Jump to a key chapter
Electrical Resistivity Meaning in Geography
Understanding electrical resistivity in geography provides insights into the properties of geological formations and natural landscapes. It forms a crucial part of research related to earth materials and their conductive capabilities.
Definition of Electrical Resistivity in Geography
Electrical resistivity in geography refers to the resistance encountered by an electric current as it passes through a geological material. It is expressed in ohm-meters (\(\Omega \cdot m\)). This property helps scientists determine the makeup and behavior of earth materials.
For instance, the resistivity of a typical rock might be around 1000 \(\Omega \cdot m\), while ocean water, which is a good conductor, might have a resistivity of less than 1 \(\Omega \cdot m\). Such values indicate significant differences in conductive properties and can be pivotal in environmental studies.
High resistivity materials are usually insulators, whereas low resistivity materials are conductors.
Electrical Resistivity Explained
Electrical resistivity is essential when investigating underground formations. It helps geoscientists in identifying water tables, locating mineral deposits, and observing fault lines. The formula for resistivity is given by \(\rho = R \cdot \frac{A}{L}\), where \(\rho\) is resistivity, \(R\) is resistance, \(A\) is the cross-sectional area, and \(L\) is the length of the conductor. This formula showcases how material properties and dimensions influence resistance.
- Suppose you have a test setup where the resistance measured is 200 ohms, the area is 0.5 square meters, and the length is 100 meters.
- Using the resistivity formula, \(\rho = 200 \cdot \frac{0.5}{100} = 1 \ \Omega \cdot m\).
- This calculation helps better understand the location's geological properties.
In-depth investigations using resistivity data can reveal fascinating insights. Advanced methods like Electrical Resistivity Tomography (ERT) create 3D models of subsurface structures by measuring resistivity variations. These models are instrumental in groundwater studies, waste management, and archaeological site exploration. You can think of ERT as a 'CT scan' for the earth, providing detailed images for analysis. The technique involves placing electrodes in the ground which inject and measure currents, enabling highly sophisticated interpretation of the subsurface compositions. Understanding these compositions aids in ecological conservation and resource management strategies. This not only assists scientists in academic research but also supports governmental agencies in making informed decisions about environmental policies.
Always pay attention to the type of material when considering its resistivity; soil, rock, and water all behave differently.
Applications of Electrical Resistivity in Geography
The application of electrical resistivity in geography is vast and offers invaluable insights for various fields like geology, environmental science, and civil engineering. Using resistivity measurements, scientists and engineers can explore underground formations and monitor environmental changes effectively.
Geophysical Survey and Exploration
In Geophysical Survey and Exploration, electrical resistivity plays a crucial role, helping to map the subsurface characteristics of the Earth. This method is highly beneficial for identifying and characterizing underground resources.
The process of measuring electrical resistivity involves injecting electrical currents into the ground using electrodes and measuring the resulting potential difference. This data helps in determining the resistivity of subsurface materials.
- For example, in mineral exploration, resistivity surveys can detect ore bodies by identifying areas with significantly lower resistivity.
- Similarly, groundwater surveys utilize these measurements to map aquifers as water-saturated zones have distinct resistivity values compared to dry regions.
Using resistivity data, advanced techniques like Electrical Resistivity Tomography (ERT) create detailed images of subsurface geology. These techniques reveal complex geological features and help in directing drilling operations more accurately. ERT achieves this by setting multiple electrodes in arrays, facilitating three-dimensional analysis.
Electrodes are positioned at the surface for data acquisition, and their arrangement can significantly influence the resolution of subsurface imaging.
Environmental Monitoring and Management
Electrical resistivity is also instrumental in Environmental Monitoring and Management. It aids in the detection and monitoring of environmental changes and hazards.
Environmental assessments often require understanding how pollutants move through the soil, and resistivity surveys offer a non-invasive way to track such movements. By identifying areas of high resistivity, scientists can deduce where contaminants are concentrated as they typically reduce soil resistivity. Managing these areas becomes crucial in preventing further environmental degradation.
- During soil contamination studies, resistivity surveys determine the extent of industrial waste penetration into the ground.
- In coastal areas, these surveys assess seawater intrusion by observing increases in salinity, which lowers electrical resistivity.
When managing coastal regions, monitoring changes in resistivity is vital for assessing the impact of climate change on sea intrusion patterns.
Examples of Electrical Resistivity in Geography
The practical use of electrical resistivity in geographical sciences is crucial for activities such as exploring groundwater resources and analyzing soil and rocks. It provides essential data that help in understanding and managing various geological phenomena.
Groundwater Exploration
Groundwater exploration relies heavily on measuring electrical resistivity to discover and assess aquifers. This non-invasive method is invaluable in hydrology for locating water resources with high accuracy. When electrical currents pass through the ground, areas of varying resistivity indicate different materials, such as water-filled layers.
In groundwater exploration, resistivity surveys are often equipped with advanced techniques like Vertical Electrical Sounding (VES), which provide detailed depth-related resistivity information. This technique helps in understanding the stratification of subsurface layers. For example, a typical resistivity setup consists of multiple electrodes placed at increasing distances. As the distance between electrodes increases, the penetration depth of the current also increases, allowing the collection of data from various depths. By interpreting these data with the formula \(\rho = \frac{V}{I}\cdot K\), where \(\rho\) is resistivity, \(V\) is voltage, \(I\) is current, and \(K\) is a geometrical factor, scientists can differentiate between conductive water-bearing layers and resistive, dry geological formations. This precision aids in determining water table depths and aquifer extents.
Consider an exploration area where a resistivity measurement returns a value of 50 \( \Omega \cdot m\) at a 20-meter depth, indicating a potential water-bearing layer. Compared to 150 \( \Omega \cdot m\) in adjacent dry rock, the lower resistivity assists in identifying promising groundwater sites.
Groundwater surveys using resistivity are beneficial in arid regions, where surface water is scarce.
Soil and Rock Analysis
Electrical resistivity is also a fundamental tool in analyzing soil and rocks within the field of engineering geology. Variations in resistivity values provide insights into the composition, porosity, and moisture content of these materials, which are critical for construction and land use planning.
In geological terms, electrical resistivity differentiates between soil types based on how easily they conduct electricity. This property is influenced profoundly by moisture, temperature, and mineral content, key factors in land assessment.
- Consider a land area being surveyed for construction. High resistivity values, say over 1000 \( \Omega \cdot m\), might indicate solid rock or dry soil, unsuitable for digging foundations without significant work.
- On the other hand, low resistivity values below 100 \( \Omega \cdot m\) could suggest clay-rich, waterlogged soil that might require drainage solutions before building.
The use of resistivity in soil and rock analysis extends to understanding geological hazards, like landslides or sinkholes. Electrical resistivity imaging (ERI), a more sophisticated method, creates a detailed two-dimensional resistivity profile by deploying an array of electrodes along the ground surface. This advanced technique can detect subsurface voids and identify zones of weakness in bedrock. By utilizing complex interpretations of the relationship between resistivity, \(\rho\), and soil characteristics, \(\phi\) (porosity), given by \(\rho = \rho_0(1+\phi(k-1))\),where \(\rho_0\) is the intrinsic resistivity, and \(k\) is related to the connectivity of pores, engineers and geologists obtain critical insights for site development and risk assessments.
Monitoring changes in soil resistivity over time helps in predicting landslides by identifying unstable slopes.
Techniques to Measure Electrical Resistivity
Measuring electrical resistivity is crucial in exploring geological formations and understanding subsurface properties. Various techniques are employed to measure resistivity, each with unique applications and methodologies.Below, we'll explore two commonly used methods: the Direct Current (DC) Resistivity Method and the Induced Polarization (IP) Method.
Direct Current (DC) Resistivity Method
The Direct Current (DC) Resistivity Method involves injecting a direct current into the ground through electrodes and measuring the potential difference created in the surrounding earth materials. This technique is widely used for environmental and engineering investigations as it provides straightforward resistivity data about subsurface layers. The method uses Ohm's Law, which is expressed as \(V = I \cdot R\), where \(V\) is the voltage, \(I\) is the current, and \(R\) is the resistance. From this, resistivity \(\rho\) can be calculated using the formula: \(\rho = R \cdot \frac{A}{L}\), where \(A\) is the cross-sectional area and \(L\) is the current path length.
Consider a survey where a DC current is applied using electrodes placed 10 meters apart. If a voltage of 20 volts is measured across the electrodes with a current of 2 amperes flowing, the resistance is calculated as \(R = \frac{20}{2} = 10\ \Omega\). Using the formula for resistivity, with a known electrode cross-section of 1 \( m^2 \), the resistivity is \( \rho = 10 \cdot \frac{1}{10} = 1\ \Omega \cdot m \).
The DC Resistivity Method is integral in geological investigations. Techniques such as Schlumberger and Wenner configurations differ in electrode placements and can impact data resolution. Often, a combination of configurations provides more comprehensive subsurface imaging, allowing for the identification of fault lines, water tables, and mineral deposits. The adoption of automated data acquisition systems enhances accuracy and efficiency, providing continuous profiles critical in hazard assessment and resource exploration.
Electrode configuration significantly influences the depth of investigation in DC resistivity surveys.
Induced Polarization (IP) Method
The Induced Polarization (IP) Method is a geophysical technique that measures the delayed response (polarization) of the ground to the removal of an electric current. This delay is often referred to as a chargeability effect and provides additional information about subsurface mineral properties, notably in the detection of disseminated sulfide ores.The IP effect is quantified by measuring the potential decay curve after the current is turned off. This decay can be expressed by the formula: \( V(t) = V_0 \cdot e^{-t/\tau} \), where \(V(t)\) is the voltage at time \(t\), \(V_0\) is the initial voltage, and \(\tau\) is the time constant.
In a scenario where a voltage decays from an initial 100 mV to 37 mV over 5 seconds, the time constant \(\tau\) can be calculated as \(\tau = 5\ seconds\). This measurement hints at a mineralogical variation beneath the surface, often indicative of valuable mineral presence.
The IP Method differentiates itself by its ability to detect variations in mineral composition and grain size, making it ideal for mineral exploration and environmental assessments. Enhanced by 3D modeling techniques, IP surveys enable detailed interpretations of ore body geometries. This is particularly advantageous in regions where traditional resistivity measurements might not discern fine mineral dispersion. Modern IP instrumentation facilitates multifrequency and multiparameter data acquisition, yielding insights into geological processes and prospective areas for mining.
IP methods can be particularly effective in identifying ore bodies that are not detectable by magnetic or gravity methods.
electrical resistivity - Key takeaways
- Electrical resistivity in geography measures the resistance to electric current flow through geological materials, expressed in ohm-meters (Ω·m).
- High resistivity indicates insulating materials, while low resistivity denotes conductive materials, useful in environmental studies and resource exploration.
- Techniques like Electrical Resistivity Tomography (ERT) create 3D models of geological formations to aid in groundwater studies and environmental management.
- Applications in geography include mapping underground formations, identifying water tables, and detecting mineral deposits using resistivity measurements.
- Resistivity measurements detect subsurface characteristics in geophysical surveys; low resistivity may indicate ore bodies or aquifers.
- Methods like Direct Current (DC) Resistivity and Induced Polarization (IP) are used to measure resistivity, aiding in detailed geological exploration and environmental assessments.
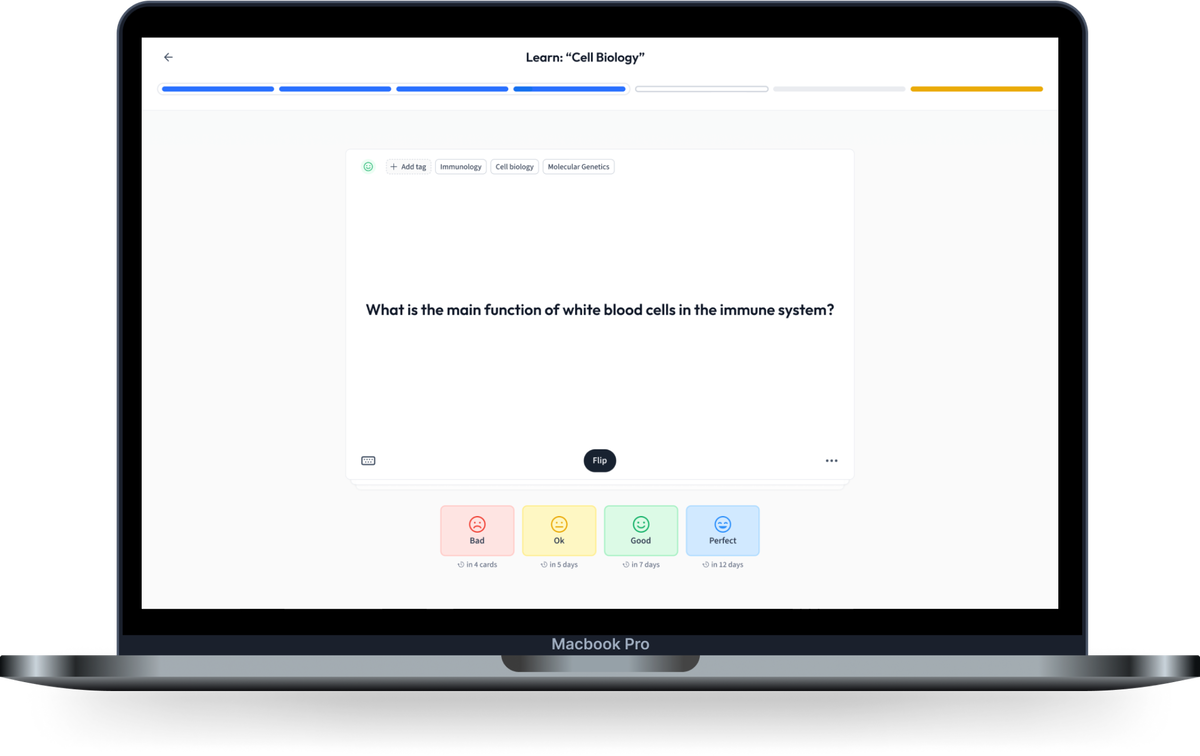
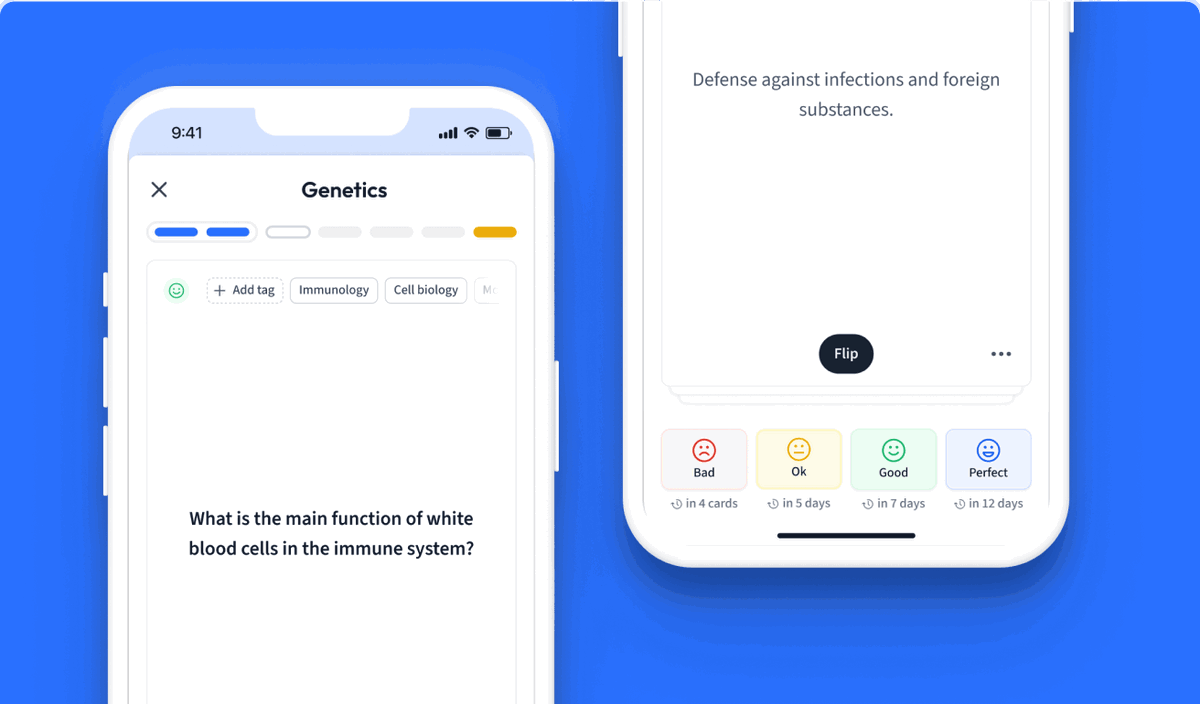
Learn with 12 electrical resistivity flashcards in the free StudySmarter app
Already have an account? Log in
Frequently Asked Questions about electrical resistivity
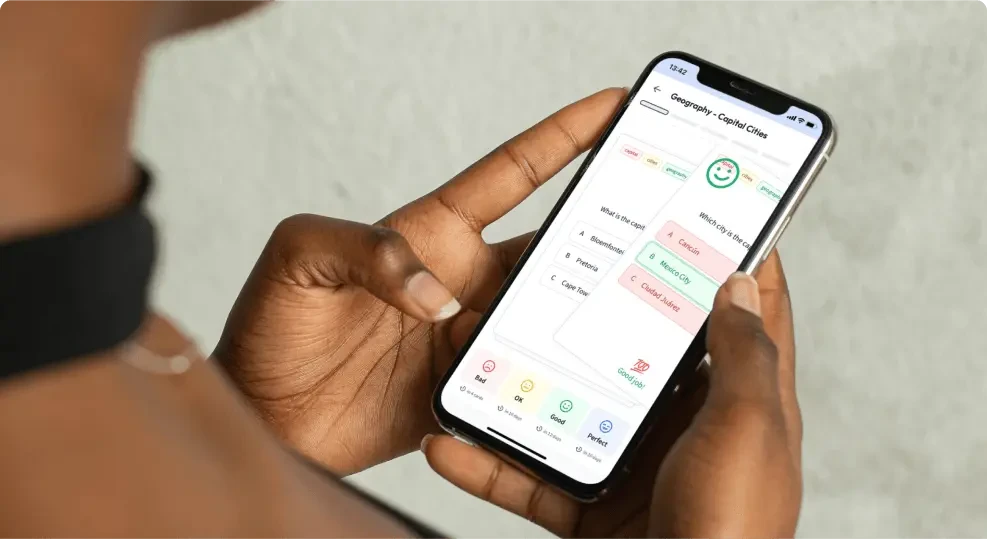
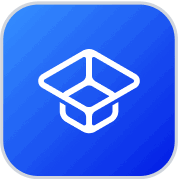
About StudySmarter
StudySmarter is a globally recognized educational technology company, offering a holistic learning platform designed for students of all ages and educational levels. Our platform provides learning support for a wide range of subjects, including STEM, Social Sciences, and Languages and also helps students to successfully master various tests and exams worldwide, such as GCSE, A Level, SAT, ACT, Abitur, and more. We offer an extensive library of learning materials, including interactive flashcards, comprehensive textbook solutions, and detailed explanations. The cutting-edge technology and tools we provide help students create their own learning materials. StudySmarter’s content is not only expert-verified but also regularly updated to ensure accuracy and relevance.
Learn more