Jump to a key chapter
Sea Ice Research Overview
Understanding sea ice is crucial for comprehending global climate patterns. Sea ice research examines the formation, movement, and melting of sea ice in polar regions. It provides insights into climate change effects and helps predict future environmental changes.
Why Sea Ice Research Matters
Sea ice research plays a crucial role in understanding Earth's climate system. Researchers study sea ice to:
- Understand the climate impact on polar ecosystems.
- Monitor changes in sea level rise.
- Assess impacts on indigenous communities.
- Track global temperature variations.
Sea ice is frozen ocean water that forms, grows, and melts in the ocean. It differs from icebergs, glaciers, and ice shelves, which originate on land.
Example: During the Arctic summer, a significant portion of the sea ice melts, affecting local wildlife, such as polar bears that rely on sea ice for hunting seals.
Did you know? Unlike freshwater ice, sea ice can be salty, but its salt content is much lower due to the expulsion of salts during the freezing process.
Methods of Sea Ice Research
Researchers utilize various methods to study sea ice, each offering unique insights:
- Satellite Observations: Provide large-scale data on ice extent and concentration.
- Field Studies: Involves direct measurement of ice thickness and composition in situ.
- Climate Models: Used for simulating future sea ice conditions.
Satellite technology has revolutionized the study of sea ice. Modern sensors can detect changes in ice thickness and movements, offering continuous global coverage. These advancements help scientists gather an immense volume of data, aiding predictions on sea ice trends and climate shifts. Despite their utility, satellites do have certain limitations; they might struggle to differentiate between ice types, which poses a challenging aspect of analyzing specific conditions within the polar regions.
Impact of Climate Change on Sea Ice
Climate change profoundly affects sea ice, resulting in:
- Decreased Ice Extent: Faster melting rates diminish ice cover, especially in the Arctic.
- Thinning Ice Layers: Ice becomes thinner due to warmer temperatures and altered precipitation patterns.
- Albedo Effect: Loss of reflective ice surfaces leads to more heat absorption, further accelerating warming.
Conducting Sea Ice Research Techniques
Conducting research on sea ice involves a variety of techniques designed to gather information about its characteristics and changes over time. These methods are essential for understanding the role of sea ice in the climatic and biological systems of our planet.
Field Techniques for Sea Ice Research
Field techniques play a critical role in gathering direct data on sea ice. Researchers typically use a combination of on-site measurements and remote sensing to collect valuable information:
- Drilling and Coring: Drilling into the ice sheet allows scientists to extract ice cores which provide data on ice formation, thickness, and age.
- Snow-depth Measurements: These measurements help assess the insulating properties of snow on top of the ice, which affects melting rates.
- Ice Buoys: Deployed on the ice surface, they relay real-time data on temperature and movement.
- Remote Cameras: Installations capturing time-lapse images, crucial for monitoring seasonal changes.
Example: Field researchers in Antarctica use core samples to examine trapped gas bubbles within the ice, which offers clues about past atmospheric conditions.
Field research, while effective, poses numerous challenges such as extreme weather conditions, potential equipment malfunctions, and limited accessibility to some areas. To overcome these hurdles, coordination with logistical support teams and the use of robust, specially designed equipment are essential. The integration of autonomous underwater vehicles (AUVs) has also advanced field techniques by enabling scientists to gather data from beneath the ice, with minimal human intervention. This expansion of capabilities significantly enhances data coverage and reliability.
Methods to Study Sea Ice Biology
Studying the biological aspect of sea ice involves understanding the organisms that inhabit these icy environments. These organisms form a crucial part of the polar ecosystems.
- Biological Sampling: Sampler Nets and Ice Scrapers are used to gather microscopic algae and zooplankton living on or within the ice.
- Microscopic Analysis: Identifying species and assessing biodiversity through microscopic examination.
- Genetic Fingerprinting: Allows for detailed analysis of genetic material to study species adaptation to cold environments.
- Remote Sensing: Using specialized sensors to detect chlorophyll concentration, which indicates the presence of algae.
Interesting fact: Algae on sea ice can contribute to its melting by absorbing sunlight due to their dark pigments, warming the ice surface more rapidly.
Passive Microwave Remote Sensing for Sea Ice Research
Passive microwave remote sensing is a key technique in sea ice research, offering unique insights into ice cover and conditions regardless of weather and light. This method collects the natural microwave radiation emitted by the Earth and its atmosphere to determine sea ice properties.
How Passive Microwave Remote Sensing Works
Passive microwave remote sensing uses satellites equipped with radiometers to collect data. These instruments measure the intensity of microwave radiation, which varies based on the emitting surface characteristics, including temperature and salinity.
The emission is affected by several factors:
- Temperature: Colder surfaces emit less microwave radiation.
- Surface Roughness: Rougher surfaces scatter radiation more.
- Salinity: Salinity levels influence the dielectric properties of ice.
Radiometers are devices used in remote sensing to measure the intensity of electromagnetic radiation within specific frequency bands.
Example: Microwave sensors on satellites like NASA's Aqua and Terra have provided continuous monitoring of sea ice, revealing significant trends in ice loss over the decades.
Did you know? Passive microwave sensors can penetrate clouds, making them ideal for monitoring remote polar regions that often experience cloudy conditions.
Advantages of Passive Microwave Remote Sensing
Passive microwave remote sensing provides several advantages in the study of sea ice, particularly in the challenging environment of polar regions:
- All-Weather Observation: Capable of capturing data regardless of cloud cover.
- Day and Night Operation: Since it relies on natural emissions, it can function without sunlight.
- Long-Term Data Acquisition: Consistent data collection over decades allows for long-term climate analysis.
One fascinating aspect of passive microwave sensing is its ability to discern between first-year and multi-year sea ice. The difference in their dielectric properties due to salinity and structural composition leads to variations in emitted microwave radiation, offering insights into ice dynamics and age. Predicting ice age helps in understanding melting patterns and contributes to accurate climate models predicting future trends.
Challenges in Microwave Sensing
Although passive microwave remote sensing offers several benefits, it also comes with a set of challenges:
- Low Spatial Resolution: Microwave sensors generally offer coarser resolution compared to optical sensors, which may limit detail.
- Calibration Needs: Precise calibration is necessary to distinguish between ice and open water radiation signatures.
- Ice Type Differentiation: Challenges exist in accurately differentiating between ice types under mixed conditions.
Despite these challenges, integrating data from multiple sources and advances in technology continue to enhance the accuracy and effectiveness of passive microwave remote sensing in sea ice research.
Biological Impact of Sea Ice Melting
The melting of sea ice has profound effects on biological ecosystems, particularly in the Arctic region. Sea ice provides a habitat and hunting ground for many species, and its loss can dramatically alter these ecosystems.
Ecological Consequences of Ice Melt in the Arctic
The Arctic is home to a delicate and unique ecosystem that is heavily dependent on sea ice. The consequences of ice melt in this region are broad and affect many aspects of the environment.
- Habitat Loss: Many species, such as polar bears and seals, rely on sea ice for resting, breeding, and hunting. The reduction of ice cover disrupts their natural habitats.
- Changes in Food Webs: Sea ice algae form the base of the food web. As ice melts, these algae diminish, impacting species that feed on them, like zooplankton, and by extension, all species higher up the food chain.
- Migration Patterns: Melting ice affects the migratory routes and breeding grounds of marine mammals and birds.
- Invasive Species: Warmer temperatures and reduced ice barriers allow for the introduction of species not traditionally found in Arctic waters, potentially threatening native wildlife.
Biological diversity, productivity, and the overall stability of the Arctic ecosystems are under threat due to these significant ecological changes. The intricate relationships between organisms and their reliance on the ice make them particularly vulnerable to rapid environmental shifts.
Sea Ice Algae: A type of algae that lives on the underside of sea ice, crucial for providing energy to the Arctic marine food web. They perform photosynthesis, thus contributing significantly to the primary production in these areas.
Example: The diminishing population of polar bears in areas where ice is retreating exemplifies the direct impact of sea ice loss on apex predators. Ice melt restricts their ability to hunt seals, leading to nutritional stress.
Fact: The loss of sea ice also influences global weather patterns. The reduction in Arctic sea ice contributes to changes in the jet stream, affecting weather systems worldwide.
Beyond the immediate effects on Arctic wildlife, the loss of sea ice has complex interactions with global climate systems. As ice melts, the albedo effect—where ice surfaces reflect sunlight—diminishes, causing dark ocean water to absorb more heat, which then contributes to further warming and ice loss in a vicious cycle known as the ice-albedo feedback. Additionally, the thawing of permafrost due to warmer temperatures releases methane, a potent greenhouse gas, further exacerbating climate change impacts. Understanding these interactions is vital for predicting future changes and implementing conservation strategies to protect these vulnerable ecosystems.
sea ice research - Key takeaways
- Sea ice research: Examines formation, movement, and melting of sea ice to understand climate impacts.
- Biological impact of sea ice melting: Affects ecosystems, food webs, and wildlife habitats in polar regions.
- Conducting sea ice research: Utilizes field techniques like drilling, remote sensing, and climate models for data collection.
- Field techniques for sea ice research: Include ice coring, snow-depth measurements, and use of ice buoys for real-time data.
- Passive microwave remote sensing for sea ice research: Measures natural radiation to assess sea ice properties, valuable for all-weather and night observations.
- Ecological consequences of ice melt in the Arctic: Lead to habitat loss, disrupted migration patterns, and introduced invasive species.
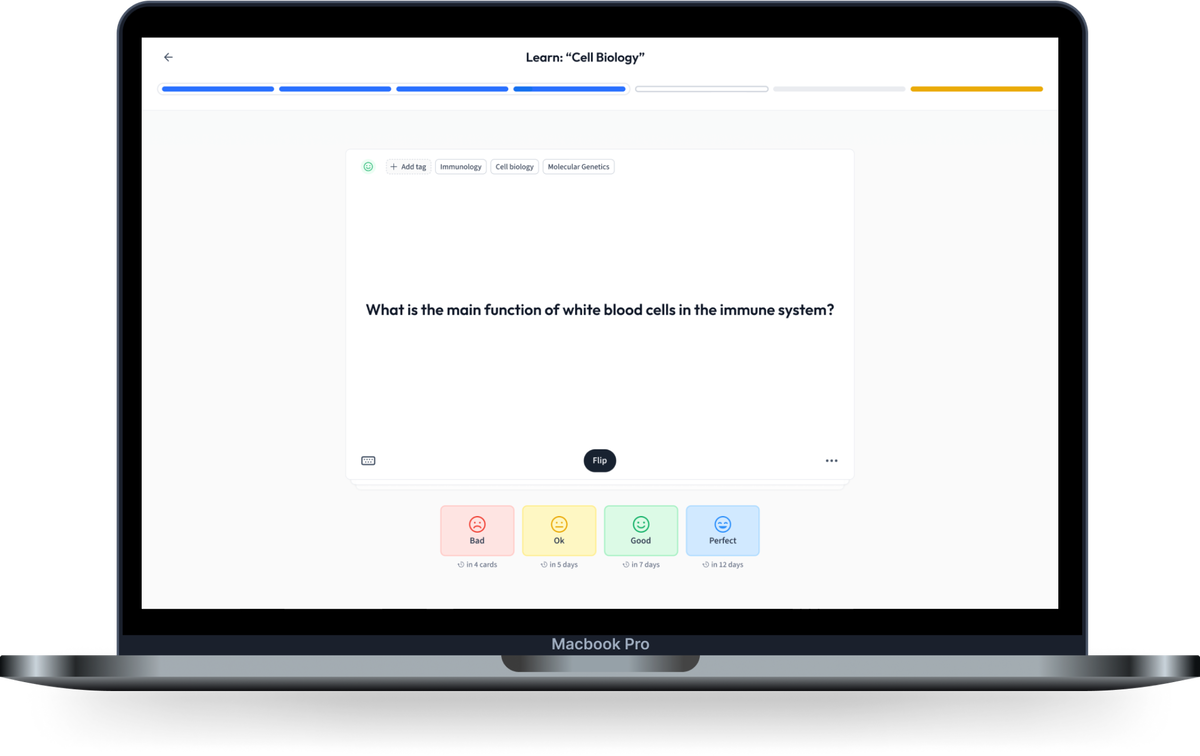
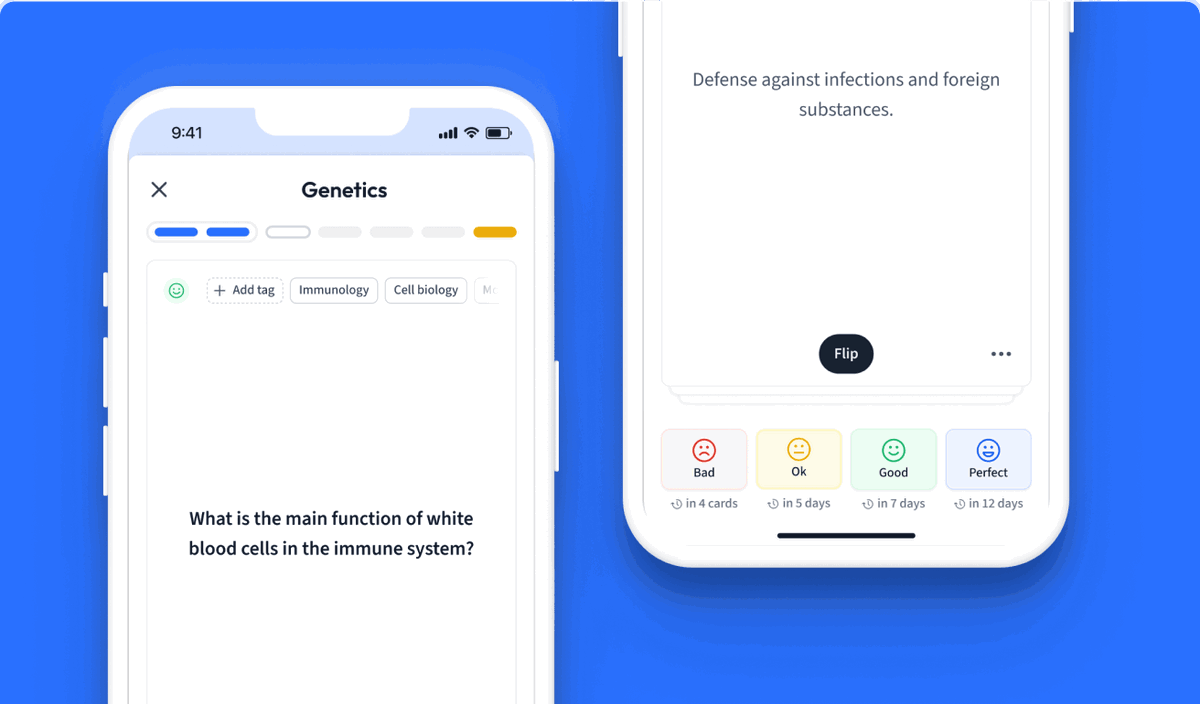
Learn with 24 sea ice research flashcards in the free StudySmarter app
We have 14,000 flashcards about Dynamic Landscapes.
Already have an account? Log in
Frequently Asked Questions about sea ice research
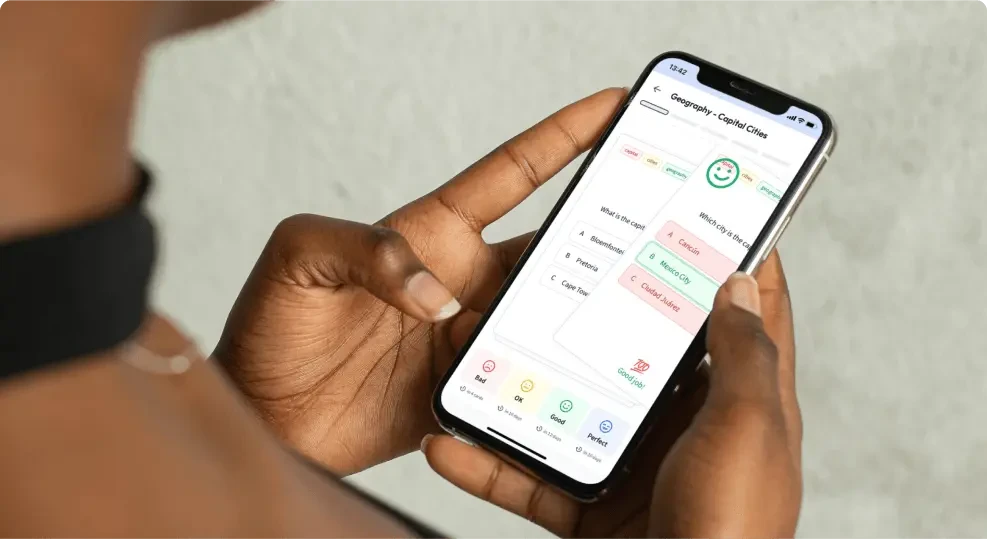
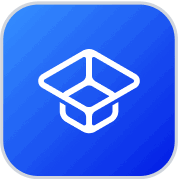
About StudySmarter
StudySmarter is a globally recognized educational technology company, offering a holistic learning platform designed for students of all ages and educational levels. Our platform provides learning support for a wide range of subjects, including STEM, Social Sciences, and Languages and also helps students to successfully master various tests and exams worldwide, such as GCSE, A Level, SAT, ACT, Abitur, and more. We offer an extensive library of learning materials, including interactive flashcards, comprehensive textbook solutions, and detailed explanations. The cutting-edge technology and tools we provide help students create their own learning materials. StudySmarter’s content is not only expert-verified but also regularly updated to ensure accuracy and relevance.
Learn more