Jump to a key chapter
What Is Nuclear Fusion?
Nuclear fusion represents a significant area of scientific research aiming at harnessing the same process that powers the sun and stars. This discipline seeks to replicate that process here on Earth to produce a clean, safe, and nearly limitless source of energy. Understanding nuclear fusion not only enhances comprehension of the universe but also holds the potential for revolutionary advancements in energy production.
Define Nuclear Fusion Reaction
A nuclear fusion reaction is a process where two light atomic nuclei combine to form a heavier nucleus, releasing a substantial amount of energy. This process is fundamental to producing the energy emitted by stars, including the sun. In stars, the gravitational force creates the extreme temperatures and pressures needed for fusion to occur. The challenge for scientists on Earth is to replicate these conditions in a controlled manner to harness the energy produced for practical use.
Nuclear Fusion Reaction: A process where two light atomic nuclei merge to form a heavier nucleus, releasing energy in the process.
The Basic Nuclear Fusion Equation Explained
The simplest and most fundamental equation for nuclear fusion involves the fusion of hydrogen isotopes to form helium, a process that releases energy. The equation can be represented as:
2H + 3H ightarrow 4He + 1n + Energy
Here, 2H (Deuterium) and 3H (Tritium) represent hydrogen isotopes, 4He represents a helium nucleus, 1n is a neutron, and Energy denotes the released energy. This equation succinctly summarises the fusion process, demonstrating the mass-to-energy conversion as per Einstein's E=mc2.
- Deuterium (2H): A hydrogen isotope with one proton and one neutron.
- Tritium (3H): A hydrogen isotope with one proton and two neutrons.
Understanding the basic nuclear fusion equation opens the door to exploring more complex reactions, such as those involving other isotopes of hydrogen or even elements like lithium. While the principle remains the same - combining lighter nuclei to form heavier ones and releasing energy - the specifics of the reactions, including the conditions required and the energies released, vary significantly. This variation underpins the ongoing research in nuclear fusion, as scientists seek the most efficient, safe, and sustainable reactions for energy production.
How Does Nuclear Fusion Work?
Nuclear fusion involves the process of combining lightweight atomic nuclei to form heavier nuclei, releasing a vast amount of energy in the process. It is the power source of the sun and holds the promise of providing a nearly inexhaustible source of clean energy for the Earth.
Understanding the Nuclear Fusion Process
The nuclear fusion process begins with the overcoming of electrostatic forces of repulsion between positively charged atomic nuclei. Once these nuclei are sufficiently close, the attractive nuclear force (strong force) outweighs the repulsive force, allowing the nuclei to combine. The resultant nucleus has slightly less mass than the sum of the original nuclei; this mass difference is released as energy according to Einstein's equation, E=mc2.
Nuclear Fusion: A nuclear reaction in which two or more atomic nuclei come close enough to form one or more different atomic nuclei and subatomic particles (neutrons or protons), with the release of energy.
An example of a nuclear fusion process is the fusion of Deuterium and Tritium, two isotopes of Hydrogen, to form Helium, a Neutron, and a significant amount of energy:
Deuterium (2H) + Tritium (3H) ightarrow Helium (4He) + Neutron (n) + Energy
- The conditions required for nuclear fusion to occur naturally exist in the cores of stars.
- Creating these conditions on Earth poses significant scientific and engineering challenges.
The Role of Extreme Temperatures and Pressures
For nuclear fusion to occur, conditions of extreme temperatures and pressures are needed. High temperatures provide the necessary energy to overcome the electrostatic repulsion between the nuclei. This is often referred to as the condition for achieving 'thermonuclear' fusion. Temperatures on the order of millions of degrees Celsius are required to initiate and sustain the fusion reaction.
Similarly, high pressures help in bringing the nuclei close enough to trigger the fusion process. In stars, the gravitational force provides this pressure. On Earth, achieving the necessary pressure and temperature for nuclear fusion is a formidable challenge and is the focus of various experimental technologies like magnetic confinement fusion (MCF) and inertial confinement fusion (ICF).
Achieving and sustaining the extreme temperatures and pressures required for nuclear fusion on Earth is complex. It involves the use of cutting-edge technologies and materials. Magnetic Confinement Fusion (MCF) uses powerful magnetic fields to confine the hot plasma (a state of matter composed of free electrons and atomic nuclei) in a controlled space. In contrast, Inertial Confinement Fusion (ICF) employs high-energy lasers or ion beams to compress and heat the fuel to fusion conditions. These approaches aim to create a self-sustaining fusion reaction that produces more energy than it consumes, a milestone known as 'ignition'.
Nuclear Fusion in the Sun
The sun, a vast and luminous sphere of hot plasma, serves as an enormous nuclear fusion reactor. At its core, nuclear fusion processes convert hydrogen into helium, releasing an immense amount of energy in the process. This energy is responsible for the sun's light and heat, making life possible on Earth.
The Sun’s Energy: A Nuclear Fusion Reactor
Within the sun's core, nuclear fusion occurs at temperatures of approximately 15 million degrees Celsius. The dense core and high temperature facilitate the fusion of hydrogen atoms into helium. This process releases vast amounts of energy, primarily in the form of gamma rays.
The energy produced in the sun's core eventually reaches the surface and then radiates into space as sunlight. This sunlight supplies the Earth with the heat and light necessary for photosynthesis, climate regulation, and supporting life.
- The sun converts about 4 million tonnes of mass into energy every second through nuclear fusion.
The Sun's ability to sustain nuclear fusion is due to its massive gravitational force, which compresses its core to conditions extreme enough for fusion to occur. The core's pressure is so intense that electrons are stripped from their atoms, creating a state of matter known as plasma. In this state, the conditions are ripe for the nuclei of hydrogen to overcome their natural repulsive force, merge, and form helium through the process of nuclear fusion. This transformation is the key to the Sun's long-lasting luminosity and stability.
From Hydrogen to Helium: Solar Nuclear Fusion
The primary reaction in the sun's nuclear fusion process involves the transformation of hydrogen to helium. This occurs through a series of steps known as the proton-proton (PP) chain reaction. The PP chain starts with the fusion of two hydrogen nuclei (protons) to form a heavy hydrogen isotope called Deuterium (2H), releasing a positron and a neutrino.
This reaction sequence proceeds through several more steps, ultimately resulting in the production of a helium-4 nucleus from four hydrogen nuclei. The process releases energy in the form of gamma rays, which is then converted to sunlight.
Proton-Proton (PP) Chain Reaction: A series of nuclear reactions by which stars convert hydrogen to helium, releasing energy.
An example of a step in the proton-proton chain reaction is:H + H ightarrow 2H + e+ + , where represents a neutrino and e+ represents a positron.
- The energy released in the Sun's core takes thousands of years to reach the Sun’s surface due to the 'random walk' process of energy transfer.
The Future of Nuclear Fusion
The quest for nuclear fusion as a feasible source of energy has been a long-standing ambition of scientists and engineers. The potential for producing clean, safe, and virtually limitless power from nuclear fusion offers a compelling future energy option.
Nuclear Fusion Reactor Development
Developing a functional nuclear fusion reactor entails overcoming substantial scientific and engineering challenges. These include achieving and maintaining the extreme temperatures and pressures needed for fusion to occur, as well as ensuring the process produces more energy than it consumes.
Recent advancements have been made in magnetic confinement fusion (MCF) and inertial confinement fusion (ICF) technologies. MCF uses powerful magnetic fields to contain and control the fusion reaction, while ICF focuses on compressing the fuel pellet to high densities using lasers or ion beams.
An example of nuclear fusion reactor development is the international ITER project, which aims to demonstrate the feasibility of fusion as a large-scale and carbon-free source of energy. ITER's approach is based on the tokamak design, a type of magnetic confinement reactor.
- The goal of ITER is to produce 10 times more energy than is required to initiate the fusion reaction.
Nuclear Fusion Breakthrough and Its Potential
A breakthrough in nuclear fusion could fundamentally change the world's energy landscape. Such a breakthrough would not only provide a bountiful source of clean energy but also substantially reduce the environmental impacts associated with fossil fuels and traditional nuclear power.
Nuclear fusion's potential extends beyond just electricity generation. It could provide heat for industrial processes, desalinate water, and even play a role in space travel by offering a compact and efficient source of power for long-duration missions.
The successful harnessing of nuclear fusion could lead to a significant reduction in greenhouse gas emissions, contributing to efforts to combat climate change. Moreover, unlike current nuclear fission reactors, fusion does not produce long-lasting radioactive waste, addressing a critical concern regarding nuclear energy's environmental footprint.
- Nuclear fusion requires fuel sources such as deuterium and tritium, which are abundant and could provide energy for millions of years.
Nuclear Fusion - Key takeaways
- Nuclear Fusion: A process in which two light atomic nuclei combine to form a heavier nucleus, releasing substantial energy, mimicking the process that powers the sun and stars.
- Nuclear Fusion Reaction Definition: The merging of two light atomic nuclei to form a heavier nucleus, notably in stars due to high temperatures and pressures, with the aim on Earth to replicate these conditions for energy production.
- Nuclear Fusion Equation: The basic equation, 2H + 3H → 4He + 1n + Energy, represents the fusion of hydrogen isotopes deuterium (2H) and tritium (3H) into helium (4He) and a neutron (1n), with energy release as per E=mc2.
- Nuclear Fusion in the Sun: The sun's core fuses hydrogen into helium at approximately 15 million degrees Celsius, releasing energy that reaches Earth as sunlight, essential for life.
- Nuclear Fusion Reactor: Devices that aim to achieve controlled nuclear fusion on Earth, with developments like the ITER project using magnetic confinement fusion (MCF) to contain and manage the process.
- Nuclear Fusion Breakthrough: The potential of nuclear fusion to provide a vast source of clean energy, reduce environmental impact, and supply deuterium and tritium fuels that could last for millions of years.
Learn faster with the 0 flashcards about Nuclear Fusion
Sign up for free to gain access to all our flashcards.
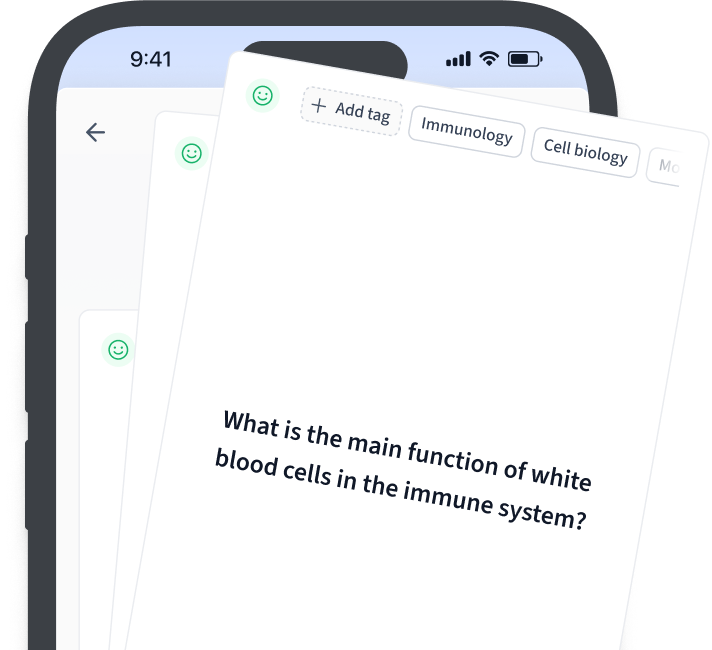
Frequently Asked Questions about Nuclear Fusion
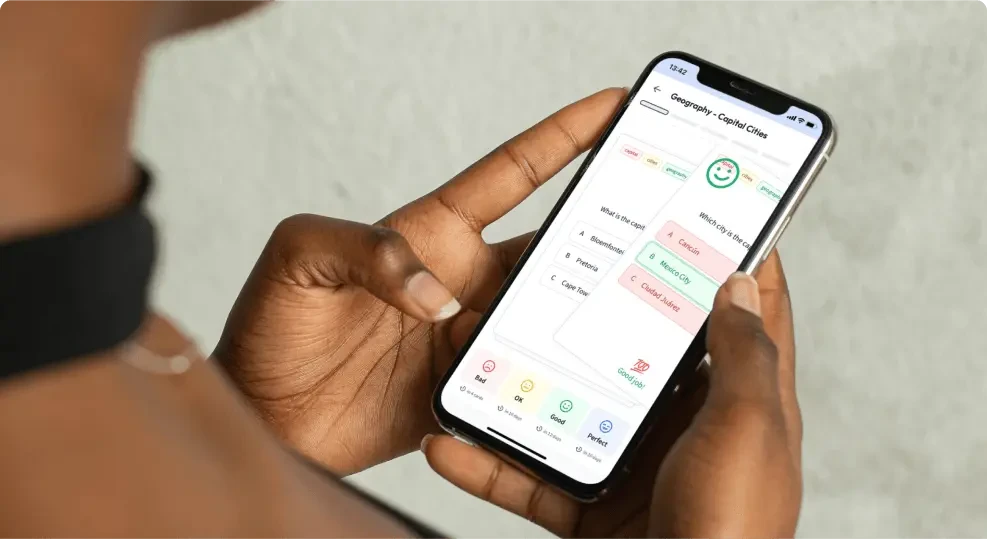
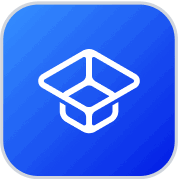
About StudySmarter
StudySmarter is a globally recognized educational technology company, offering a holistic learning platform designed for students of all ages and educational levels. Our platform provides learning support for a wide range of subjects, including STEM, Social Sciences, and Languages and also helps students to successfully master various tests and exams worldwide, such as GCSE, A Level, SAT, ACT, Abitur, and more. We offer an extensive library of learning materials, including interactive flashcards, comprehensive textbook solutions, and detailed explanations. The cutting-edge technology and tools we provide help students create their own learning materials. StudySmarter’s content is not only expert-verified but also regularly updated to ensure accuracy and relevance.
Learn more