Jump to a key chapter
Sea Surface Temperature Definition
Sea Surface Temperature (SST) is a term used to describe the temperature of the surface layer of the ocean, typically the top few meters. Monitoring SST is crucial for understanding weather patterns, climate change, and marine ecosystems. It plays a key role in numerous Earth processes, including influencing wind patterns and ocean currents.
SST is measured using various methods, including satellite observations, which provide broad coverage, and buoys, which offer precise local measurements. Satellite data can cover vast areas, providing detailed maps of temperature variations across different oceans and seas globally. Buoys, meanwhile, float on the water's surface and continually collect temperature data, contributing to long-term databases that are essential for reliable analysis.
Sea Surface Temperature (SST): The temperature of the ocean's surface layer, typically measured in the top few meters of the ocean, indicating the heat condition of the uppermost ocean layer that interacts with the atmosphere.
Think of SST like the top layer of a pot of water on the stove. If the stove is on, the surface of the water will heat up faster than the water below. Similarly, the ocean's surface temperature can change quickly, especially with direct sunlight, making it crucial for understanding short-term climate changes.
Did you know? SST variations can cause phenomena like El Niño and La Niña, which significantly impact global weather patterns.
To measure SST, scientists use infrared sensors on satellites, which detect the heat emitted by the ocean surface. These sensors collect data over large areas, offering a comprehensive view of ocean temperature changes globally. Infrared sensors provide high-resolution data, enabling scientists to detect even minute changes in the SST. They work by measuring the emitted radiation from the ocean, which correlates directly with temperature. However, these measurements can be affected by clouds, so sometimes additional instruments like microwave sensors are used.
Sea Surface Temperature Explained
Sea Surface Temperature (SST) is fundamental for understanding the Earth's climate system. It refers to the temperature of the ocean's uppermost layer, which directly interacts with the atmosphere. Monitoring SST is crucial because it can significantly impact weather patterns, marine ecosystems, and global climate.SST is influenced by several factors, including solar radiation, ocean currents, and atmospheric conditions. It is generally higher near the equator because of more direct sunlight and tends to decrease towards the poles.
There are multiple ways to measure SST, including:
- Satellite Observations: Cover large areas and provide a comprehensive picture of global SST variations.
- Buoy Measurements: Offer precise, continuous data for specific locations.
Consider an example of SST's impact: an increase in SST in the Pacific can lead to a warming event known as El Niño, altering weather patterns worldwide.
Did you know? SST data can help predict hurricane formation and intensity, as warmer waters fuel these powerful storms.
The relationship between SST and atmospheric conditions can be described by the heat transfer equation. For instance, the heat transfer from ocean to atmosphere \(Q\) can be calculated using the formula: \[Q = \rho \times c_p \times \frac{\text{d}T}{\text{d}t}\]where \( \rho \) is the density of seawater, \( c_p \) is the specific heat capacity of seawater, and \( \frac{\text{d}T}{\text{d}t} \) is the rate of change of temperature with time. This formula helps in understanding how heat energy is transferred between the ocean and atmosphere, influencing weather and climate patterns.
Techniques to Measure Sea Surface Temperature
Understanding Sea Surface Temperature (SST) is vital for tracking climate change and predicting weather events. Various techniques are used to measure SST, each with its strengths.
Satellite Measurements for Sea Surface Temperature
Satellites play a crucial role in measuring SST, providing extensive coverage and real-time data. They use infrared sensors and microwave radiometers to collect data across vast ocean areas.
- Infrared sensors detect thermal radiation emitted by the sea surface.
- Microwave radiometers can penetrate clouds, providing data even in adverse weather conditions.
For example, the Advanced Very High Resolution Radiometer (AVHRR) aboard NOAA satellites measures SST with an accuracy of approximately ±0.5°C.
Satellites like NOAA and Sentinel also help track thermal hotspots, essential for monitoring coral reef health.
Satellite data integration involves sophisticated algorithms for modeling. For instance, estimating SST from microwave sensors is done using: \[T_{s} = a + b \times T_{m} + c \times V\]where \(T_{s}\) is the sea surface temperature, \(T_{m}\) is the temperature measured by the microwave sensor, \(V\) is a variable representing environmental conditions, and \(a\), \(b\), \(c\) are constants derived from calibration.
Buoys and their Role in Measuring Sea Surface Temperature
Buoys are floating devices that provide accurate and consistent SST data. They are deployed in oceans worldwide and offer precise local measurements, contributing to long-term climate data.
- Drifting buoys follow ocean currents, gathering data over large areas.
- Moored buoys remain anchored, offering steady data sets for specific locations.
An example of buoy technology is the Argo Program, with thousands of drifting buoys worldwide, collecting data on temperature, salinity, and ocean currents at different depths.
Buoys gather data that can be used to improve climate models. For instance, consider a mathematical model used to interpret buoy data: \[T_{meas} = T_{actual} + \epsilon\]where \(T_{meas}\) is the measured temperature, \(T_{actual}\) is the actual sea surface temperature, and \(\epsilon\) denotes measurement error. Accurate buoy data help refine these models to better predict climate patterns.
Ship-based Measurements of Sea Surface Temperature
Ships are another vital resource for measuring SST, especially in data-sparse regions. They use instruments like thermometers and thermistors to record temperatures directly from the ocean surface or through water intakes.
- Thermistors offer continuous temperature records as they travel across different oceanic regions.
- Regular shipments by commercial and research vessels add valuable data for SST mapping.
For instance, research vessels equipped with modern sensors can record high-resolution SST profiles. The CLIVAR (Climate and Ocean: Variability, Predictability, and Change) program engages in systematic shipborne measurement campaigns.
Ship-based SST measurements complement satellite and buoy data by filling in coverage gaps, especially in coastal and high-latitude areas.
Ship-based observations can be modeled statistically to enhance global SST datasets. For instance, a statistical model using ship-based data might look like: \[T(x,y,t) = f(x,y) + g(t) + \delta(x,y,t)\]where \(T(x,y,t)\) represents temperature as a function of location \((x,y)\) and time \(t\), \(f(x,y)\) is a spatial component, \(g(t)\) is a temporal component, and \(\delta(x,y,t)\) is the stochastic error term. This model enables the blending of ship-based SST data with other sources to create comprehensive temperature profiles.
Causes of Sea Surface Temperature Variation
The sea surface temperature (SST) is an essential aspect of the Earth's climate system, influenced by various natural and anthropogenic factors. Understanding what causes variations in SST is crucial for climate prediction and management.
Solar Radiation and Its Impact on SST
The primary driver of variations in SST is solar radiation, which is unevenly distributed across the Earth's surface due to the planet's spherical shape. Areas near the equator receive more direct sunlight, resulting in higher SSTs compared to polar regions. The angle at which sunlight reaches the surface, known as the solar zenith angle, affects the intensity and distribution of this radiation.
For example, during summer in the Northern Hemisphere, regions like the Gulf of Mexico experience higher SSTs due to increased solar radiation, which can lead to intensified hurricane activity.
The variation in solar radiation is calculated using the formula: \[I = S_0 \times \cos(\theta)\]where \(I\) is the insolation or incoming solar radiation, \(S_0\) is the solar constant (approximately 1361 W/m²), and \(\theta\) is the solar zenith angle. This calculation helps in determining the heat received by different parts of the Earth's surface, influencing SST.
Ocean Currents and Their Influence on SST
Ocean currents play a significant role in redistributing heat across the globe. They can raise or lower SSTs by transporting warm or cold water from one region to another. These currents are driven by wind patterns, the Earth's rotation, and differences in water density. Currents such as the Gulf Stream in the Atlantic Ocean bring warm water from tropical regions towards northern latitudes, moderating temperatures along coastlines.
An example is the California Current, which flows southward along the western coast of North America, bringing cooler waters that lower SSTs along the coast.
Did you know? Ocean currents regulate climate by storing and distributing heat around the planet, impacting weather patterns and marine life.
The movement of ocean currents can be understood by the Coriolis effect, described mathematically as: \[f = 2 \times \Omega \times \sin(\phi)\]where \(f\) is the Coriolis parameter, \(\Omega\) is the angular velocity of the Earth, and \(\phi\) is the latitude. This effect causes moving water to deflect to the right in the Northern Hemisphere and to the left in the Southern Hemisphere, influencing current patterns and thus SST.
Atmospheric Conditions and Seasonal Changes
Atmospheric conditions greatly affect SST variations. Wind patterns, evaporation rates, and cloud cover all impact how heat is transferred to and from the ocean. Seasonal changes also contribute as different regions experience shifts in weather conditions, affecting SST. For instance, monsoon seasons can drastically change the temperature profile of the sea surface due to increased rainfall and cloud cover.
During La Niña events, stronger trade winds increase upwelling in the eastern Pacific, bringing cooler water to the surface and lowering SSTs.
The exchange of heat between the atmosphere and ocean can be modeled by the latent heat flux equation: \[Q = L_v \times E \]where \(Q\) is the latent heat flux, \(L_v\) is the latent heat of vaporization, and \(E\) is the evaporation rate. This flux represents the energy transferred from the ocean to the atmosphere, which influences SST.
Impact of Sea Surface Temperature on Marine Life
The sea surface temperature (SST) has a profound impact on various marine ecosystems. Changes in SST can disrupt the delicate balance of marine habitats, leading to significant consequences for marine life and biodiversity. Among the most affected are coral reefs and fish populations.
Coral Reefs and Sea Surface Temperature Changes
Coral reefs are highly sensitive to changes in SST. When temperatures rise beyond a coral's tolerance level, coral bleaching can occur, where corals expel the symbiotic algae that give them color and essential nutrients. This can lead to severe coral mortality if stressful conditions persist. Even a slight increase in SST can pose a threat to these ecosystems, often resulting in:
- Loss of biodiversity
- Disruption of marine food chains
- Increased susceptibility to disease
Coral Bleaching: A phenomenon where corals lose their vibrant colors due to the expulsion of symbiotic algae, often triggered by elevated sea temperatures.
The Great Barrier Reef, one of the most diverse ecosystems on Earth, has experienced multiple mass bleaching events due to rising SST.
Coral reefs contribute to reducing coastal erosion and support fisheries, emphasizing their environmental and economic importance.
The process of coral bleaching can be better understood through thermoregulation and energy stress models. As SST increases, corals experience oxidative stress, leading to physiological damage. Researchers use fluorescence measurements to assess coral stress levels, providing early warnings of bleaching events.Further study into the genetics of coral resilience is underway, aiming to breed 'super corals' capable of withstanding higher temperatures. This involves analyzing genetic markers associated with thermotolerance and developing coral strains based on these findings.
Fish Migration Influenced by Sea Surface Temperature
Fish populations are highly influenced by SST, which affects their distribution and migration patterns. As ocean temperatures change, fish tend to migrate towards cooler waters, which can impact:
- Availability of prey and food resources
- Spawning grounds and recruitment success
- Competition with other marine species
For instance, the North Sea has seen northward shifts in species like cod and haddock due to warmer sea temperatures, affecting local fishing industries.
SST changes also impact fish behavior, affecting their growth rates, survival, and reproduction.
The relationship between SST and fish migration is studied using climate models and fish tracking technologies. Tracking devices, like satellite tags, provide data on fish location, depth, and temperature preferences over time. This is crucial for developing sustainable fisheries management strategies.Modeling fish migration involves understanding the thermal habitat preferences of fish species. For example, a model used for Atlantic cod factors in variables like spawning temperature range and juvenile survival rates to predict shifts in distribution in response to SST increases. This information helps in the strategic planning of fisheries to mitigate economic losses and conserve fish stocks.
How Does Gyre Control Sea Surface Temperature
Ocean gyres are large systems of circular ocean currents formed by global wind patterns and the forces created by Earth's rotation. These gyres play a critical role in controlling Sea Surface Temperature (SST) by redistributing heat across the ocean's surface. Understanding their influence can offer insights into climate patterns and marine environmental changes.
Mechanics of Ocean Gyres
Ocean gyres are driven by the balance of wind forces and Coriolis effect, causing water to swirl in vast circles. There are five major ocean gyres worldwide, each significantly impacting SST in their region.The rotation and movement of water within a gyre distribute warm and cold water, affecting SST in different zones. For example, the North Atlantic Gyre carries warm water from the equator towards Europe, moderating the climate.
Gyres can be categorized into two main zones:
- Subtropical Gyres: Typically characterized by warm, nutrient-poor waters, found between the equatorial current and the poleward boundary currents.
- Subpolar Gyres: Found in higher latitudes, associated with nutrient-rich waters and colder temperatures.
The Gulf Stream, part of the North Atlantic Gyre, influences the climate of the eastern United States and Western Europe by transporting warm tropical water northward.
Subtropical gyres often have clearer waters due to reduced upwelling, which impacts marine life including primary production.
Gyres and Heat Transport
The primary function of gyres in controlling SST is through the movement of heat. As water circulates through a gyre, it gradually mixes and adjusts surface temperatures by transporting heat to cooler areas and absorbing cold water into warmer regions.This heat transport is mathematically expressed as:\[ Q = \rho \times C_p \times V \times \frac{dT}{dt} \]where:
- \( Q \) = heat content
- \( \rho \) = density of seawater
- \( C_p \) = specific heat capacity
- \( V \) = volume of water moved
- \( \frac{dT}{dt} \) = change in temperature over time
The concept of Ekman transport is vital in understanding vertical motion within gyres, where surface waters are deflected due to the Coriolis effect. The resulting movement creates a spiral effect, causing upwelling in some areas and downwelling in others. This movement significantly impacts the SST across the gyre.Numerous climate models incorporate Ekman transport for better predictions of SST changes, crucial for forecasting climate patterns and marine ecosystem dynamics.
sea surface temperature - Key takeaways
- Definition of Sea Surface Temperature (SST): The temperature of the ocean's surface layer, typically the top few meters, crucial for understanding weather patterns, climate change, and marine ecosystems.
- Techniques to Measure SST: SST is measured using satellite observations, which provide broad coverage, and buoys that offer precise local measurements. Infrared sensors and microwave radiometers are used in satellites for data collection.
- Causes of SST Variation: Variations are caused by factors like solar radiation, ocean currents, and atmospheric conditions. SST is higher near the equator and tends to decrease towards the poles.
- Impact on Marine Life: SST affects marine ecosystems, with changes disrupting habitats and leading to phenomena like coral bleaching and altering fish migration patterns.
- How Gyre Controls SST: Ocean gyres, formed by global wind patterns and the Earth's rotation, redistribute heat across the ocean's surface, influencing SST through heat transport and vertical motion.
- Importance of Monitoring SST: Monitoring SST is vital for predicting weather phenomena like El Niño and La Niña, anticipating hurricane formation, and understanding the Earth's climate system.
Learn faster with the 24 flashcards about sea surface temperature
Sign up for free to gain access to all our flashcards.
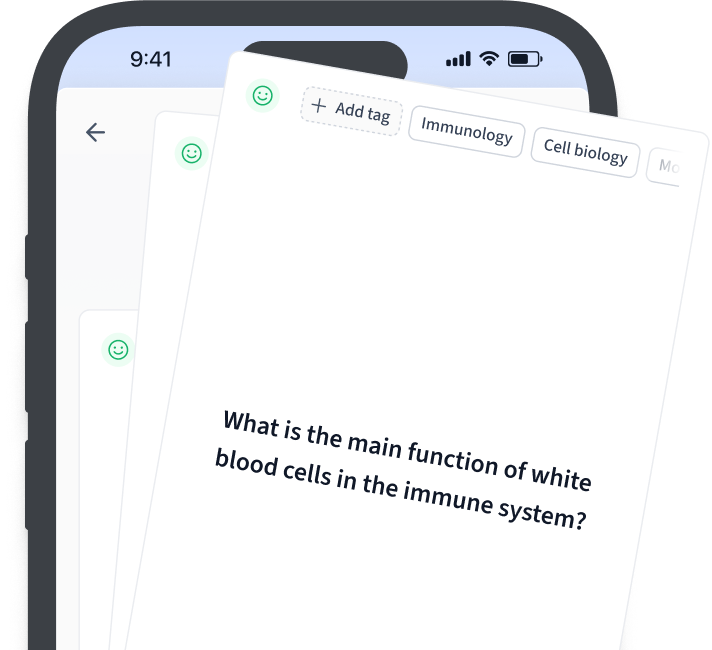
Frequently Asked Questions about sea surface temperature
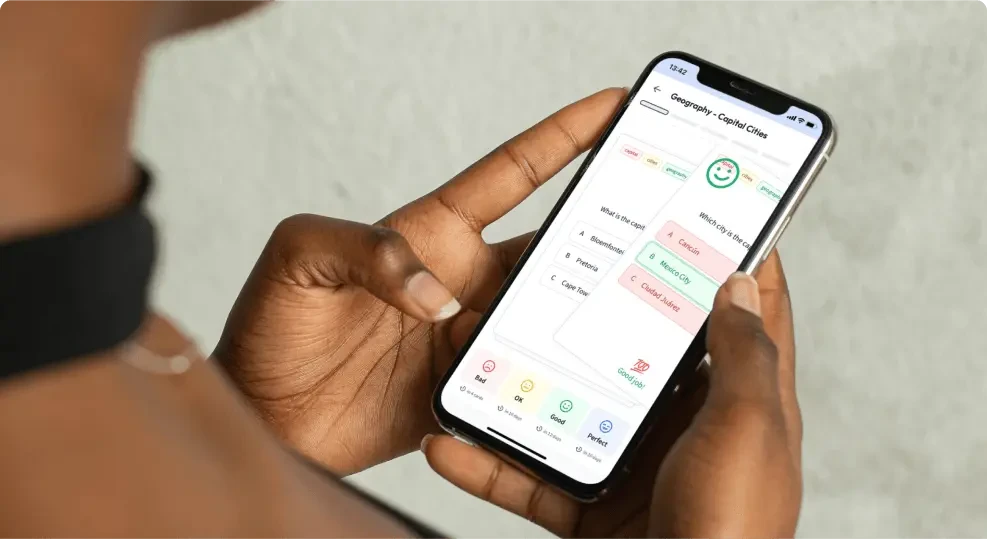
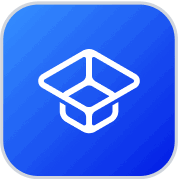
About StudySmarter
StudySmarter is a globally recognized educational technology company, offering a holistic learning platform designed for students of all ages and educational levels. Our platform provides learning support for a wide range of subjects, including STEM, Social Sciences, and Languages and also helps students to successfully master various tests and exams worldwide, such as GCSE, A Level, SAT, ACT, Abitur, and more. We offer an extensive library of learning materials, including interactive flashcards, comprehensive textbook solutions, and detailed explanations. The cutting-edge technology and tools we provide help students create their own learning materials. StudySmarter’s content is not only expert-verified but also regularly updated to ensure accuracy and relevance.
Learn more