Jump to a key chapter
Definition of Ocean Bottom Pressure
Ocean bottom pressure refers to the pressure exerted by the weight of the water column above a certain point at the ocean's bottom. This pressure is an essential factor in oceanography and environmental science as it provides critical data about ocean density and circulation patterns.
What is the Pressure at the Bottom of the Ocean
The pressure at the bottom of the ocean is determined by the weight of the water column above it. This can be extremely high due to the immense depths of the ocean. The force is measured in pascals (Pa), where 1 pascal is equal to 1 newton per square meter. To give you a sense of the magnitude, at average sea level, the pressure is about 101,325 Pa.
For example, at the deepest part of the ocean, known as the Mariana Trench, the pressure exceeds 100,000,000 Pa. This is over 1,000 times the standard atmospheric pressure that you experience at sea level.
Pressure not only depends on depth but also on the density of the water above, which can vary with temperature and salinity.
Interestingly, humans have developed advanced technology to measure the pressure at the ocean's bottom remotely. Devices like the pressure gauge and submersibles help scientists in gathering accurate data for their research. Such measurements are crucial for understanding tidal changes and seismic activities underwater.
Relationship Between Ocean Depth and Pressure
The relationship between ocean depth and pressure is linear. As the depth increases, the pressure increases proportionally. Generally, for every 10 meters of water depth, the pressure increases by approximately 1 atmosphere (about 101,325 Pa). This relationship is essential for marine biology and engineering, as it impacts everything from the buoyancy of submersibles to the physiology of marine life.
Formula for Pressure at a Given Depth: P = \/rho\*g\*h Where:
- P is the pressure
- \/rho is the density of the water
- g is the acceleration due to gravity
- h is the depth
An example to illustrate this is calculating the pressure at 500 meters depth in seawater with a density of 1025 kg/m³. Using the formula P = \/rho\*g\*h, the calculated pressure would be approximately 5,025,000 Pa.
Due to this pressure-depth relationship, deep-sea organisms have adapted unique features to survive in such environments. Their cells have a robust cell membrane structure to withstand the crushing pressures. Researchers study these adaptations to gain insights into potential applications in materials science and biotechnology.
Effects of Ocean Bottom Pressure on Marine Life
Ocean bottom pressure plays a crucial role in shaping the marine environment. It affects various aspects of marine biology and has a direct impact on how marine creatures live and thrive in their habitats.
Importance of Ocean Bottom Pressure in Marine Biology
Ocean bottom pressure is essential for understanding many processes within marine biology. It influences ocean circulation, which in turn affects nutrient distribution and temperature. These factors are vital for the health of marine ecosystems. Moreover, pressure affects the buoyancy of marine life. Many organisms have evolved to use the pressure levels at different depths to their advantage. The deeper you go, the more specialized the adaptations become. Here are some significant ways ocean bottom pressure impacts marine biology:
- Regulating buoyancy in marine creatures, allowing them to maintain their position in the water column.
- Influencing metabolic rates and biological processes of organisms adapted to high-pressure environments.
- Affects the distribution of species across various depths.
Ocean Circulation: The large-scale movement of water masses in the ocean, driven by wind, water density differences, and tides, crucial for distributing heat and nutrients.
For instance, deep-sea fish often have a reduced need for speed or efficiency and have adapted to conserving energy. These adaptations are directly influenced by the pressure at the ocean’s bottom.
A fascinating aspect of the depth-pressure relationship is how it governs the structure and behavior of deep-sea organisms. Some of these creatures exhibit bioluminescence, a phenomenon enabled by chemical reactions within their bodies, which is critically influenced by the pressure conditions they live under. Scientists are keenly studying these organisms to learn about our planet's biology and potential life on other planets.
Biological Adaptations to High Pressure Environments
Marine organisms adapt to high-pressure environments to survive and thrive. These adaptations are vital for withstanding the extreme conditions found in the deep ocean. Some examples include:
- Cell Membrane Adaptations: Deep-sea organisms often possess more flexible cell membranes to accommodate high external pressure.
- Enzyme Functionality: Many enzymes in such organisms operate efficiently at high pressures, ensuring vital biological processes can proceed.
- Structural Adaptations: The skeletal structures of deep-sea animals are often minimized to prevent compression under high pressure.
Examples of adaptation can be seen in the giant squids, which have special muscles and tissues that are compressive, and the anglerfish, which uses its bioluminescent lure to attract prey in the dark, high-pressure depths.
Research into these incredible adaptations not only enhances our understanding of marine life but also offers innovations in technology and material sciences.
Ocean Bottom Pressure and Marine Ecosystems
The dynamics of ocean ecosystems are significantly affected by the pressure at the ocean's bottom. Understanding this pressure is key to studying how marine ecosystems function and evolve under varying conditions.
Influence on Ocean Currents
Ocean bottom pressure plays a crucial role in shaping ocean currents. These currents are primarily driven by differences in water density, temperature, and salinity, all of which are influenced by the pressure at the ocean floor. The bottom pressure helps to:
- Drive the global ocean conveyor belt, a crucial component of Earth's climate system.
- Affect nutrient availability, as currents transport nutrients from deeper waters to the surface.
- Influence weather patterns and climatic conditions around the world.
Ocean Currents: Large-scale movement of water within the oceans, influenced by wind, water density differences, and tides.
A notable example of ocean currents influenced by bottom pressure is the Gulf Stream. This powerful Atlantic Ocean current carries warm water from the Gulf of Mexico up the eastern coast of the United States and across the Atlantic Ocean towards Europe.
Monitoring ocean bottom pressure helps forecast changes in major ocean currents, which is critical for understanding global climate trends.
Due to the intricate relationship between ocean bottom pressure and currents, scientists employ advanced technologies like satellite altimetry and deep-sea sensors to monitor pressure changes. These technologies provide valuable data for climate models, helping to predict how shifts in ocean currents might impact global weather patterns.
Impact on Ocean Floor Biology
Ocean bottom pressure significantly affects the biology of the ocean floor, where numerous organisms make their home. The high-pressure environment requires unique adaptations for organisms to survive. Key biological impacts include:
- Species Distribution: Pressure influences where different species can thrive, with some organisms adapted to specific pressure ranges.
- Metabolic Processes: Organism metabolism is adapted to function efficiently under high-pressure conditions.
- Reproductive Strategies: Certain deep-sea species have unique reproductive adaptations to ensure survival in high-pressure habitats.
An example of pressure adaptation is the deep-sea tube worm, which resides near hydrothermal vents. These creatures have specialized biochemistry that allows them to withstand the high-pressure and high-temperature environment.
The study of deep-sea organisms not only helps us understand life's limits on Earth but might also offer insights into potential extraterrestrial life.
Marine technology advancements, such as submersible vehicles and autonomous underwater drones, have opened up new possibilities for studying the ocean floor's complex biology. These tools allow for the collection of valuable data, such as biological samples under pressure-simulating conditions, providing deeper insights into the adaptations and functioning of deep-sea life forms.
Measuring and Studying Ocean Bottom Pressure
Understanding ocean bottom pressure is essential in oceanography, allowing scientists to study ocean dynamics, tides, and underwater geophysical events. This knowledge is critical as it helps predict sea-level changes and understand ocean circulation.
Tools and Techniques for Measuring Pressure
Several advanced tools and techniques are utilized to measure ocean bottom pressure accurately. Here is a look at some of the primary methods:
- Pressure Sensors: Deployments of pressure sensors on the seabed help provide continuous data on pressure changes. These sensors are highly accurate and can detect subtle fluctuations.
- Submersibles: These are used to manually measure pressure at different ocean depths. Equipped with pressure gauges and instruments, they collect valuable data for analysis.
- Remote Sensing: Satellite altimetry can indirectly measure ocean pressure through changes in sea surface height.
Pressure Sensor: A device that measures the pressure exerted by a fluid, offering continuous data on the pressure conditions at the ocean floor.
For example, the deployment of the Argo float system, which consists of a global array of robotic floats, provides critical pressure data, temperature profiles, and salinity readings from different parts of the world's oceans.
In recent years, researchers have been developing autonomous buoyancy-driven gliders capable of making extended, long-term pressure measurements across ocean basins. These gliders can traverse large areas while collecting high-resolution data, proving invaluable for oceanographic surveys and research.
Many oceanographic monitoring systems rely heavily on both fixed and mobile sensor platforms to ensure comprehensive data coverage.
Historical and Recent Research Developments
Research into ocean bottom pressure has evolved dramatically over the years. From the initial theories of ocean dynamics to sophisticated modern technologies, there has been substantial progress.Historically, early measurements were limited by the technology available, often relying on manual depth and pressure recording techniques. These methods have become more refined and technologically advanced over time.
- Data Repositories: Today, vast databases store ocean pressure data collected from multiple sources, offering researchers immediate access to historical and recent trends.
- Technological Innovations: Innovations in data logging and analysis have significantly enhanced the accuracy and scope of pressure measurements.
- International Collaborations: Countries and institutions worldwide collaborate to standardize methods and share data, advancing global understanding of oceanic processes.
A notable development is the Global Ocean Observing System (GOOS), which coordinates activities and networks collecting oceanographic data, including pressure. This system enhances the ability to predict ocean conditions accurately.
The integration of artificial intelligence and machine learning in analyzing ocean pressure data is rapidly advancing predictive capabilities in ocean sciences.
One of the cutting-edge areas of research focuses on underwater seismology. Scientists are using ocean bottom pressure data to study seafloor movements and seismic activity with greater precision, offering insights into earthquake prediction and underwater volcanic activity. This interdisciplinary approach enriches our understanding of both oceanic and geophysical sciences, highlighting the interconnectedness of Earth's systems.
ocean bottom pressure - Key takeaways
- Definition of Ocean Bottom Pressure: The pressure exerted by the weight of the water column at a specific point on the ocean floor, crucial for oceanography.
- Pressure at the Bottom of the Ocean: Extremely high due to immense depths; measured in pascals (Pa), exceeding 100,000,000 Pa in the Mariana Trench.
- Relationship Between Ocean Depth and Pressure: Linear; for every 10m of depth, pressure increases by approximately one atmosphere.
- Importance in Marine Biology: Influences ocean circulation, nutrient distribution, and temperature, affecting marine ecosystems.
- Biological Adaptations: Marine organisms develop adaptations like flexible cell membranes and specialized enzymes to withstand high-pressure environments.
- Effects on Marine Life: Regulates buoyancy, influences metabolism, and determines species distribution across different depths.
Learn faster with the 24 flashcards about ocean bottom pressure
Sign up for free to gain access to all our flashcards.
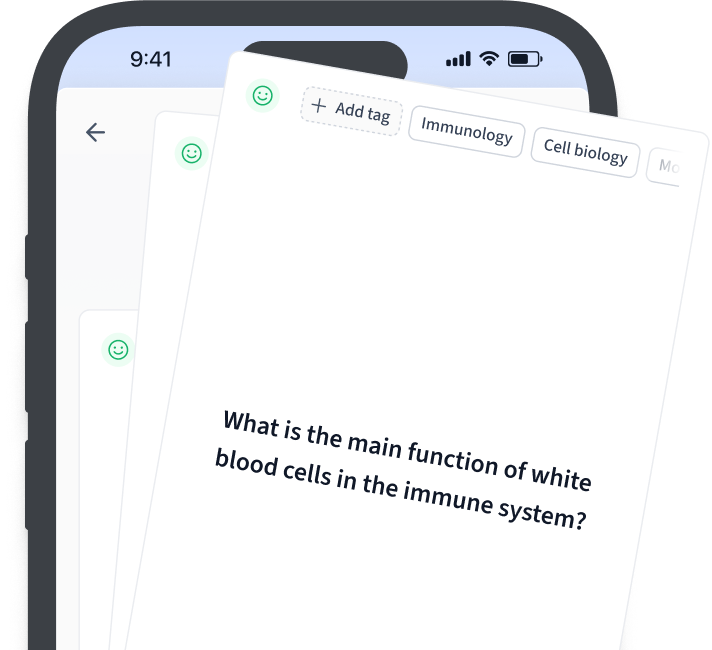
Frequently Asked Questions about ocean bottom pressure
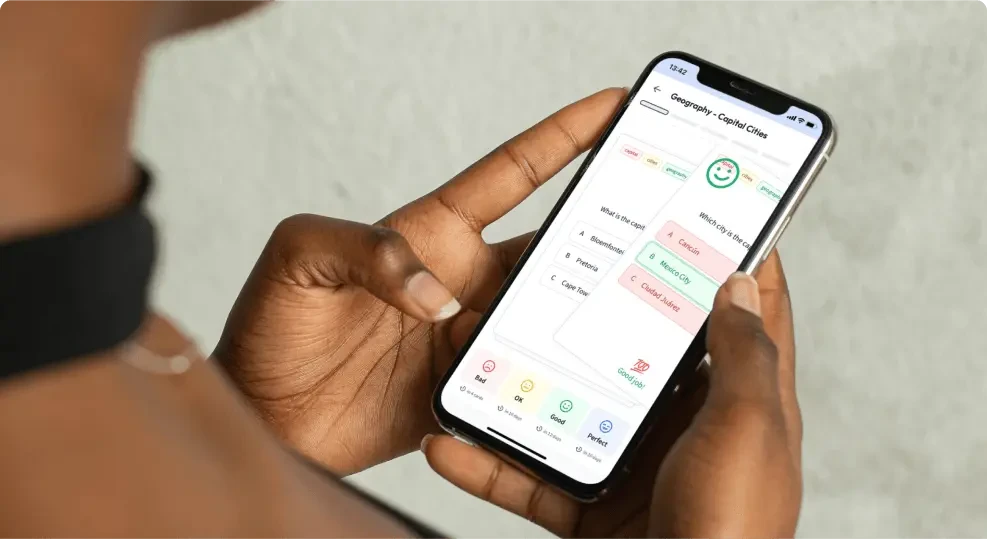
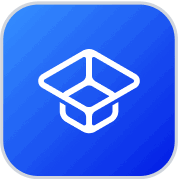
About StudySmarter
StudySmarter is a globally recognized educational technology company, offering a holistic learning platform designed for students of all ages and educational levels. Our platform provides learning support for a wide range of subjects, including STEM, Social Sciences, and Languages and also helps students to successfully master various tests and exams worldwide, such as GCSE, A Level, SAT, ACT, Abitur, and more. We offer an extensive library of learning materials, including interactive flashcards, comprehensive textbook solutions, and detailed explanations. The cutting-edge technology and tools we provide help students create their own learning materials. StudySmarter’s content is not only expert-verified but also regularly updated to ensure accuracy and relevance.
Learn more