Jump to a key chapter
Estuarine Chemistry Explained
Estuarine chemistry is a fascinating study of the chemical processes that occur in estuaries, where freshwater from rivers meets and mixes with saltwater from the ocean.
The Significance of Estuarine Chemistry
Understanding the chemical interactions in estuaries is crucial because these areas are dynamic and support diverse ecosystems. They act as nurseries for various marine species and are vital for the water filtering process. Estuarine chemistry can influence the availability of nutrients and the presence of pollutants, which have significant implications for biological productivity.
Key chemical characteristics of estuarine waters include:
- Salinity gradients, as freshwater mixes with seawater.
- Variations in pH levels that can impact organism health.
- The presence of nutrients like nitrogen and phosphorus.
Salinity and Mixing Patterns
The salinity of estuarine waters is not constant and depends on the interaction between freshwater input and tidal mixing. This creates distinct layers of water with varying salinity levels. Key concepts in understanding salinity include:
- Halocline, which is a layer where the salinity changes more rapidly with depth.
- Brackish water is the typical condition in estuaries, denoting a mix of fresh and saltwater.
Salinity affects many chemical processes, including the solubility of gases and minerals. As the salinity increases or decreases, the water's ability to hold oxygen (O2) or carbon dioxide (CO2) changes.
Calculate the salinity in a simple estuarine model where the river water (0 psu) and seawater (30 psu) mix equally. If 1 liter of river water mixes with 1 liter of seawater, the resulting salinity (S) is:
\[S = \frac{0 \text{ psu} + 30 \text{ psu}}{2} = 15 \text{ psu}\]Nutrient Cycles and Their Impact
Estuaries are significant in nutrient cycling, which involves the transformation and movement of nutrients like nitrogen and phosphorus. These nutrients play a vital role in supporting marine life and primary productivity. Nutrients in estuarine environments can come from:
- Runoff from agricultural lands.
- Discharge from wastewater treatment plants.
- Natural cycling through plant and animal life.
However, excessive nutrients can lead to eutrophication, causing harmful algal blooms and depleting oxygen levels, which can be detrimental to aquatic life.
To understand how nutrient concentration affects algal growth, suppose you have an estuary with different phosphorus levels. Plotting algal biomass (B) against phosphorus concentration (P) might show:
\[B = 0.8P - 0.6\]This simple linear model indicates that as phosphorus concentration increases, so does algal biomass, up to a certain threshold.
Estuarine environments are complex due to their fluctuating nature. Elements such as nitrogen and phosphorus undergo several transformation processes. Nitrogen can transition between forms like ammonium (NH4+), nitrate (NO3-), and gaseous nitrogen (N2), affecting the overall nitrogen budget. These transformations are mediated by biological processes like nitrification and denitrification.
The role of chemical reactions with estuarine sediments is equally important. For example, redox reactions in sediments can release or absorb elements such as iron and manganese, altering their availability in the water column.
Moreover, the interaction with organic matter adds another layer of complexity. Bacteria break down organic materials, influencing the cycles of carbon, nitrogen, and oxygen. Understanding these intricate processes is crucial for managing and protecting estuarine ecosystems from the adverse effects of pollution and climate change.
Biochemical Processes in Estuaries
The unique environment of estuaries harbors complex biochemical processes that play vital roles in maintaining the health of these ecosystems. Estuarine environments are characterized by variable salinity levels, diverse habitats, and the influence of both marine and freshwater sources, leading to intricate biochemical dynamics.
Photosynthesis and Primary Production
Photosynthesis is a crucial biochemical process in estuaries, performed by phytoplankton and aquatic plants. Through this process, these organisms convert sunlight into chemical energy, producing oxygen as a byproduct. This not only supports the primary production but also forms the foundation of the aquatic food web.
Key factors influencing photosynthesis in estuaries include:
- Availability of sunlight.
- Nutrient concentrations.
- Presence of pollutants and their impact on light penetration.
Did you know that estuaries often have higher primary productivity rates compared to the open ocean due to nutrient-rich waters?
Decomposition and Nutrient Cycling
In estuaries, the decomposition of organic matter is another essential biochemical process. This process is primarily carried out by bacteria and fungi, which break down dead plant and animal material, releasing nutrients back into the water column and sediment, making them available for uptake by primary producers.
Important aspects of decomposition in estuaries include:
- Role in nutrient recycling.
- Influence on sediment composition.
- Interaction with environmental factors like oxygen levels.
The rate of decomposition in estuaries can vary significantly depending on environmental conditions. In well-oxygenated waters, aerobic bacteria efficiently break down organic matter, releasing carbon dioxide, water, and nutrients. However, in areas where oxygen is limited, anaerobic bacteria take over the process, producing different byproducts such as methane and hydrogen sulfide, which can impact water quality and smell.
Additionally, certain estuarine environments can experience what is known as anoxic events, where oxygen levels fall to critically low levels. During such events, the entire biochemical processing of organic material shifts, with significant implications for nutrient cycling and energy flow within the ecosystem.
Biogeochemical Interactions
Biogeochemical interactions in estuaries involve the cycle and transformation of chemical elements between the living (biological) and non-living (geological and chemical) components of the environment. These interactions are crucial for maintaining ecosystem stability, influencing sediment formation, and regulating essential nutrients like nitrogen and phosphorus.
Estuaries serve as a critical zone for:
- Transformation of organic and inorganic materials.
- Integration of terrestrial and coastal nutrient cycles.
- Filtration and modification of materials before they enter the ocean.
Biogeochemical Cycle: This term refers to the natural pathway through which essential elements circulate through the biological and geological components of the Earth. In estuaries, this cycling is highly dynamic due to the interplay between land, fresh water, and marine influences.
Estuarine Nutrient Cycles
Estuarine nutrient cycles are integral processes that govern the exchange and recycling of nutrients in estuarine ecosystems. These cycles support the biological productivity and sustainability of estuarine habitats, balancing the ecosystem's health and resilience.
Nitrogen Cycle
The nitrogen cycle in estuaries involves various transformations and interactions that are crucial for supporting aquatic life. Nitrogen is an essential element, contributing to the growth of organisms. In estuarine settings, the nitrogen cycle encompasses several key processes:
- Nitrogen fixation: Conversion of atmospheric nitrogen (N2) into ammonium (NH4+) by bacteria.
- Nitrification: Conversion of ammonium into nitrites (NO2-) and then nitrates (NO3-), making it available to plants.
- Denitrification: The return of nitrogen to the atmosphere by converting nitrates into nitrogen gas.
Imagine an estuary where nitrification plays a vital role. The conversion process here might follow the equation:
\[ \text{Ammonium (NH}_4^+\text{)} \rightarrow \text{Nitrite (NO}_2^-\text{)} \rightarrow \text{Nitrate (NO}_3^-\text{)} \]This transformation enhances nutrient availability for primary producers.
Phosphorus Cycle
The phosphorus cycle in estuaries is vital for energy transfer and the formation of genetic material in organisms. Unlike nitrogen, phosphorus does not have a gaseous phase; hence it primarily cycles through water, soil, and sediments. Important aspects of the phosphorus cycle in estuaries include:
- Sedimentation and uptake by aquatic plants.
- Release through decomposition and leaching.
- Impact of human activities such as agriculture runoff, which can lead to eutrophication.
Phosphorus dynamics in estuaries can be intricate due to interactions with other minerals and organic materials. In sediment, phosphorus can bind with iron, creating iron-phosphate complexes that affect its bioavailability. Changes in redox conditions, often influenced by tides and biological activity, can shift phosphorus between mobile and immobile forms.
Research indicates that anthropogenic factors have significantly altered phosphorus cycling in many estuaries, increasing the risk of phosphorus surplus, which leads to nutrient pollution. Innovative estuarine management practices aim to mitigate these impacts by controlling phosphorus inputs from urban and agricultural sources.
Carbon Cycle
The carbon cycle in estuaries involves the exchange of carbon among the biological, geological, and chemical components of the ecosystem. It is essential for regulating primary production and storing carbon. The carbon cycle in estuaries incorporates the following processes:
- Photosynthesis: Conversion of CO2 to organic carbon by aquatic plants and phytoplankton.
- Respiration: Release of CO2 back into the atmosphere by organisms.
- Decomposition: Breakdown of organic matter, returning carbon to soil and water.
Estuarine environments act as significant carbon sinks, helping to mitigate climate change by storing carbon in sediments.
Estuarine Salinity Gradients
Estuarine environments are defined by complex salinity gradients, resulting from the mixture of freshwater from rivers and saltwater from the ocean. These gradients significantly impact the chemical and biological processes in estuaries.
Estuarine Chemistry Examples
Understanding the unique chemical compositions within estuaries can be achieved through various examples that illustrate the interactions of elements in these regions.
Examples of estuarine chemistry involve:
- Calcium carbonate formations, influenced by salinity and pH levels, affect shell formation in mollusks.
- Trace metals like copper and zinc can have variable solubility and availability in estuarine waters.
- The role of dissolved gases such as oxygen and carbon dioxide in supporting aquatic life and regulating chemical reactions.
Consider a scenario where the dissolution of gypsum (CaSO4) in an estuary is observed. The dissolution process can facilitate the release of sulfate ions, influencing the overall sulfate concentration which plays a role in nutrient cycling.
Freshwater | Seawater | Result |
0 mg/L | 28 mg/L | 14 mg/L Sulfate |
Estuarine systems are hotspots for biogeochemical processes largely due to their unique mixing densities and salinity features. The presence of diverse ion concentrations often results in the precipitation of certain minerals, such as calcite and aragonite, which deposit as sediment layers influencing the morphology and physical characteristics of the estuary.
Moreover, the nature of these sediments is crucial in determining the water's buffering capacity against pH changes, impacting species that inhabit these environments.
Salinity gradients can vary not only with tides but also seasonally, impacting nutrient cycles and organism distribution.
Importance of Estuarine Chemistry in Biology
The study of estuarine chemistry is integral to understanding biological functions within these diverse ecosystems. Chemical interactions in estuaries affect the availability of essential nutrients, habitats, and the survival mechanisms of resident species.
Key biological implications include:
- Osmoregulation in fish, which must adapt to varying salinity levels through physiological changes.
- Influence on metabolic rates of organisms due to temperature and salinity shifts.
- Nutrient concentrations affecting the growth and reproduction of plants and algae.
Osmoregulation: The process by which organisms maintain the balance of water and salts within their bodies to adapt to surrounding environmental changes, crucial for survival in estuarine habitats with fluctuating salinity conditions.
In estuarine ecosystems, the adaptability of species shows remarkable biological resilience. For instance, bivalves like oysters exhibit changes in shell composition based on the surrounding water chemistry, particularly concerning calcium availability and pH levels. This ability to adapt is essential for thriving in dynamic estuarine conditions.
In addition, estuarine vegetation such as seagrasses contribute significantly to nutrient cycling, acting as both nutrient sinks and sources, thereby supporting a complex web of life forms dependent on these critical chemical interactions.
estuarine chemistry - Key takeaways
- Estuarine Chemistry: Study of chemical processes in estuaries where freshwater meets saltwater.
- Importance: Influences ecosystem diversity, nutrient availability, and pollutant presence affecting biological productivity.
- Salinity Gradients: Variation in salinity due to freshwater and seawater mixing, affecting chemical interactions and organism health.
- Nutrient Cycles: Movement and transformation of nutrients like nitrogen and phosphorus essential for primary productivity.
- Biochemical Processes: Includes photosynthesis and decomposition crucial for energy flow and nutrient recycling in estuaries.
- Biological Implications: Affects osmoregulation, metabolic rates, and growth in organisms adapting to estuarine environments.
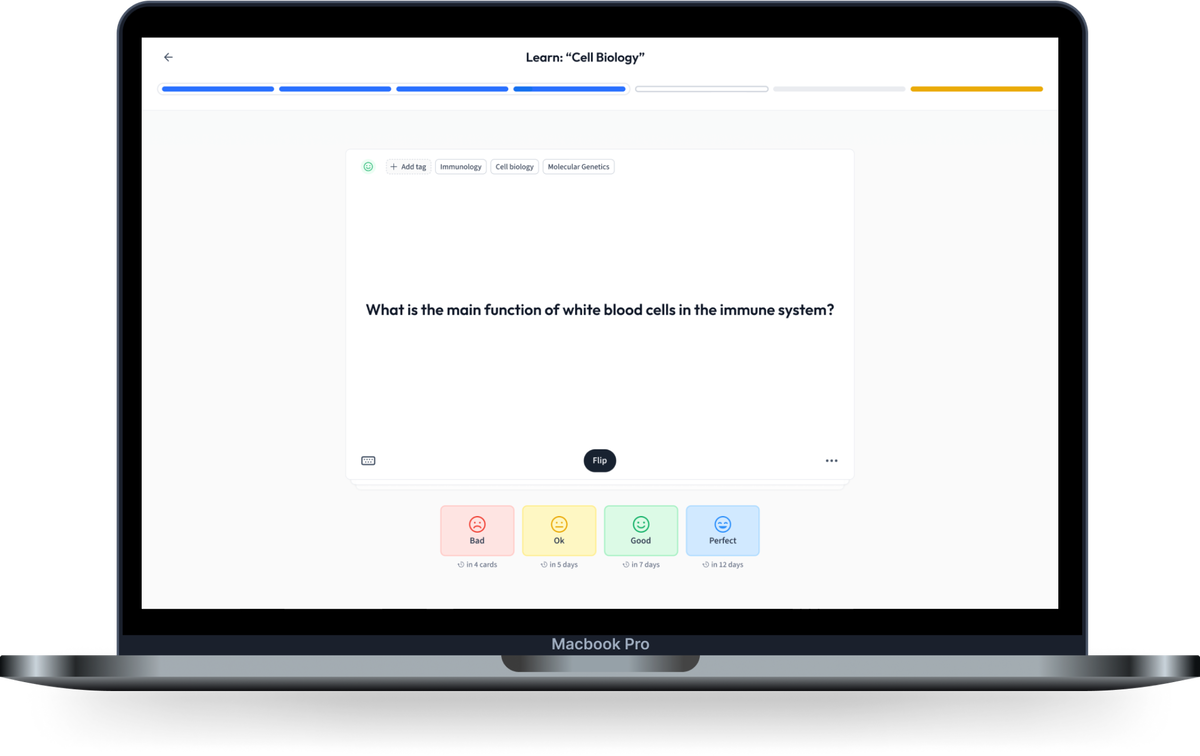
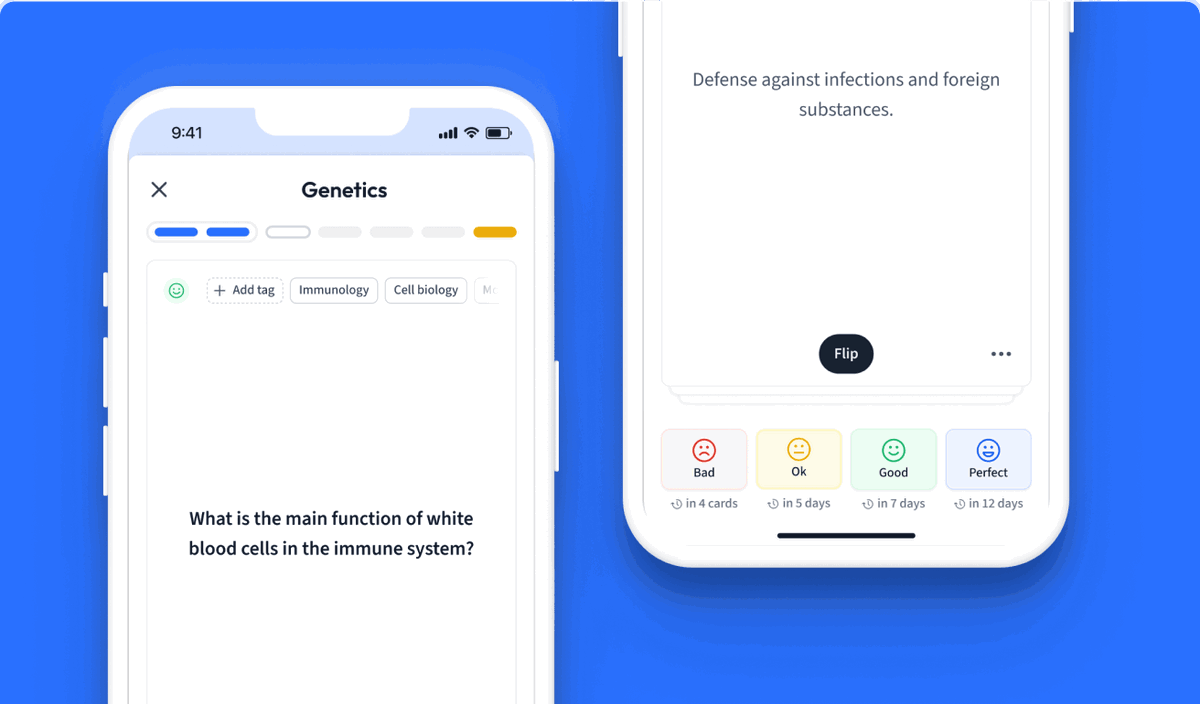
Learn with 24 estuarine chemistry flashcards in the free StudySmarter app
Already have an account? Log in
Frequently Asked Questions about estuarine chemistry
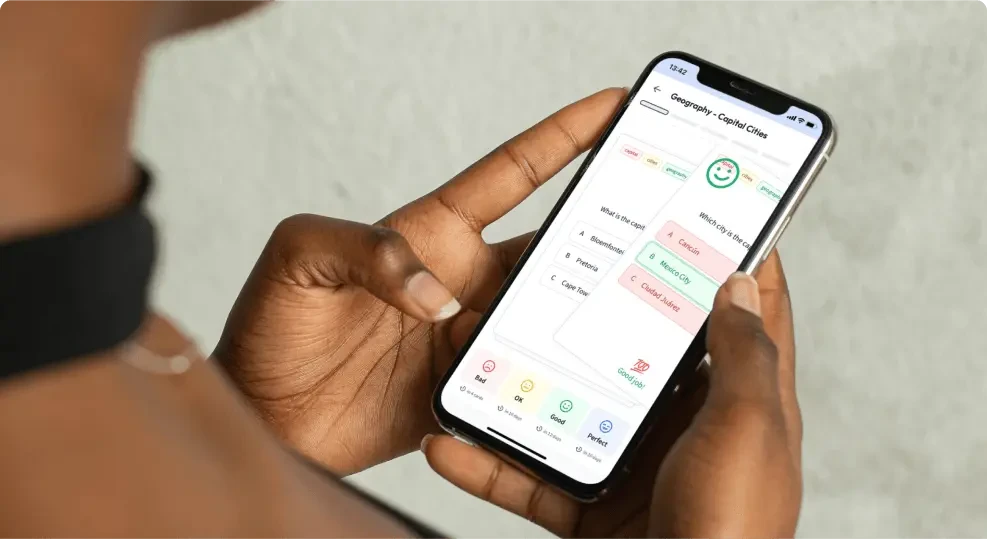
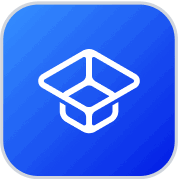
About StudySmarter
StudySmarter is a globally recognized educational technology company, offering a holistic learning platform designed for students of all ages and educational levels. Our platform provides learning support for a wide range of subjects, including STEM, Social Sciences, and Languages and also helps students to successfully master various tests and exams worldwide, such as GCSE, A Level, SAT, ACT, Abitur, and more. We offer an extensive library of learning materials, including interactive flashcards, comprehensive textbook solutions, and detailed explanations. The cutting-edge technology and tools we provide help students create their own learning materials. StudySmarter’s content is not only expert-verified but also regularly updated to ensure accuracy and relevance.
Learn more