Jump to a key chapter
Lithosphere Rheology Definition
Understanding lithosphere rheology is a fundamental part of grasping how the Earth’s surface behaves under different conditions. It involves the study of how the Earth's lithosphere, the rigid outer layer, deforms and flows. This concept helps you comprehend many natural phenomena, such as earthquakes and mountain formation.
What is Lithosphere Rheology?
The term rheology refers to the study of the flow and deformation of matter. When applied to the lithosphere, rheology focuses on how this rigid layer of the Earth deforms in response to various forces. The lithosphere consists of the crust and the uppermost mantle. It behaves like a solid on short timescales and a viscous fluid over geological timescales, making its study complex but fascinating. Factors that affect lithosphere rheology include:
- Temperature: Higher temperatures increase ductility, making the lithosphere more prone to deformation.
- Pressure: Increased pressure at greater depths affects the ductile behavior of rocks.
- Composition: The mineral content of rocks influences their mechanical properties.
- Strain rate: The speed at which rocks are deformed can determine whether they fracture or bend.
Lithosphere Rheology: The study of how the Earth's lithosphere deforms and flows under stress, particularly focusing on its behavior over different timescales and conditions.
Consider a chocolate bar left in the sun. Initially, it remains solid. However, over time and under heat, it starts to deform and eventually melts. Similarly, the lithosphere can bend, crack, or flow depending on temperature and pressure conditions, though over geological timescales.
The lithosphere is not homogeneous. Different types of rocks within it can have varying rheological properties, contributing to the complexity of its behavior.
Importance of Studying Lithosphere Rheology
Understanding lithosphere rheology allows you to predict geological events and understand Earth's dynamic processes. This knowledge is essential for a variety of reasons:
- It helps in understanding the mechanics of tectonic plate movements, which are crucial for explaining earthquakes, volcanic activity, and mountain building.
- Predicting potential geological hazards becomes more accurate, aiding in disaster preparedness and mitigation.
- It informs the extraction of natural resources such as oil, gas, and minerals, as knowledge of subsurface deformation can guide drilling efforts.
Rheological models of the lithosphere vary greatly. Common models include the Maxwell model, which considers the lithosphere as a combination of elastic and viscous components, and the Burgers model, which includes an additional Kelvin element to better simulate the complex viscoelastic behavior observed in real-world scenarios. These models are crucial for simulating and predicting the behavior of the lithosphere under different stress conditions, providing insights into how these models can be applied to understand past geological events and forecast future changes.
Lithosphere Rheology Explained
Delving into lithosphere rheology is crucial for understanding how the Earth's outer layer behaves under various forces. This encompasses studying how the Earth's lithosphere, consisting of the crust and upper mantle, deforms and flows over time. It's key to understanding natural occurrences like earthquakes and mountain building and how these processes affect the Earth's surface.
Lithosphere Rheology Fundamentals
The concept of rheology revolves around the study of the flow and deformation of materials. When associated with the lithosphere, it addresses how this rigid layer deforms under different forces over varying timescales. Factors influencing lithosphere rheology include temperature, pressure, the mineral composition of rocks, and strain rate. For example, an increase in temperature tends to make the lithosphere more ductile and susceptible to deformation.
Factors | Effects on Lithosphere |
Temperature | Higher temperatures increase ductility. |
Pressure | Influences the depth-related ductile behavior. |
Composition | Mineral content affects mechanical properties. |
Imagine bending a plastic ruler. If you bend it slowly, it flexes without breaking, illustrating ductile behavior. However, bend it quickly and it snaps, demonstrating brittle failure. The lithosphere behaves similarly under varying strain rates, deforming slowly like the ruler bending until its limits are exceeded.
The thickness of the lithosphere can vary greatly, influencing how it responds to tectonic forces. Recognizing these variations is essential for advanced rheological modeling.
Application of Lithosphere Rheology
Studying lithosphere rheology is paramount in estimating and analyzing geological phenomena. This understanding plays a vital role in various domains:
- Tectonic Plate Movements: It aids in explaining and predicting seismic activities, volcanic eruptions, and orogenesis (mountain forming) events.
- Geological Hazard Prediction: Effective disaster preparation requires accurate predictions of earthquakes and rock deformations.
- Resource Extraction: Knowledge of deformation guides natural resource extraction methods such as mining or drilling.
Advanced rheological studies involve analyzing the viscoelastic behavior of rocks under different conditions to better understand geological transformations. Cutting-edge research often utilizes computational simulations based on historical data to predict movements, aiding in the identification of strain accumulation zones. These studies not only reaffirm historical data but also forecast future geologic transformations, reflecting the lithosphere's response to continuous stresses applied over time. Such advancements contribute to economic and safety improvements in industries relying heavily on these geological insights.
Rheology Stress Strain of Lithosphere
The behavior of the lithosphere under various forces can be understood by examining its rheology, especially focusing on concepts like stress and strain. This involves understanding how rocks within the lithosphere respond to internal and external forces, leading to deformation and other geological phenomena.
Stress: A force applied over an area within the lithosphere that influences its deformation. Mathematically, stress is defined as \( \sigma = \frac{F}{A} \), where \( F \) represents the force applied, and \( A \) denotes the area.
In the context of the lithosphere, stress can be categorized into different types:
- Compressive Stress: Results from forces pushing rocks together, leading to shortening and thickening.
- Tensile Stress: Arises from forces pulling rocks apart, causing lengthening and thinning.
- Shear Stress: Occurs when forces are applied parallel to a surface, leading to distortion and slippage.
Strain: The extent of deformation experienced by the lithosphere in response to applied stress. Strain is typically calculated as \( \varepsilon = \frac{\Delta L}{L_0} \), where \( \Delta L \) is the change in length and \( L_0 \) is the original length.
Imagine stretching a rubber band. The amount it stretches is a measure of its strain in response to the applied force, or stress. Similarly, the lithosphere can undergo elastic deformation where it returns to its original shape after the stress is removed, or plastic deformation where permanent changes occur.
The relationship between stress and strain in the lithosphere can be described through various models, such as Hooke's Law for elastic deformation, given by:\( \sigma = E \varepsilon \)where \( E \) represents the Young’s Modulus, a measure of the lithosphere's ability to withstand deformation. When the stress exceeds the elastic limit, the lithosphere may undergo permanent deformation, including plastic deformation or fracturing.
The response of the lithosphere to stress and strain is not instantaneous and can vary depending on its compositional make-up and temperature conditions.
Understanding these mechanisms is crucial for predicting geological events and for applications in construction and resource extraction. Engineers, for example, need to know how much weight a geological formation can bear without failure, which is critical in designing safe structures.
Beyond traditional stress-strain models, more complex representations include the Mohr-Coulomb failure criterion, commonly used in geology to predict failure conditions in rocks. This criterion is expressed as:\( \tau = \sigma_n \tan(\phi) + c \)where \( \tau \) is the shear stress at failure, \( \sigma_n \) is the normal stress, \( \phi \) is the angle of internal friction, and \( c \) denotes cohesion. This equation helps in understanding conditions under which rocks within the lithosphere will fracture, providing invaluable insight into earthquake mechanics and resource exploration. The criterion accounts for the fact that increased normal stress can result in higher shear strength, delaying fracture.
Lithosphere Deformation Processes
The deformation processes of the lithosphere involve understanding how the Earth's rigid outer layer changes shape under various physical influences. These processes are critical to explaining the movement of tectonic plates, the formation of geological structures, and various natural phenomena, including earthquakes and volcanic activity.
Lithosphere Asthenosphere Rheology
The interaction between the lithosphere and the underlying asthenosphere is vital for understanding the Earth's dynamic nature. The asthenosphere, characterized by its relatively lower viscosity and higher ductility compared to the lithosphere, provides a semi-fluid layer over which tectonic plates move. This differential behavior can be attributed to temperature and pressure differences within these layers.
Asthenosphere: The layer of the Earth located just beneath the lithosphere, composed of semi-molten rock that is ductile and allows tectonic plates to move.
Picture a frozen cracker (lithosphere) floating on a thick syrup (asthenosphere). While the cracker is rigid and can break or bend, the syrup flows beneath it, allowing slow movement and changes.
The rheological properties of the lithosphere and asthenosphere influence tectonic processes such as:
- Subduction: The downward movement of an oceanic plate beneath a continental plate, heavily influenced by rheology.
- Rifting: The process of lithosphere extension and thinning, often initiating volcanic activity.
Geologists utilize rheological models to simulate how stress and temperature may affect the lithosphere and asthenosphere interactions over geological time.
Factors Influencing Rheology Lithosphere
Several factors influence the rheology of the lithosphere and determine its response to geological forces. These factors include:
- Temperature: A higher temperature reduces rock strength and enhances ductility, influencing deformation patterns.
- Pressure: Occurring with depth, pressure affects the compaction and ductile flow of rocks.
- Composition: Varying mineral compositions result in diverse mechanical properties within the lithosphere.
- Strain Rate: A rapid application of stress may lead to brittle failure, while a slower application allows ductile deformation.
Consider two chocolate bars: one in a cold environment and the other under the sun. The one exposed to warmth becomes malleable, demonstrating the effect of temperature on deformation, similar to how thermal conditions influence the lithosphere.
Beyond simple parameters, advanced rheological studies consider the role of surface forces and fluid interactions in the Earth's interior dynamics. Scientists employ state-of-the-art simulation technologies incorporating real-time data to model lithospheric deformation, with applications in earthquake prediction and resource exploration.
Applications of Lithosphere Rheology in Geology
Understanding the rheological characteristics of the lithosphere is crucial in various geological applications and contributes to safety, resource management, and environmental conservation. Key applications include:
- Seismic Risk Assessment: Predicting areas susceptible to earthquakes.
- Resource Exploration: Guiding the extraction of oil, gas, and minerals.
- Geotechnical Engineering: Designing infrastructure that withstands lithospheric stresses.
Innovations in rheology help improve the precision of simulation models, leading to enhanced possibilities in predicting geological phenomena.
Case Studies on Lithosphere Rheology
Analyzing real-world case studies provides insight into the practical applications of lithosphere rheology. These studies often focus on regions with significant geological activity and offer a better understanding of lithospheric processes.Notable case studies encompass:
- The San Andreas Fault: Detailed analysis of its rheology helps predict seismic activity and design earthquake-resistant structures.
- Mid-Atlantic Ridge Rifts: Where rheological models assist in understanding sea-floor spreading and volcanic activity.
- The Himalayan Orogeny: Investigating how lithospheric deformation influences mountain building and regional tectonics.
Case studies often reveal new insights into the interplay between lithosphere rheology and tectonic activities. Utilizing high-resolution data from satellite observations, such as GPS and InSAR, geologists are more equipped to study the minute movements and deformations of the lithosphere across different environmental conditions. This integration of technology elevates our understanding of lithosphere dynamics, enabling more accurate predictions of geological events like earthquakes and volcanic eruptions.
lithosphere rheology - Key takeaways
- Lithosphere rheology involves understanding how the Earth's outer layer deforms under various conditions and forces.
- Rheology of the lithosphere examines its behavior over short and geological timescales, considering factors like temperature, pressure, composition, and strain rate.
- The lithosphere is composed of the crust and upper mantle, behaves elastically over short periods, and as a viscous fluid over long periods.
- Models like the Maxwell and Burgers are used to simulate the lithosphere's complex viscoelastic behavior under different stress conditions.
- Lithosphere deformation processes and stress-strain relationships are crucial for predicting geological events and informing resource extraction.
- Understanding lithosphere rheology aids in exploring tectonic processes and interactions between the lithosphere and asthenosphere, including subduction and rifting.
Learn faster with the 12 flashcards about lithosphere rheology
Sign up for free to gain access to all our flashcards.
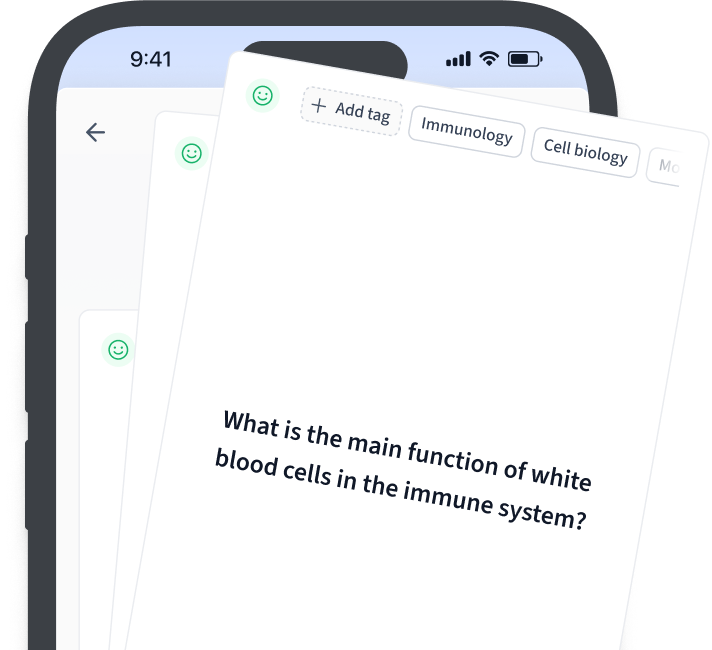
Frequently Asked Questions about lithosphere rheology
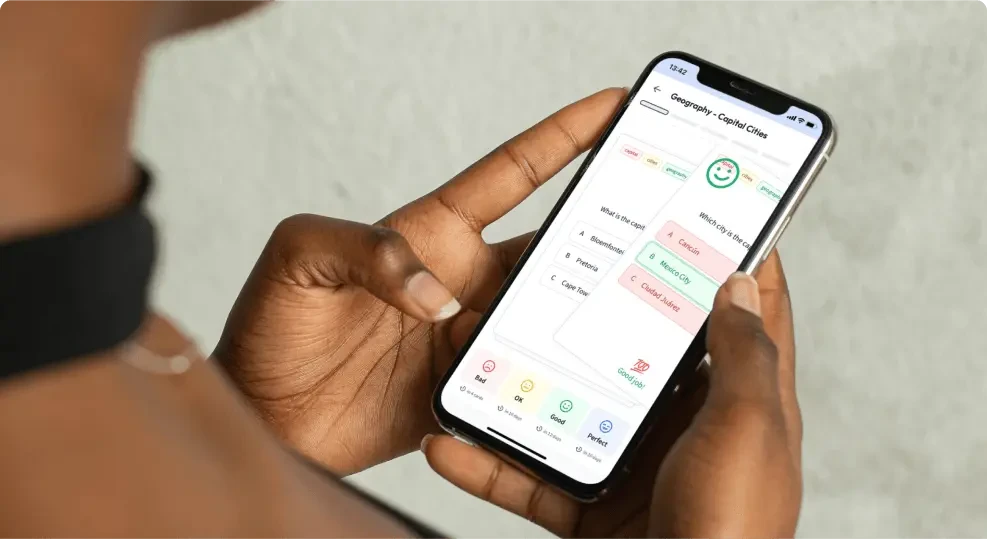
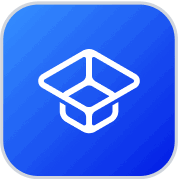
About StudySmarter
StudySmarter is a globally recognized educational technology company, offering a holistic learning platform designed for students of all ages and educational levels. Our platform provides learning support for a wide range of subjects, including STEM, Social Sciences, and Languages and also helps students to successfully master various tests and exams worldwide, such as GCSE, A Level, SAT, ACT, Abitur, and more. We offer an extensive library of learning materials, including interactive flashcards, comprehensive textbook solutions, and detailed explanations. The cutting-edge technology and tools we provide help students create their own learning materials. StudySmarter’s content is not only expert-verified but also regularly updated to ensure accuracy and relevance.
Learn more