Jump to a key chapter
Dynamics of Sea Ice Overview
The dynamics of sea ice play a crucial role in the Earth's climate system. Understanding how sea ice forms, deforms, and melts helps us grasp its impact on global climate patterns. Let's dive deeper into these dynamics and their implications.
Formation and Growth of Sea Ice
Sea ice formation begins when ocean water freezes. This process is influenced by temperature, salinity, and ocean currents. Initially, small ice crystals, known as frazil ice, form in the water. As they accumulate, they create a slushy layer. Eventually, these ice crystals solidify into a continuous ice cover called nilas.
An example of sea ice growth can be seen in the Arctic, where the temperature falls below freezing, leading to a rapid accumulation of ice. The thickness of the ice can range from a few centimeters to several meters.
Sea ice can be defined as frozen ocean water, which floats on the sea surface. It's pivotal in regulating heat exchange between the ocean and the atmosphere.
As sea ice thickens, it particularly relies on thermodynamic processes and mechanical forces. Thermodynamically, ice thickens from the bottom as heat is lost to the colder atmosphere above. This is governed by the formula: \[ G = k(T_s - T_b)/H \]where \(G\) represents the growth rate, \(k\) is thermal conductivity, \(T_s\) is surface temperature, \(T_b\) is the bottom temperature, and \(H\) is ice thickness.
Deformation and Movement of Sea Ice
Deformation occurs when sea ice is subjected to winds, currents, and temperature changes. This leads to processes like rafting and ridging. Rafting happens when thinner ice slabs slide over one another, while ridging involves the formation of thicker, jagged ice features.
The study of sea ice motion is complex due to the myriad forces at play. The motion is affected by the Coriolis effect, which causes a deflection of currents and wind. This resultant movement can be modeled through the equation: \[ F = ma = \rho A C_d (v - u)^{1/2} \]where \(F\) is the force exerted by the wind on the ice, \(a\) is acceleration, \(m\) is mass, \(\rho\) is air density, \(A\) is the area, \(C_d\) is the drag coefficient, and \(v-u\) is the relative velocity of the wind to the ice.
Melting and Decline of Sea Ice
Sea ice melting is influenced by several factors including temperature rise, salinity changes, and solar radiation. The absorption of heat by ice, expressed in the equation: \[Q = mc\Delta T\]where \(Q\) is the heat absorbed, \(m\) is mass, \(c\) is specific heat capacity, and \(\Delta T\) is the temperature change, leads to melting.
For instance, during the summer months, increased solar radiation heats the surface, leading to a significant reduction in Arctic sea ice extent.
Melting sea ice is distinct from melting icebergs or glaciers in its contribution to sea level rise, as sea ice is already floating and displaces its mass in water. However, it can alter ocean salinity and temperature.
Moreover, as ice melts, it contributes to the albedo effect, where less sunlight is reflected back into space. This creates a feedback loop, where increased absorption of sunlight by the ocean accelerates further ice melt, influencing climate patterns on a larger scale. Understanding these dynamics helps predict future climate changes and their global impacts.
Factors Influencing Sea Ice Dynamics
The factors influencing the dynamics of sea ice involve a range of environmental and physical components that interact to affect its formation, movement, and melting. These factors are critical for understanding sea ice behavior and its broader impact on global climate systems.
Temperature
Temperature is one of the most significant factors affecting sea ice dynamics. Colder temperatures promote ice formation and growth, while warmer temperatures contribute to melting. The influence of temperature is evident through the variation in ice thickness and extent across different seasons and regions.
The Earth's polar regions act as temperature regulators, affecting atmospheric conditions worldwide. Hence, changes in sea ice dynamics can amplify global climatic alterations.
Temperature variation affects sea ice through a process known as thermodynamics. It involves energy transfer between the atmosphere and the ocean, influencing ice formation and melt rate.
A detailed examination of thermodynamic processes shows the energy balance equation critical for understanding sea ice dynamics: \[ Q = \rho c_v (T_s - T_i) + L \] where \(Q\) represents heat flux, \(\rho\) is density, \(c_v\) is specific heat capacity, \(T_s\) is surface temperature, \(T_i\) is ice temperature, and \(L\) is latent heat of fusion.
Ocean Currents
Ocean currents play a crucial role in driving the movement of sea ice. Currents distribute heat and impact ice thickness by gusting warm water to the ice edges, enhancing melting, or colder water, aiding growth.
Growing evidence suggests that variations in the Atlantic Meridional Overturning Circulation (AMOC) significantly affect the patterns and dynamics of Arctic sea ice, causing shifts in ice extent.
Ocean currents interact with sea ice through forces such as friction and pressure, leading to deformation. The dynamics can be modeled using equations like: \[ \tau = \rho_w u_w^2 C_d \]where \(\tau\) is the shear stress, \(\rho_w\) is water density, \(u_w\) is water velocity, and \(C_d\) is the drag coefficient.
Wind Patterns
Wind is an external force that heavily influences sea ice dynamics, primarily in terms of movement and deformation. Strong winds can drive the ice across the ocean surface, forming pressure ridges and open leads.
During polar vortex events, high wind speeds can significantly alter sea ice distribution.
A well-known concept is the Ekman transport, which describes how wind influences ocean surface layers, leading to significant ice drift across the polar regions.
The interaction between wind and sea ice can be captured by the force balance equation: \[ F = \rho_a C_d A (u_a - u_i) \]where \(F\) is the wind force, \(\rho_a\) is air density, \(C_d\) is the drag coefficient, \(A\) is the area, and \(u_a\) and \(u_i\) are the velocities of air and ice, respectively.
Biology of Sea Ice and Its Ecosystem
The unique environment of sea ice supports diverse biological communities that are adapted to extreme conditions. These ecosystems contribute significantly to the Earth's biodiversity and influence global oceanic food webs. Understanding the biology within sea ice is crucial for comprehending its ecological roles.
Primary Producers: Algae and Photosynthesis
Sea ice hosts algae that conduct photosynthesis, serving as primary producers. These algae grow on the undersurface of the ice, thriving in nutrient-rich brine channels. Their photosynthetic process captures solar energy, producing oxygen and organic matter essential for other organisms in the ecosystem.
Sea ice algae can be defined as microscopic plants living within the sea ice matrix, playing a vital role in carbon fixation and as a food source for higher trophic levels.
An example of sea ice primary production is seen in the Arctic, where the presence of algae leads to blooms that support zooplankton populations during the spring.
The productivity of sea ice algae is quantified by measuring chlorophyll-a concentration, using the light attenuation coefficient in the formula: \[ P = P_{max} (1 - e^{-C_{chl-a}I}) \]where \(P\) is the photosynthesis rate, \(P_{max}\) is the maximum rate of photosynthesis, \(C_{chl-a}\) is chlorophyll-a concentration, and \(I\) is the irradiance.
Zooplankton and Nutrient Cycling
Zooplankton are a key part of the sea ice ecosystem, feeding on algae and other microorganisms. They play a crucial role in nutrient cycling by breaking down organic matter, recycling nutrients back into the ecosystem. This ensures continued productivity and sustenance for higher trophic levels like fish and birds.The migration patterns of zooplankton, such as \textit{copepods}, provide an important link in the food web, moving nutrients vertically through the water column.
The unique brine channels within sea ice create microhabitats that support these and many other microscopic organisms.
Predators in the Sea Ice Ecosystem
The sea ice ecosystem supports a variety of predators, including fish, seabirds, and marine mammals. These predators rely on the extensive food chains sustained by ice algae and zooplankton.
For example, polar bears depend on seals as a primary prey source, using the sea ice as a platform for hunting.
In this intricate food web, changes in sea ice dynamics can influence predator-prey relationships, impacting the survival and distribution of species.
Studies have shown that the predation success of species like the ringed seal (\textit{Pusa hispida}) is closely linked to ice thickness, as it affects the accessibility to breathing holes maintained in the ice. The population dynamics of predators can be modeled with Lotka-Volterra equations, reflecting these intricate interactions.
Impact of Climate Change on Sea Ice
Climate change significantly affects the dynamics of sea ice, leading to alterations in its extent, thickness, and movement patterns. These changes have ripple effects on global climate systems and marine ecosystems. Understanding these impacts is crucial for comprehending climate trends.
Arctic Sea Ice Characteristics
Arctic sea ice is vital to Earth's climate regulation, reflecting sunlight due to its high albedo. As global temperatures rise, the characteristics of Arctic sea ice are transforming, leading to various environmental consequences.Over recent decades, data indicates a noticeable decline in Arctic sea ice extent, particularly during summer months. This reduction affects heat exchange processes between the ocean and atmosphere, influencing weather patterns globally.
The Arctic is experiencing more rapid warming compared to other regions, a phenomenon known as 'Arctic amplification'.
Arctic sea ice can be characterized by its seasonal variability. It reaches maximum extent during winter and minimum in summer, a dynamic greatly influenced by temperature and environmental conditions.
The complexity of Arctic sea ice is also evident in its physical structure. Besides the surface ice, there are layers of melt ponds and ridges formed by dynamic forces. These structures can be analyzed using formulas that consider ice stress and strain rates: \[ \sigma = E\varepsilon \]where \(\sigma\) is stress, \(E\) is the modulus of elasticity, and \(\varepsilon\) is strain.
A practical example of changing Arctic sea ice characteristics is evident in the reduction of multi-year ice, which is thicker and less salty compared to first-year ice. This transition impacts habitat availability for species like polar bears and seals.
Sea Ice Convection Processes
Convection processes within sea ice occur due to temperature gradients and salinity differences, leading to vertical and horizontal movements. These processes are crucial for nutrient mixing and biological activity within sea ice environments.The convection currents can be analyzed through buoyancy-driven flow equations: \[ B_f = g \left( \frac{\Delta\rho}{\rho} \right) \]where \(B_f\) represents buoyancy force, \(g\) is gravitational acceleration, \(\Delta\rho\) is the change in density, and \(\rho\) is the reference density.
Sea ice convection involves fluid flow driven by density differences due to temperature and salinity gradients, facilitating heat and matter exchange within the ice-water system.
Convection within sea ice can enhance nutrient availability for algae, promoting biological productivity.
In polar regions, brine rejection during sea ice formation increases salinity in surrounding waters, initiating convection currents that influence ocean circulation patterns.
Sea ice convection plays a significant role in the thermohaline circulation, which is a global-scale movement of ocean water driven by differences in temperature and salinity. This process can be visualized using the concept of the 'Great Ocean Conveyor Belt'. The mathematical representation is influenced by the Stommel-Arons model addressing meridional overturning circulation dynamics.
dynamics of sea ice - Key takeaways
- Dynamics of sea ice encompasses the processes of formation, deformation, and melting, influencing the global climate system.
- Sea ice is formed when ocean water freezes, creating frazil ice, and eventually solidifying into nilas, with thickness ranging from a few centimeters to several meters.
- Sea ice dynamics include processes like rafting and ridging due to wind, currents, and temperature changes, playing a key role in sea ice movement and deformation.
- The Biology of sea ice ranges from algae that are primary producers to predators including fish and mammals, maintaining the biodiversity and ecological roles within sea ice ecosystems.
- Sea ice convection is driven by temperature and salinity gradients, crucial for nutrient mixing and biological activity within the sea ice environment.
- The impact of climate change on sea ice includes alterations in its extent, thickness, and movement, with significant effects on global climate systems and the Arctic sea ice.
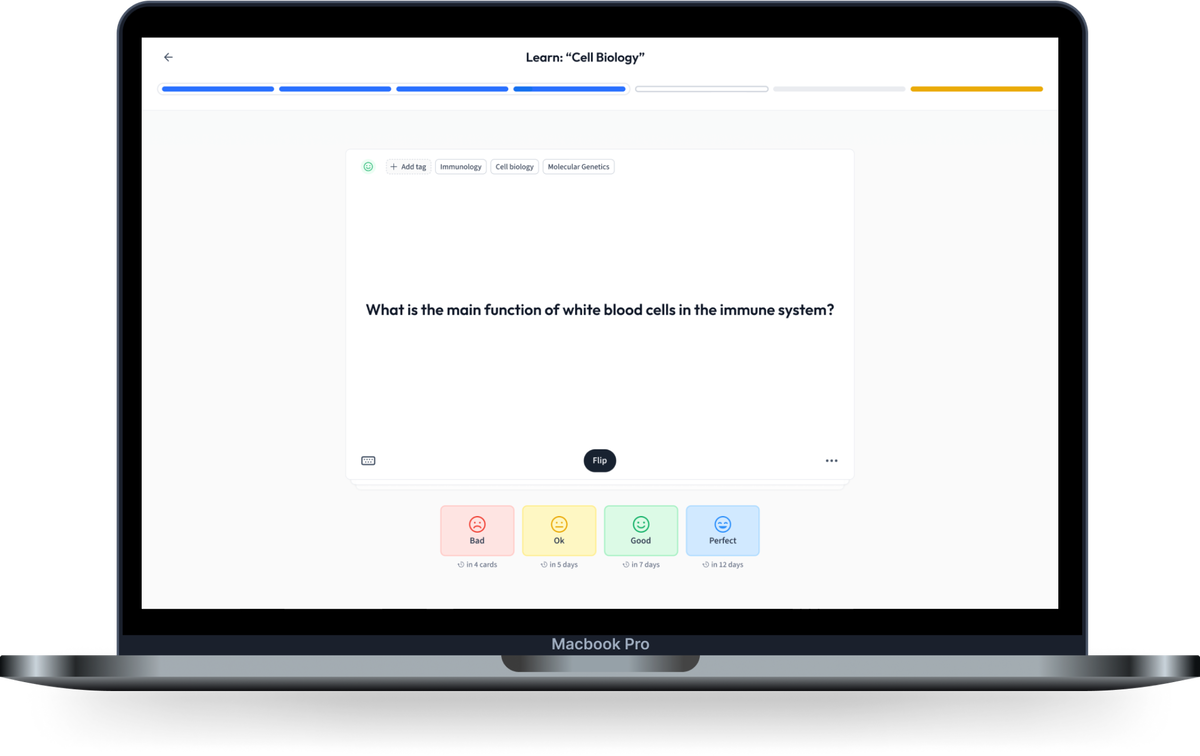
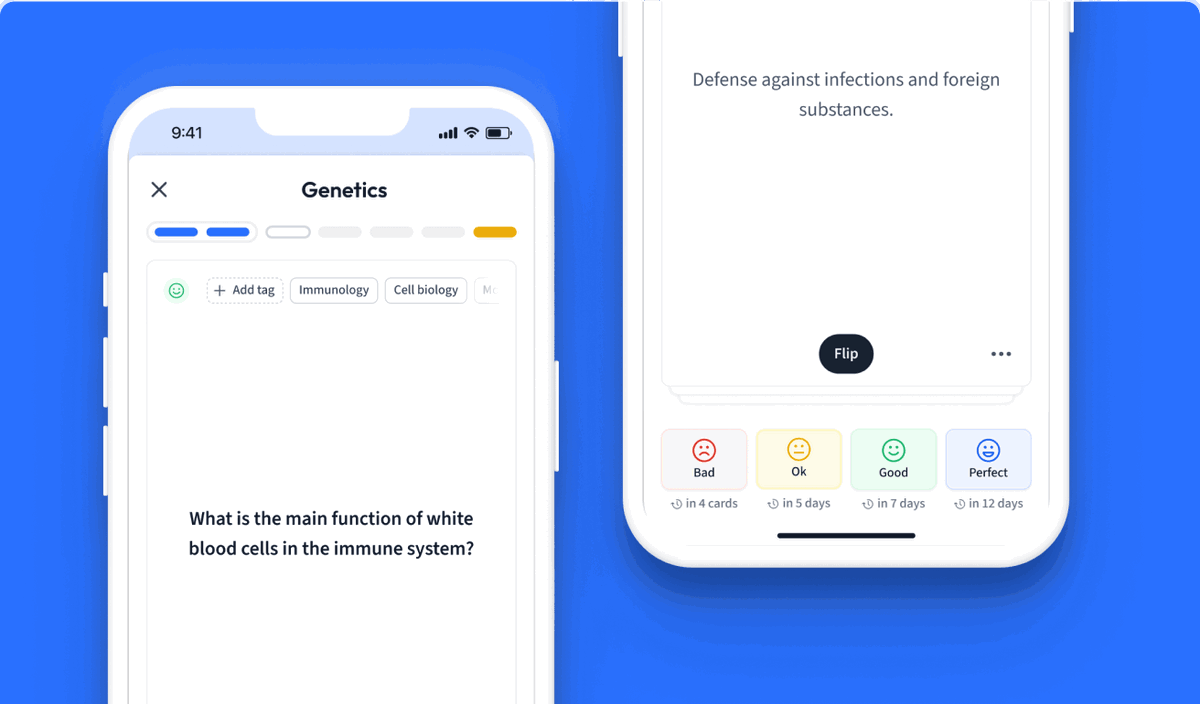
Learn with 24 dynamics of sea ice flashcards in the free StudySmarter app
Already have an account? Log in
Frequently Asked Questions about dynamics of sea ice
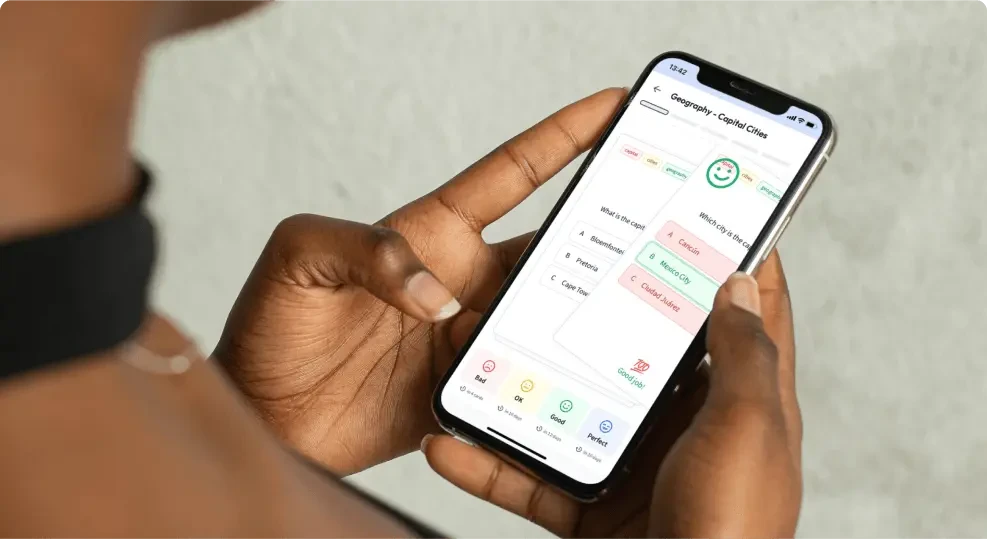
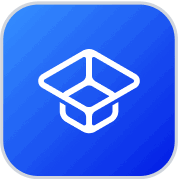
About StudySmarter
StudySmarter is a globally recognized educational technology company, offering a holistic learning platform designed for students of all ages and educational levels. Our platform provides learning support for a wide range of subjects, including STEM, Social Sciences, and Languages and also helps students to successfully master various tests and exams worldwide, such as GCSE, A Level, SAT, ACT, Abitur, and more. We offer an extensive library of learning materials, including interactive flashcards, comprehensive textbook solutions, and detailed explanations. The cutting-edge technology and tools we provide help students create their own learning materials. StudySmarter’s content is not only expert-verified but also regularly updated to ensure accuracy and relevance.
Learn more