to different activities. The primary sensor technologies used include:
- Pressure sensors adjust the limb's movement dynamically based on the force applied by the user.
- Myoelectric sensors detect electrical potential generated by muscle fibers, allowing for device control based on user intent.
Biomechanics of Prosthetic Design
The field of prosthetic design is deeply intertwined with biomechanics, the science of movement mechanics in living organisms. Understanding the biomechanics of human motion helps engineers create prosthetic limbs that function seamlessly with the user's body. This integration ensures that prosthetics not only restore mobility but also prevent discomfort and injury by mimicking natural limb movements. By analyzing kinematics, dynamics, and joint mechanics, engineers can design prostheses that provide both functionality and comfort.
Biomechanics refers to the study of the structure, function, and motion of the mechanical aspects of biological systems. In prosthetic design, biomechanics involves creating artificial limbs that replicate natural human movements.
Kinematics in Prosthetic Design
Kinematics, a branch of classical mechanics, deals with the geometry of motion without considering the forces that cause it. In prosthetic design, kinematics is used to study and replicate the range of motion in limbs. By understanding the kinematic chains of human limbs, prosthetic engineers can accurately design joints and segments. These aspects ensure that the prosthesis moves in the same way an organic limb does.Important components of kinematics include:
- Angular displacement: The change in angle as a body rotates about an axis.
- Velocity and acceleration: Critical for timing the prosthetic's response to motion.
Designing a prosthetic knee joint requires an understanding of angular displacement: \[ \theta = \theta_0 + \omega \cdot t + \frac{1}{2}\alpha \cdot t^2 \] where \( \theta \) is the final angle, \( \theta_0 \) is the initial angle, \( \omega \) is the angular velocity, \( \alpha \) is angular acceleration, and \( t \) represents time.
A deeper dive into kinematics involves analyzing the entire gait cycle of walking. The modular approach segments the movement into:
- Stance phase: Begins when the foot contacts the ground, providing stability and support.
- Swing phase: Involves the leg moving through the air preparing for the next step.
Dynamics and Joint Mechanics
While kinematics focuses on movement geometry, dynamics incorporates the forces and torques that produce motion. In prosthetics, understanding dynamics ensures that prosthetic joints can handle loads and produce realistic movements.The fundamental aspects of dynamics in prosthetic design include:
- Mass and inertia: Affect how a limb responds to movement and change in motion.
- Torque and force: Influence how energy is used to initiate and sustain motion. Calculated using the formula \[ \tau = I \cdot \alpha \] where \( \tau \) is torque, \( I \) is the moment of inertia, and \( \alpha \) is angular acceleration.
An example of dynamic analysis is in designing an ankle prosthesis. The torque required to lift a foot is determined by factors such as limb weight and expected acceleration: \[ F = m \cdot a \] where \( F \) is the force, \( m \) is mass, and \( a \) is acceleration.
Prosthetic limbs often incorporate hydraulic or pneumatic systems to better manage energy and enhance control during dynamic movements.
Design of Prosthetic Limbs
The design of prosthetic limbs involves merging engineering with medical sciences to restore functionality and aid mobility for those with limb loss. Engineers must balance biomechanics, materials science, and sensor technology in their designs to ensure an optimal fit and function.The process involves analyzing natural limb movements to create artificial counterparts that mimic these actions. This requires a deep understanding of both the engineering principles and the user's needs.
Prosthetic Leg Designs
Prosthetic leg designs are tailored to assist individuals in regaining mobility. These designs must consider joint mechanics, weight distribution, and dynamic response to various activities—from walking to running.Key components in prosthetic leg design include:
- Knee joint mechanisms: Such as rotational hinges or microprocessor-controlled joints for smoother motion.
- Foot design: Flexible or spring-loaded feet that react to user weight and stride.
A microprocessor-controlled prosthetic knee uses sensors to detect movement and automatically adjusts the limb for a more natural gait.
For example, a microprocessor-controlled knee joint in a prosthetic leg adapts to the user's speed and terrain, offering stability. The force exerted by the user can be calculated as \( F = m \cdot a \), where \( F \) is force, \( m \) is mass, and \( a \) is acceleration.
A deeper look into leg prosthetics reveals advancements like:
- Hydraulic systems: Allow for controlled resistance and smoother movements.
- Dynamic socket fittings: Custom-fitted for comfort and to mitigate skin irritation.
Prosthetic Arm Design
Designing a prosthetic arm involves understanding the complex range of motion of the human arm and translating this into artificial limb mechanics. These prostheses aim to restore dexterity and strength for everyday tasks.Critical elements include:
- Elbow joints: Can be mechanical or motorized, simulating bending and extending motions.
- Shoulder attachments: Provide rotational movement and support to the arm.
Consider an electric motor used in a prosthetic elbow. The torque necessary for lifting an object is influenced by formula \( \tau = r \times F \), where \( \tau \) is torque, \( r \) is the position vector, and \( F \) is the force vector.
Modern prosthetic arms can connect to smartphones, allowing users to fine-tune settings for specific activities.
Myoelectric technology in arm prosthetics allows for control using the electrical signals generated by the user's own muscles. Sensors embedded in the prosthetic detect these signals and convert them into movements. This technology enables a high degree of customization and control, enhancing usability. For example, the commands for different hand grips are encoded based on the intensity and duration of muscle signals detected.This level of intricate design showcases the synergy between human anatomy and engineering, paving the way for prosthetics that closely mimic natural limb function.
Prosthetic Hand Design
Prosthetic hand designs focus on replicating the complex movements and tactile feedback of a natural hand. This includes fine motor skills such as gripping and manipulation.Design considerations involve:
- Finger articulation: Multi-jointed fingers for dexterity.
- Sensor integration: Provides haptic feedback, allowing users to 'feel' pressure and texture.
A myoelectric prosthetic hand uses electrical signals from the user's muscles to control hand movements, mimicking natural hand functions.
A myoelectric hand may open and close by detecting changes in muscle activity levels. The force of the grip can be adjusted using the equation \( F \approx \frac{V}{R} \), where \( F \) is the force, \( V \) is the voltage from muscle signals, and \( R \) is resistance in the circuit.
prosthetics design - Key takeaways
- Prosthetics Design: The field of prosthetics design integrates engineering, healthcare, and technology to create artificial devices that replace missing limbs, enhancing mobility and rehabilitation.
- Biomechanics of Prosthetic Design: Biomechanics plays a pivotal role, focusing on replicating human movements in prosthetic limbs through joint mechanics, load distribution, and kinematics.
- Engineering Principles in Prosthetics: These principles involve understanding human motion and translating it into mechanical operations using biomechanics, materials science, and sensor technology.
- Design of Prosthetic Limbs: Balancing biomechanics, materials, and technology to mimic natural limb movements, prosthetic limbs are designed for optimal fit and function.
- Prosthetic Leg Designs: Focus on joint mechanics, weight distribution, and dynamic responses, often incorporating technologies like microprocessor-controlled knee joints.
- Prosthetic Arm and Hand Design: These designs aim to restore dexterity and involve complex mechanics and biosensors, with hands emphasizing articulation and haptic feedback for realistic function.
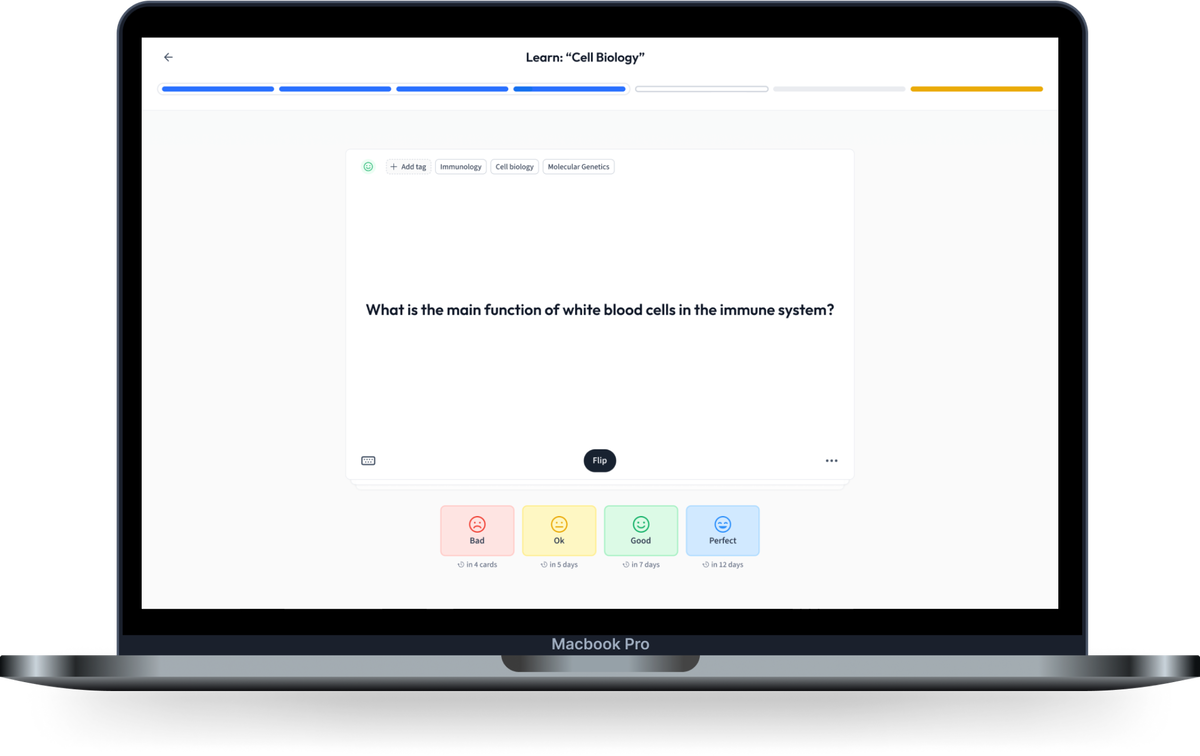
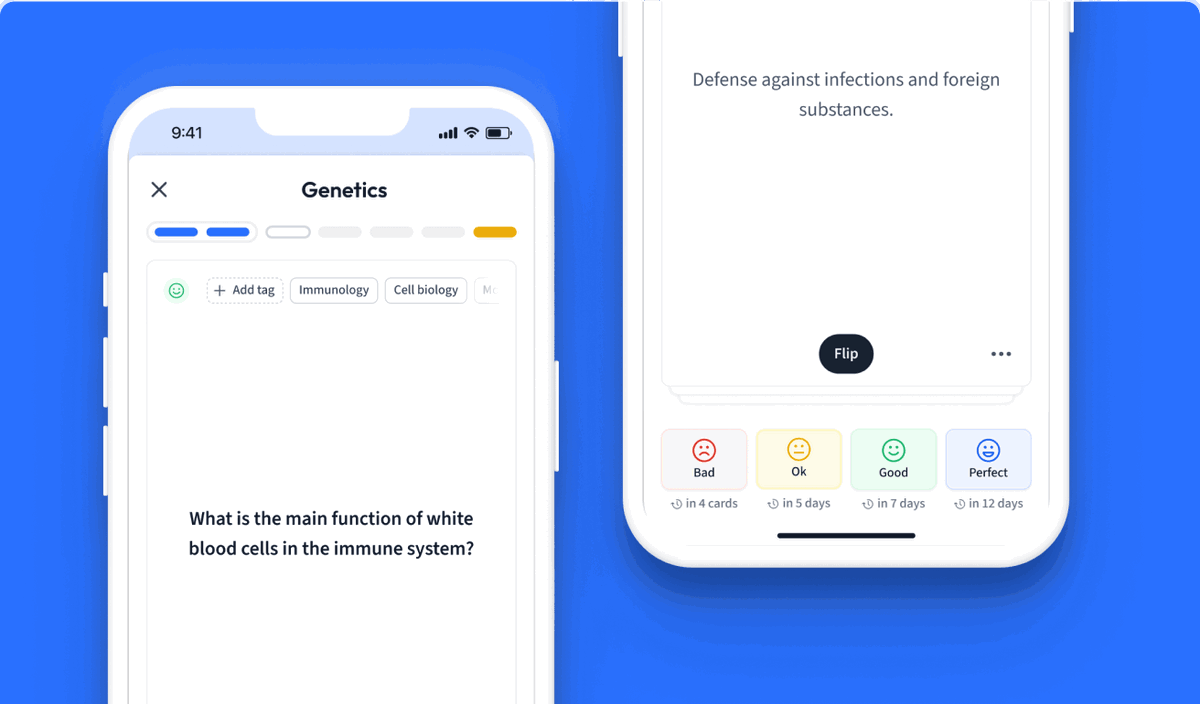
Learn with 12 prosthetics design flashcards in the free StudySmarter app
Already have an account? Log in
Frequently Asked Questions about prosthetics design
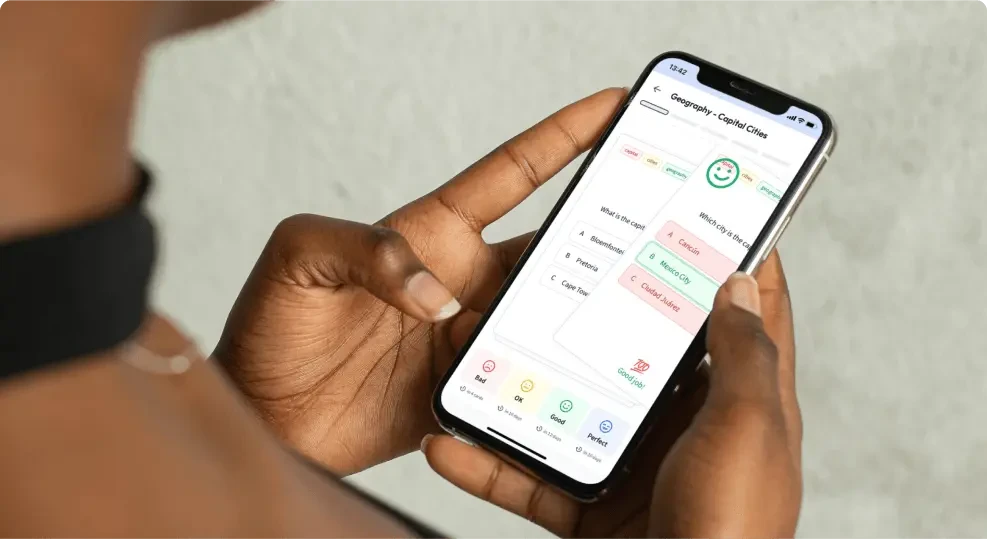
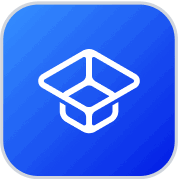
About StudySmarter
StudySmarter is a globally recognized educational technology company, offering a holistic learning platform designed for students of all ages and educational levels. Our platform provides learning support for a wide range of subjects, including STEM, Social Sciences, and Languages and also helps students to successfully master various tests and exams worldwide, such as GCSE, A Level, SAT, ACT, Abitur, and more. We offer an extensive library of learning materials, including interactive flashcards, comprehensive textbook solutions, and detailed explanations. The cutting-edge technology and tools we provide help students create their own learning materials. StudySmarter’s content is not only expert-verified but also regularly updated to ensure accuracy and relevance.
Learn more