Jump to a key chapter
Definition of Mineral Electrical Properties
Mineral electrical properties refer to how minerals conduct electricity. These properties are influenced by the composition, structure, and presence of certain elements within the mineral. Understanding these properties is important in many scientific and industrial applications.
Overview of Mineral Electrical Properties
Electrical properties of minerals include conductivity, resistivity, and permittivity. These parameters help determine how effectively a mineral can transport electric charge. Let's delve into the primary concepts that govern these properties:
- Conductivity is a measure of a material's ability to allow the flow of electric current through it. It is typically measured in siemens per meter (S/m). The general formula for conductivity is \[\sigma = \frac{1}{\rho}\] where \(\sigma\) is conductivity and \(\rho\) is resistivity.
- Resistivity is the inverse of conductivity and represents how much a mineral opposes the flow of electric current. The general formula for resistivity is \[\rho = R \times \frac{A}{L}\] where \(R\) is resistance, \(A\) is the cross-sectional area, and \(L\) is the length of the material.
- Permittivity refers to a mineral's ability to store electrical energy in an electric field. It is crucial in understanding how minerals interact with electromagnetic fields.
Did you know? Pyrite, often known as fool's gold, can conduct electricity due to its metallic characteristic!
Importance of Studying Mineral Electrical Properties
Studying the electrical properties of minerals is crucial because it helps in a wide range of areas including energy exploration, electronics, and ecological assessments. Some of the significant reasons include:
- In geophysics, understanding electrical properties aids in detecting underground mineral resources such as oil, gas, and metals.
- The electronics industry relies on minerals for components like semiconductors, where knowledge of conductivity is essential for material selection.
- In environmental science, monitoring the resistivity changes in groundwater when assessing pollution levels is crucial.
Application | Importance |
Geophysics | Resource exploration |
Electronics | Material selection |
Environmental Science | Pollution assessment |
Electrical Properties of Minerals and Rocks
Understanding the electrical properties of minerals and rocks is essential in various scientific fields. These properties determine how minerals and rocks respond to electric fields and conduct electricity, impacting areas from resource exploration to environmental monitoring.
Comparison: Electrical Properties of Rocks and Minerals
When comparing the electrical properties of rocks and minerals, it's vital to consider different factors that affect their behavior. These factors include:
- Composition: The mineralogical composition of rocks influences their electrical properties. For example, rocks high in quartz are typically less conductive compared to those rich in metallic minerals like pyrite.
- Structure: The arrangement of atoms and the presence of defects in the crystal lattice can affect conductivity. Crystalline materials generally have higher conductivity than amorphous ones.
While rocks are generally poor conductors due to their composite nature, certain minerals within them stand out for conducting electricity. Some minerals, like galena and graphite, are exceptions. Graphite, for instance, exhibits significant conductivity due to its layered structure, enabling electrons to move freely between layers. Understanding these exceptions helps in practical applications such as designing electronic components and superconductors.
A classic example of mineral conductivity is copper. Known for its excellent conductivity, copper is used extensively in electrical wiring. The formula for calculating the resistance \(R\) of a copper wire given its resistivity \(\rho\), length \(L\), and cross-sectional area \(A\) is:\[R = \rho \times \frac{L}{A}\]This formula helps engineers determine how effectively copper wires transport electricity.
Rock salt, despite being used for de-icing roads, is a poor conductor unless dissolved in water, where it then conducts electricity due to the movement of ions.
Variations in Electrical Conductivity
The electrical conductivity of minerals varies widely based on intrinsic and extrinsic factors. Key factors include:
- Temperature: Conductivity generally increases with temperature, as thermal energy facilitates charge carrier movement.
- Impurities: Even trace amounts of impurities can alter conductivity. For example, impurities in semiconductors like silicon enhance their electrical properties.
- Pressure: Increased pressure may alter the structural bonding within minerals, potentially enhancing or diminishing conductivity.
Mineral electrical properties refer to the attributes dictating a mineral's ability to conduct and resist electricity, which include conductivity, resistivity, and permittivity.
Techniques for Studying Mineral Electrical Properties
Studying the electrical properties of minerals is vital for numerous applications in science and industry. Different techniques are used to explore these properties, each offering unique insights and data. The investigation is often divided into laboratory and field-based methods, each with its own set of practices and tools.
Laboratory Techniques
Laboratory techniques provide a controlled environment to study the electrical properties of minerals. These techniques often involve sophisticated equipment to ensure accurate measurements.Common methods include:
- DC and AC conductivity tests: These involve passing a current through the mineral sample and measuring its response. Conductivity can be calculated using the formula:\[ \sigma = \frac{L}{R \cdot A} \]where \(\sigma\) is conductivity, \(R\) is resistance, \(A\) is the cross-sectional area, and \(L\) is the length of the sample.
- Dielectric spectroscopy: This technique measures the permittivity of a mineral by applying an alternating electric field and analyzing its response. It's useful for understanding how minerals interact with electromagnetic waves.
- Electrochemical impedance spectroscopy (EIS): EIS enables the study of complex impedance, helping researchers determine the resistive and reactive properties of minerals.
Consider a scenario where you use dielectric spectroscopy to study limestone's permittivity. By applying varying frequencies, you can observe changes and extract valuable information about its structure and mineral constituents.
For better accuracy in DC conductivity tests, ensure that your mineral samples are dry and devoid of surface contaminants, as they can significantly affect the outcome.
Field Methods
Field methods complement laboratory techniques by allowing the study of minerals amidst natural geological settings. They are vital for real-world application and exploration.Common field methods include:
- Electrical Resistivity Tomography (ERT): This technique measures the difference in electrical resistivity across a field. By inserting electrodes into the ground and passing current, the resistivity of various minerals can be mapped.
- Induced Polarization (IP): An extension of ERT, IP observes the delay in voltage decay, providing additional data on mineral properties such as metallic content.
- Ground Penetrating Radar (GPR): Although not directly measuring electrical properties, GPR helps map sub-surface structures by using electromagnetic signals, offering indirect insights.
Method | Application |
ERT | Mapping resistivity in subsurface |
IP | Detecting chargeable materials like ores |
GPR | Understanding ground structures |
In the context of field methods, variations in resistivity can indicate the presence of groundwater, mineral deposits, or even voids within the earth. This makes techniques like ERT invaluable for resource exploration and environmental assessments. Furthermore, advanced processing of IP data can help differentiate between clay and metallic mineralization, which is crucial for mining operations.
Factors Affecting Mineral Electrical Properties
Understanding the factors influencing mineral electrical properties is critical in fields like geology, electronics, and environmental science. Factors such as mineral composition, structural characteristics, temperature, and pressure significantly impact a mineral's ability to conduct electricity.
Mineral Composition and Structure
The composition and structure of minerals play a pivotal role in determining their electrical properties. Here's how these aspects contribute:
- Elemental Composition: Minerals containing metallic elements like copper and silver tend to have higher conductivity due to the presence of free electrons.
- Crystal Structure: The arrangement of atoms in a crystal lattice affects how electrons move. A highly ordered structure can either facilitate or hinder electron flow, depending on the presence of pathways or defects.
- Ionic Composition: Minerals with ionic bonds can conduct electricity when ions are free to move, typically seen in molten or dissolved states.
Quartz is an example of a mineral with poor conductivity due to its strong covalent bonds and lack of free electrons. Conversely, graphite, despite being composed purely of carbon like diamond, has a layered structure that allows free electron movement, resulting in higher conductivity.
Layered structures, as found in graphite, often allow better conductivity due to increased surface area for electron movement.
Temperature and Pressure Influence
The effects of temperature and pressure on mineral electrical properties are profound. As temperature increases, the electrical conductivity of most minerals also increases due to the enhanced movement of electrons or ions. Pressure changes can alter the atomic structure, influencing conductivity.Here's a more detailed look:
- Temperature: With rising temperatures, the energy provided enables charge carriers to overcome potential barriers more easily, thereby enhancing conductivity. This can be expressed mathematically as:\[\sigma(T) = \sigma_0e^{-\frac{E_a}{kT}}\]where \(\sigma(T)\) is the temperature-dependent conductivity, \(\sigma_0\) is the pre-exponential factor, \(E_a\) is the activation energy, \(k\) is Boltzmann's constant, and \(T\) is the temperature in Kelvin.
- Pressure: Higher pressure may lead to denser atomic packing, impacting the pathways available for electron or ion movement. This can either enhance or hamper conductivity depending on mineral-specific characteristics.
Temperature and pressure not only influence conductivity but also affect the phase transitions of minerals. For instance, perovskite, a mineral closely studied for its conductive properties, undergoes changes in its conductive behavior under different temperature and pressure conditions. The study of these transitions provides insight into Earth's interior processes and potential technological applications such as superconductors.
Examples of Mineral Electrical Properties
The study of mineral electrical properties reveals a lot about their conductivity and utility in various applications. By examining common minerals and their ability to conduct electricity, you can gain insight into their roles in geological and industrial contexts.
Common Mineral Examples and Their Conductivity
Different minerals exhibit a wide range of conductivity. Some are excellent conductors, while others are highly resistive. Here’s a closer look at some common examples:
- Graphite: Known for its high conductivity due to its structure. The layers within graphite allow electrons to move freely. The conductivity of graphite can be expressed as:\[\sigma = n \cdot e \cdot \mu\]where \(n\) is charge carrier density, \(e\) is the charge of an electron, and \(\mu\) is electron mobility.
- Pyrite: Often mistaken for gold, pyrite can conduct electricity owing to its metallic sulfide content. This conductivity is crucial in processes such as flotation in mining.
- Quartz: A poor conductor due to its strong covalent bonds and lack of free electrons, making it valuable in creating insulators.
Mineral | Type of Conductivity |
Graphite | High |
Pyrite | Moderate |
Quartz | Low |
Consider copper, widely known for its exceptional conductivity. This property makes it ideal for electrical wiring. The conductivity of copper is often used as a standard to compare against other materials, underpinning industries from telecommunications to power distribution.
Diamonds, like quartz, have low electrical conductivity due to the strong covalent bonding between carbon atoms, making them excellent insulators.
Special Cases in Electrical Properties of Minerals
In addition to commonly known conductive minerals, some minerals exhibit unique electrical properties under specific conditions. These special cases can include:
- Semiconductors: Certain minerals, like galena (lead sulfide), exhibit semiconductor properties. These are used in electronics for their ability to conduct electricity under specific conditions, with conductivity modeled by:\[\sigma = \sigma_0 e^{-\frac{E_g}{kT}}\]where \(\sigma_0\) is the conductivity at 0 K, \(E_g\) is the band gap energy, \(k\) is Boltzmann's constant, and \(T\) is temperature.
- Piezoelectric Minerals: Minerals such as tourmaline create an electric charge in response to mechanical stress. This property finds applications in sensors and actuators.
- Superconductors: While not found naturally, certain minerals become superconducting at low temperatures, allowing resistance-free electron flow.
Superconducting minerals, although rare, offer significant insights into the future of energy technology. Materials like cuprates exhibit superconductivity at relatively high temperatures compared to traditional superconductors, which opens the door to practical applications in maglev trains and powerful electromagnets used in MRI machines. Superconductivity is a state where electrical resistance drops to zero, allowing limitless current flow, described through critical temperature \(T_c\) and critical field \(H_c\):\[R = 0, \, \text{for} \, T < T_c \, \text{and} \, H < H_c\]
Though superconductors offer no resistance, they require very low temperatures to maintain the superconducting state, often making them expensive to utilize currently.
mineral electrical properties - Key takeaways
- Definition of Mineral Electrical Properties: Refers to how minerals conduct electricity, influenced by composition, structure, and elements present.
- Key Electrical Properties of Minerals: Includes conductivity, resistivity, and permittivity, which determine a mineral's capacity to transport electric charge.
- Techniques for Studying Mineral Electrical Properties: Laboratory methods (DC, AC tests, dielectric spectroscopy, EIS) and field methods (ERT, IP, GPR) provide insights into mineral behaviors.
- Factors Affecting Mineral Electrical Properties: Influence of composition, structure, temperature, and pressure on a mineral's ability to conduct electricity.
- Examples of Mineral Electrical Properties: Graphite (high conductivity), Quartz (low conductivity), and Pyrite illustrates varying conductive behaviors in minerals.
- Important Mineral Cases: Semiconductors, piezoelectric minerals, and superconductors show unique electrical properties useful in advanced technology development.
Learn faster with the 10 flashcards about mineral electrical properties
Sign up for free to gain access to all our flashcards.
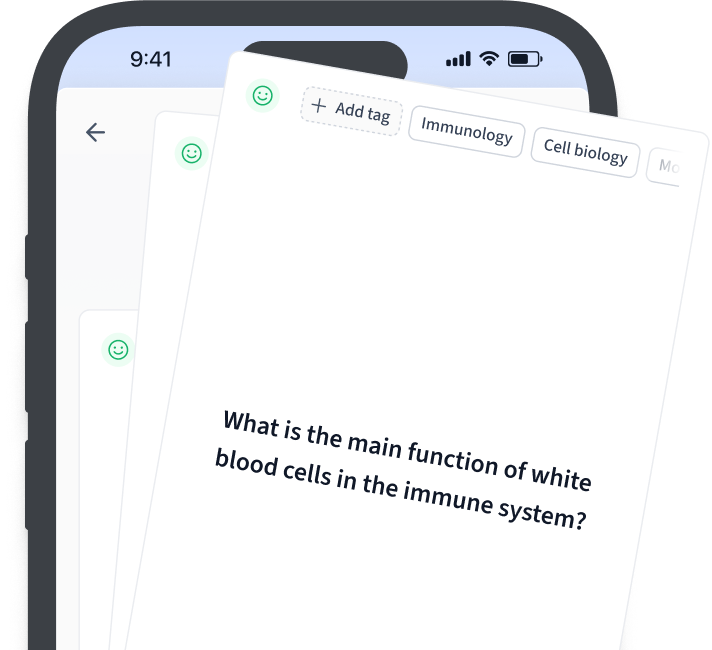
Frequently Asked Questions about mineral electrical properties
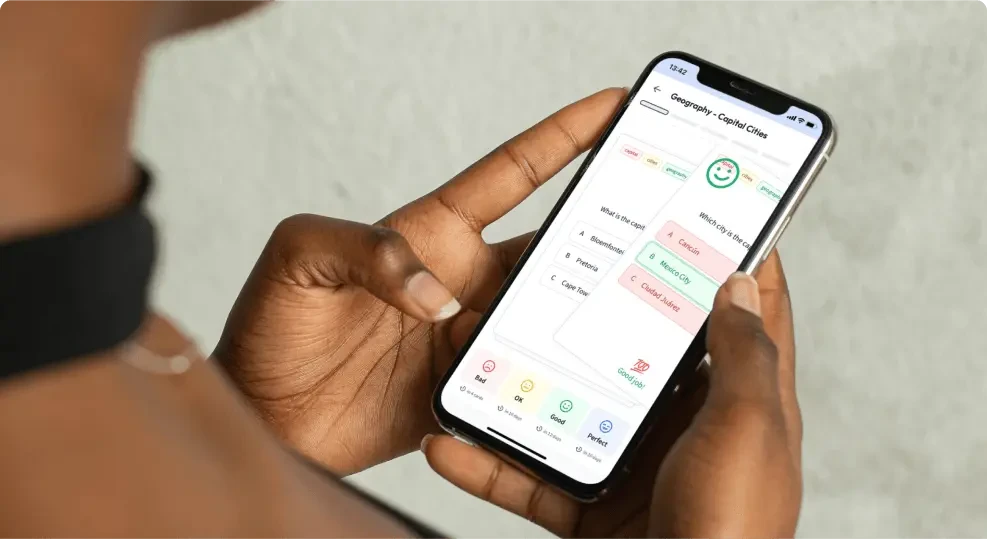
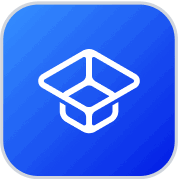
About StudySmarter
StudySmarter is a globally recognized educational technology company, offering a holistic learning platform designed for students of all ages and educational levels. Our platform provides learning support for a wide range of subjects, including STEM, Social Sciences, and Languages and also helps students to successfully master various tests and exams worldwide, such as GCSE, A Level, SAT, ACT, Abitur, and more. We offer an extensive library of learning materials, including interactive flashcards, comprehensive textbook solutions, and detailed explanations. The cutting-edge technology and tools we provide help students create their own learning materials. StudySmarter’s content is not only expert-verified but also regularly updated to ensure accuracy and relevance.
Learn more