Jump to a key chapter
Genetic Improvement Meaning and Implications
Understanding genetic improvement involves exploring the modifications of an organism's genetic material to enhance its traits. This concept is crucial in both agricultural advancements and ecological conservation efforts. Genetic improvement can lead to more resilient crops or the preservation of endangered species.
Genetic Improvement Definition
Genetic Improvement: A process by which the genetic composition of organisms is altered to achieve desirable traits, such as increased yield in plants or improved disease resistance.
Genetic improvement involves several techniques, including traditional breeding, genetic engineering, and gene editing. These methods are employed in multiple sectors, demonstrating the extensive applications and benefits within ecosystems and agriculture.
It's important to understand how genetic improvement differs from genetic modification, though they often overlap in practice.
Historical Context of Genetic Improvement
The history of genetic improvement dates back thousands of years. Early farmers began selectively breeding animals and plants to produce specific traits, unknowingly laying the foundation for modern genetic techniques. These ancient practices eventually evolved into systematic methods utilized today.By the 20th century, advancements in genetics began to revolutionize agriculture. The discovery of DNA and subsequent understanding of genetic code permitted precise manipulations of genes. This led to increased food production and enhanced quality of crops and livestock.
In the mid-20th century, the 'Green Revolution' marked a significant breakthrough in genetic improvement. The introduction of high-yielding variety (HYV) seeds notably increased agricultural output in many developing countries. Scientists like Norman Borlaug played pivotal roles in these advancements, contributing to hunger prevention worldwide.
Genetic Improvement Examples in Ecosystems
In natural ecosystems, genetic improvement helps ensure the stability and diversity of life forms. For instance, conservation geneticists focus on genetic restoration to prevent species extinction. Another example is the selective breeding of plants and animals which contributes to ecosystem sustainability.
A practical example is the genetic improvement of coral species to withstand changing ocean conditions. By selecting corals with heat-resistant traits, scientists aim to support coral reef survival under climate change duress.
Marine ecosystems often leverage genetic improvement techniques to bolster resilience against environmental stressors.
Genetic Improvement Techniques
Exploring genetic improvement techniques reveals two primary categories: traditional and modern methods. Each approach offers unique strategies and benefits for enhancing organism traits, with diverse applications in agriculture, medicine, and environmental science.The significance of genetic improvement has grown substantially, providing vegan dishes, climate-resilient crops, and sustainable ecosystem management.
Traditional Techniques in Genetic Improvement
Traditional genetic improvement techniques have roots in early agriculture where methods such as selective breeding were commonplace. These techniques rely on inherent genetic variations observed in nature to enhance desirable traits.Key traditional techniques include:
- Selective Breeding: Mating chosen animals or plants with desired characteristics to produce offspring with those traits.
- Hybridization: Crossing different species or strains to combine advantageous traits.
- Mutagenesis: Inducing mutations using chemicals or radiation to achieve beneficial changes.
Technique | Description |
---|---|
Selective Breeding | Deliberate mating of organisms to enhance specific traits. |
Hybridization | Crossing different species for advantageous traits. |
Mutagenesis | Use of chemicals/radiation to induce beneficial mutations. |
For example, hybridization was applied in creating 'Broccolini,' a hybrid between broccoli and Chinese kale, capitalizing on desirable traits of both vegetables.
Modern Techniques in Genetic Improvement
Modern genetic improvement techniques harness advanced scientific knowledge to enact precise genetic changes. These advancements allow for highly targeted modifications, often unattainable through traditional methods.Notable modern techniques include:
- Genetic Engineering: Direct manipulation of an organism's DNA.
- CRISPR-Cas9: A cutting-edge tool for gene editing that offers precision in altering genetic information.
- Genomic Selection: Using genomic information to predict and select desirable traits.
Technique | Description |
---|---|
Genetic Engineering | Direct alteration of an organism's genetic material. |
CRISPR-Cas9 | Precise gene editing tool for altering DNA sequences. |
Genomic Selection | Employing genomic data to select advantageous traits. |
CRISPR-Cas9 technology revolutionizes genetic improvement by allowing scientists to edit genes with unprecedented accuracy. The system works by locating a specific DNA sequence and cutting it, enabling targeted gene insertion, deletion, or replacement. This technique holds significant potential in correcting genetic defects and combating diseases.
Ethical Considerations in Genetic Improvement
The practice of genetic improvement inevitably raises ethical questions, concerning the balance of benefits against potential risks and moral dilemmas.Key ethical considerations include:
- Biodiversity: Potential loss if genetic uniformity is pursued.
- Ecosystem Impact: Unintended consequences on natural systems.
- Food Security: Accessibility and affordability of genetically modified organisms (GMOs) for all populations.
- Consent: Whether adequate informed consent is obtained for human genetic interventions.
Consideration | Description |
---|---|
Biodiversity | Concern over reduced genetic diversity. |
Ecosystem Impact | Possible adverse effects on ecosystems. |
Food Security | Equitability in GMO distribution. |
Consent | Importance of informed consent for genetic changes in humans. |
Engaging in genetic improvement demands a careful consideration of ethical frameworks to ensure sustainable and just applications.
Impact of Genetic Improvement on Environmental Sustainability
The impact of genetic improvement on environmental sustainability is profound, with significant implications for biodiversity, climate change mitigation, and socio-economic benefits. By understanding these connections, you can better appreciate the potential of genetic technologies in promoting a healthier planet.
Genetic Improvement and Biodiversity
Genetic improvement plays a vital role in preserving and enhancing biodiversity. By improving traits like disease resistance and climate adaptability in plants and animals, these technologies can bolster the resilience of ecosystems.
- Enhancement of genetic diversity within species can prevent population bottlenecks and extinctions.
- Conservation efforts benefit from genetic improvement through the preservation of endangered species.
- Introduction of beneficial traits can secure ecosystem health and function.
Selective breeding of crops like drought-resistant maize ensures their survival in varying climatic conditions, supporting biodiversity by maintaining crop diversity.
The concept of 'rewilding' uses genetic improvement to restore ecosystems to their natural states. This involves reintroducing species that play critical roles in ecosystem dynamics, sometimes utilizing improved genetic strains to enhance their survival.
Genetic Improvement in Climate Change Mitigation
The role of genetic improvement in climate change mitigation is increasingly recognized. By adapting crops and trees to changing environmental conditions, genetic technologies help sequester carbon and sustain agricultural yields.
- Development of crops that require fewer inputs (such as water or fertilizers) reduces environmental footprints.
- Genetically improved trees with enhanced growth or carbon capture capacities offer climate change solutions.
- Resilient crops ensure food security despite climate variability.
Carbon Sequestration: The process of capturing and storing atmospheric carbon dioxide to mitigate climate change.
Genetic improvements in crops can reduce reliance on chemical pesticides, further decreasing environmental impact.
Socio-Economic Benefits of Genetic Improvement
Socio-economic benefits from genetic improvement manifest through increased agricultural productivity, reduced waste, and improved quality of life. Through efficient resource use and enhanced crop traits, communities can experience economic growth and stability.
- Better crop yields translate to higher farmer incomes and improved food security.
- Improved crop resilience lowers risk and increases economic stability in agricultural sectors.
- Resources such as land, water, and labor are utilized more efficiently.
In regions like Sub-Saharan Africa, genetically improved crops contribute to increased food production and income generation, aiding in poverty reduction efforts.
Aside from economic advantages, genetic improvement can have profound social impacts by improving nutritional content of crops, thereby enhancing public health. Golden Rice, engineered to contain vitamin A, serves as a pivotal example in reducing nutritional deficiencies among vulnerable populations.
Applications for Genetic Improvement in Agriculture and Forestry
The use of genetic improvement techniques in agriculture and forestry is essential for addressing food security and environmental challenges. This section delves into how genetic strategies can advance crop yields, adapt to environmental changes, and provide real-world examples in agriculture.
Crop Yield Enhancement Through Genetic Improvement
To increase agricultural productivity, genetic improvement techniques focus on enhancing crop yield through various methods. These include:
- Genetic Engineering: Direct alteration of genes to promote growth and resistance.
- Crossbreeding: Combining different varieties to improve yield and quality.
- Marker-Assisted Selection: Using molecular markers to select desirable traits efficiently.
Enhanced crop varieties often require less water and pesticides, contributing to resource conservation.
A notable example of yield enhancement is the development of genetically engineered rice varieties that are resistant to diseases like bacterial blight, resulting in significantly improved harvests.
In-depth studies have shown that genetic improvements, such as the deployment of 'super crops,' can lead to a 20%-30% increase in yields. These genetically superior crops are engineered to thrive in various environmental conditions and are less susceptible to pests, offering promising solutions to global food shortages.
Genetic Adaptation Strategies in Environmental Science
Genetic adaptation strategies are pivotal in mitigating the impacts of climate change and environmental degradation. Through adaptation, species can become more resilient to stressors such as:
- Temperature Variability: Developing heat-tolerant crop species.
- Disease Pressure: Breeding disease-resistant plants and trees.
- Water Scarcity: Introducing drought-resistant varieties to conserve water.
Genetic strategies can be tailored to target specific environmental challenges in different regions.
Case Studies of Genetic Improvement in Agriculture
Several case studies illustrate successful applications of genetic improvement in agriculture. These studies highlight the diverse benefits of genetic strategies:
- Drought-Resistant Maize: In Africa, genetically improved maize varieties have been introduced to withstand arid conditions, boosting productivity.
- Golden Rice Project: In Asia, rice enhanced with vitamin A addresses nutritional deficiencies, benefiting public health.
- Pest-Resistant Cotton: In the United States, genetically engineered cotton exhibits reduced pesticide needs, leading to sustainable farming practices.
The success of drought-resistant maize in African regions is a testament to genetic improvement capabilities. These maize varieties maintain yields even under water stress, supporting food security for millions.
Golden Rice, fortified with beta-carotene, represents a groundbreaking biofortification effort to combat vitamin A deficiency. This initiative showcases the potential of genetic improvement in addressing global nutritional challenges while maintaining agricultural productivity.
genetic improvement - Key takeaways
- Genetic Improvement Meaning: Refers to alterations in an organism's genetic makeup to enhance specific traits like yield or disease resistance.
- Genetic Improvement Techniques: Encompasses methods like traditional breeding, genetic engineering, and CRISPR-Cas9.
- Impact on Environmental Sustainability: Assists in climate change mitigation, biodiversity preservation, and minimal input agriculture.
- Genetic Adaptation Strategies: Involves creating heat-tolerant or drought-resistant species to adapt to environmental changes.
- Applications in Agriculture: Enhances crop yields and resilience with applications like drought-resistant maize and pest-resistant cotton.
- Examples in Ecosystems: Includes selective breeding in coral species for climate adaptability and biodiversity conservation.
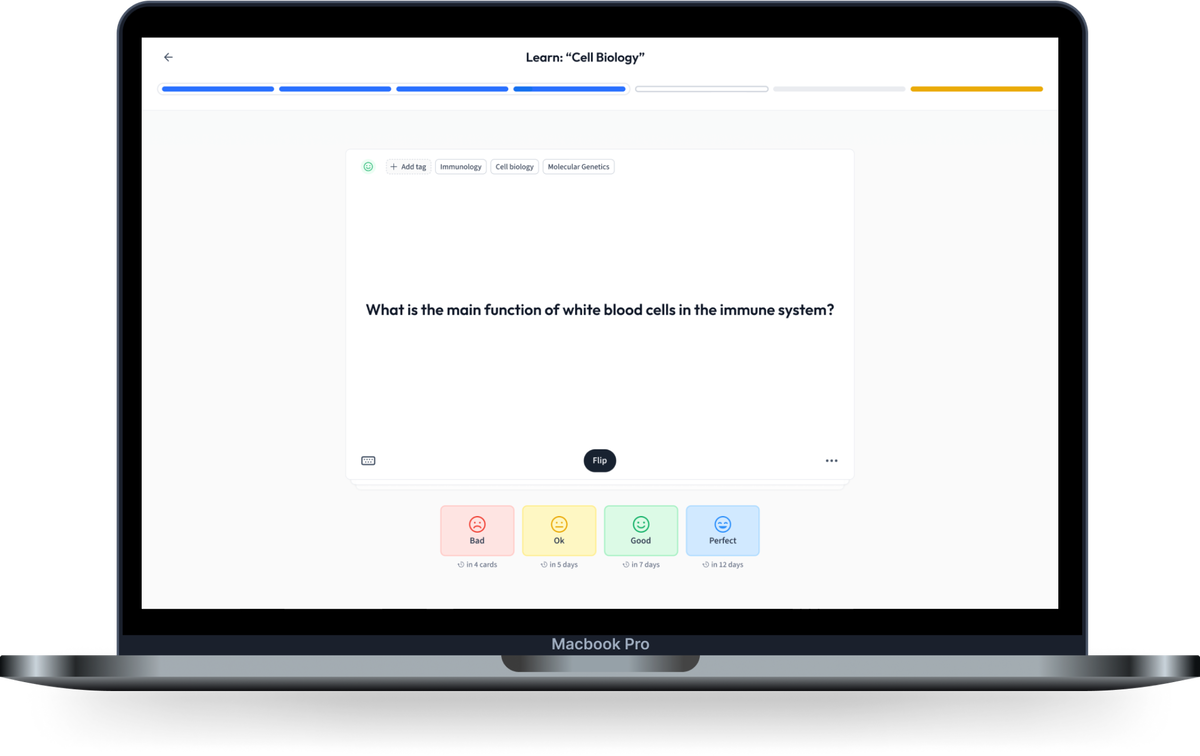
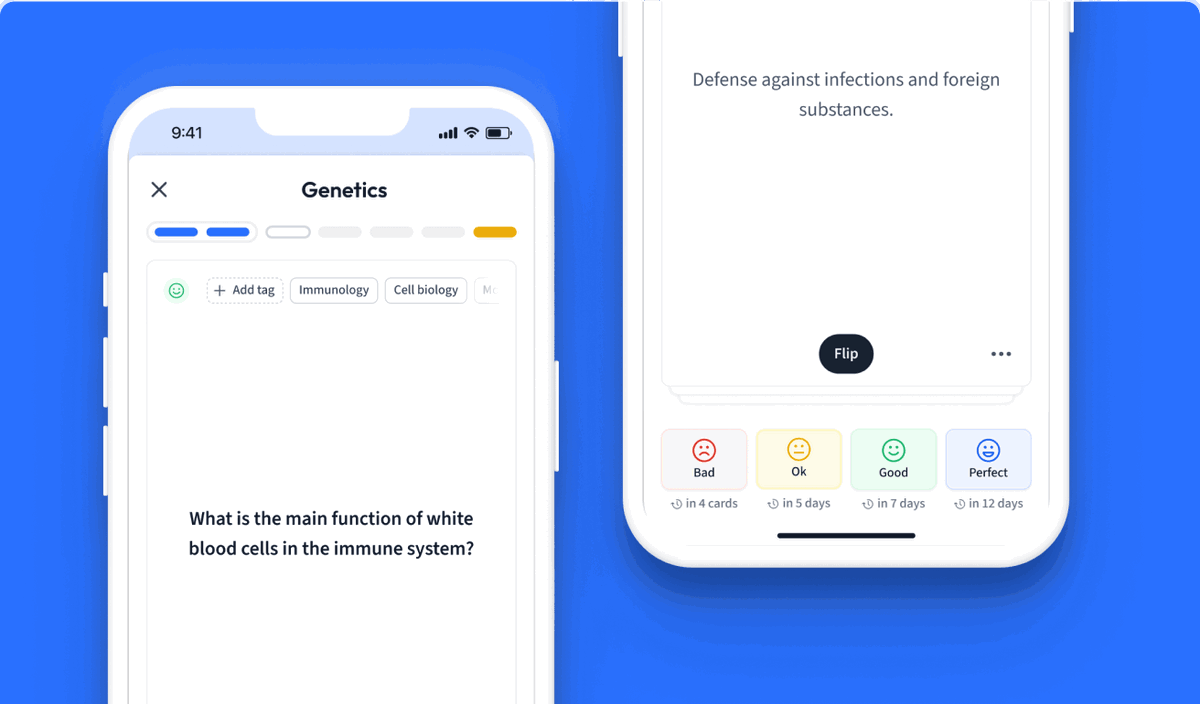
Learn with 24 genetic improvement flashcards in the free StudySmarter app
Already have an account? Log in
Frequently Asked Questions about genetic improvement
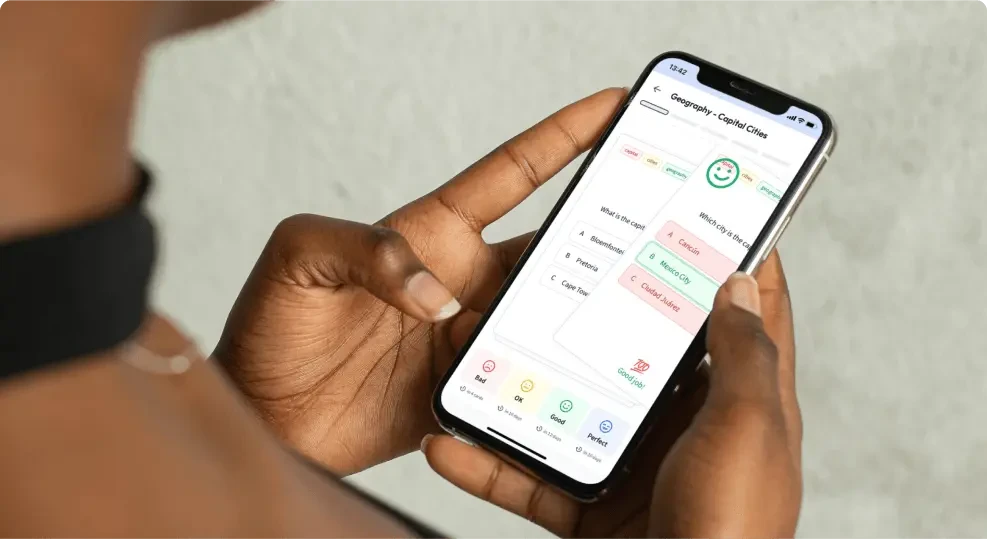
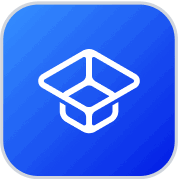
About StudySmarter
StudySmarter is a globally recognized educational technology company, offering a holistic learning platform designed for students of all ages and educational levels. Our platform provides learning support for a wide range of subjects, including STEM, Social Sciences, and Languages and also helps students to successfully master various tests and exams worldwide, such as GCSE, A Level, SAT, ACT, Abitur, and more. We offer an extensive library of learning materials, including interactive flashcards, comprehensive textbook solutions, and detailed explanations. The cutting-edge technology and tools we provide help students create their own learning materials. StudySmarter’s content is not only expert-verified but also regularly updated to ensure accuracy and relevance.
Learn more