Jump to a key chapter
Definition of Mineral Spectroscopy
Mineral Spectroscopy is a scientific technique utilized to study the optical properties of minerals. It involves the analysis of the interaction between light and minerals, allowing you to determine the mineral's composition and structural features.
Understanding the Basics of Mineral Spectroscopy
Mineral spectroscopy operates by observing how minerals absorb, reflect, or emit electromagnetic radiation. By understanding these interactions, you can gain insights into:
- The chemical composition of minerals
- Structural information about the mineral lattice
- Crystallography details
Electromagnetic Spectrum: A continuous range of electromagnetic waves including visible light, infrared, ultraviolet, and other forms. In mineral spectroscopy, the focus is primarily on the infrared and visible regions.
Example of Mineral Spectroscopy Application:Consider a mineral suspected to contain iron. By using mineral spectroscopy, you can direct light towards the sample and measure the intensity of the absorbed wavelengths. Fe-bearing minerals show distinct absorption bands, which can be identified with spectroscopy.
Mineral spectroscopy becomes particularly fascinating when examining complex mineral compositions. The method allows the differentiation between minerals that appear similar. For instance, minerals like pyroxenes and olivines may seem alike in appearance, but spectroscopy reveals that they absorb different wavelengths due to variations in their structure and composition. By using sophisticated devices, these details become evident, illustrating the incredible depth mineral spectroscopy offers.
Typically, minerals that are opaque or dark in color may absorb more light, leading to prominent absorption bands in their spectrum.
Mineral Spectroscopy Techniques
Mineral spectroscopy techniques are diverse and highly effective in exploring the physical and chemical properties of minerals. Understanding these techniques helps unravel the mysteries of the Earth's components. Various methods are used, each providing unique insights into the mineral's characteristics.
Infrared Spectroscopy
Infrared spectroscopy is a commonly used technique in mineral spectroscopy. This method involves measuring the infrared light absorbed by a mineral, which results in a spectrum that provides vital information about its molecular vibrations. The key advantages include:
- Ability to identify mineral structures
- Detection of water and hydroxyl groups
- Non-destructive analysis
Example of Infrared Spectroscopy:Imagine analyzing a sample of clay mineral. Its infrared spectrum reveals peaks corresponding to the Si-O and Al-O vibrations, indicating its structural components.
Raman Spectroscopy
Raman spectroscopy is another invaluable method used in mineral spectroscopy. This technique involves scattering light off the mineral and measuring the inelastic scattering to gain insights into vibrational modes. It's highly useful for:
- Identifying mineral composition
- Structural analysis
- Characterizing crystal structures
Raman spectroscopy is particularly effective in analyzing carbon-related minerals like graphite and diamond, due to their unique Raman shifts.
X-ray Diffraction (XRD) Spectroscopy
X-ray diffraction (XRD) spectroscopy is used to determine the crystallographic structure of minerals. By directing X-rays at a mineral and measuring the diffraction pattern, XRD provides powerful insights into:
- Lattice structure
- Phase identification
- Crystallite size
Beyond basic mineral identification, X-ray diffraction allows for the detection of impurities in minerals due to its precise measurement capabilities. This makes it particularly valuable in the mining industry, where understanding the purity of mineral deposits is crucial. The precision of XRD helps in tailoring industry processes to maximize yield and reduce waste.
Raman Spectroscopy Minerals
Raman spectroscopy is an innovative and non-destructive technique applied to study minerals. It is based on the Raman effect, where light scatters inelastically, revealing vibrational, rotational, and other low-frequency modes in a system. This technique is incredibly versatile for mineral analysis.
Principles of Raman Spectroscopy in Mineral Analysis
When you utilize Raman spectroscopy in mineral analysis, the primary focus is on observing shifts in the wavelength of scattered light. This shift provides a 'fingerprint' by which you can identify different minerals. The Raman spectrum generated offers insights into:
- The mineral's molecular vibrations
- Bonding characteristics
- Crystal structure
Raman Effect: A change in the wavelength of light that occurs when it is deflected by molecules. This effect is crucial for understanding vibrational properties in minerals and helps identify them at a molecular level.
Example of Raman SpectroscopySuppose you are analyzing a mineral suspected to be quartz. When you apply Raman spectroscopy, specific peaks appear at 464 cm-1, indicative of the mineral's Si-O stretching vibrations, confirming the mineral as quartz.
Applications in Mineral Identification
Raman spectroscopy is highly beneficial for identifying various types of minerals, particularly in geology and environmental science. It can determine the mineral composition in:
- Rocks and soils
- Mining samples
- Archaeological artifacts
Raman spectroscopy can be performed on very small samples, even those less than 1 mm in size, without destroying the sample.
A deeper exploration into Raman spectroscopy reveals its application in detecting phase transitions in minerals. For instance, the polymorphs of titanium dioxide (TiO2) such as anatase and rutile exhibit different Raman spectra. Monitoring these transitions under varying temperature and pressure can help understand geological processes occurring beneath the Earth's crust. This capability underscores the significance of Raman spectroscopy in both academic research and industrial applications.
Infrared Spectroscopy of Minerals and Related Compounds
Infrared spectroscopy is a powerful tool in the study of minerals and related compounds. It focuses on the absorption of infrared light by minerals, which provides critical insights into their molecular vibrations and chemical bonds. Through this technique, you can identify structural composition and the presence of certain groups such as hydroxyl (OH) and carbonate (CO3).
Educational Examples of Mineral Spectroscopy
Infrared spectroscopy can be illustrated using examples that showcase its application in identifying mineral properties. Consider the mineral gypsum, which contains water molecules in its structure. When analyzed, its infrared spectrum presents distinct absorption bands attributed to water molecules and sulfate ions. These provide evidence of the mineral's identity.In another example, calcite's infrared spectrum reveals characteristic peaks associated with carbonate ions. The presence of these peaks allows you to confirm its composition without ambiguity.
Mineral | Characteristic Peak (cm-1) |
Gypsum | 3400 (OH stretch) |
Calcite | 1415 (CO3 stretch) |
Example of ApplicationWhen assessing a rock sample suspected to contain minerals like quartz, observing the infrared spectrum at 1085 cm-1 confirms the presence of Si-O-Si bond vibrations.
Infrared and Raman Spectroscopies of Clay Minerals
Clay minerals, characterized by their layered structures, are effectively studied using both infrared and Raman spectroscopy. These techniques complement each other in revealing the mineral's fine details. Infrared spectroscopy highlights hydroxyl group vibrations and interlayer water, while Raman spectroscopy offers information about their crystal lattice.Consider montmorillonite, a clay mineral. Infrared spectroscopy detects strong absorption at 3620 cm-1 due to OH stretching, and Raman spectroscopy can identify low-frequency modes attributed to the octahedral sheet vibrations. Such spectroscopic analyses provide invaluable data for characterizing clay mineral structures in environmental and geological studies.The behavior of clay minerals in Raman spectroscopy can be mathematically expressed by the equation:\[\Delta u = u_{incident} - u_{scattered}\]where \(\Delta u\) represents the shift due to scattering, \(u_{incident}\) is the frequency of the incoming light, and \(u_{scattered}\) is the frequency of the scattered light.
Clay minerals often contain water in their structure, detectable through characteristic infrared absorption bands at around 3400 cm-1.
A deeper understanding reveals that clay minerals play a crucial role in soil science and environmental engineering. Their ability to absorb water and act as a medium for chemical exchange means they are heavily analyzed using both infrared and Raman spectroscopic methods. This capability extends to the study of environmental pollution, as clay minerals can adsorb heavy metals and organic compounds, which can also be detected and quantified through these spectroscopy techniques. Understanding these interactions aids in evaluating soil health and designing remediation strategies for polluted sites.
mineral spectroscopy - Key takeaways
- Mineral Spectroscopy Definition: A scientific technique used to study the optical properties of minerals by analyzing light interaction to determine composition and structure.
- Raman Spectroscopy of Minerals: A method based on inelastic light scattering, providing insights into molecular vibrations and crystal structures.
- Infrared Spectroscopy of Minerals and Related Compounds: This technique analyzes infrared light absorption, revealing molecular vibrations and chemical bonds in minerals.
- Mineral Spectroscopy Techniques: Includes infrared spectroscopy, Raman spectroscopy, and X-ray diffraction to explore mineral characteristics.
- Educational Examples: Examples using IR and Raman spectroscopy to identify minerals like quartz, gypsum, calcite, and clay minerals, focusing on characteristic peaks.
- Infrared and Raman of Clay Minerals: These techniques provide detailed information on clay minerals' structures, beneficial for environmental and soil science.
Learn faster with the 12 flashcards about mineral spectroscopy
Sign up for free to gain access to all our flashcards.
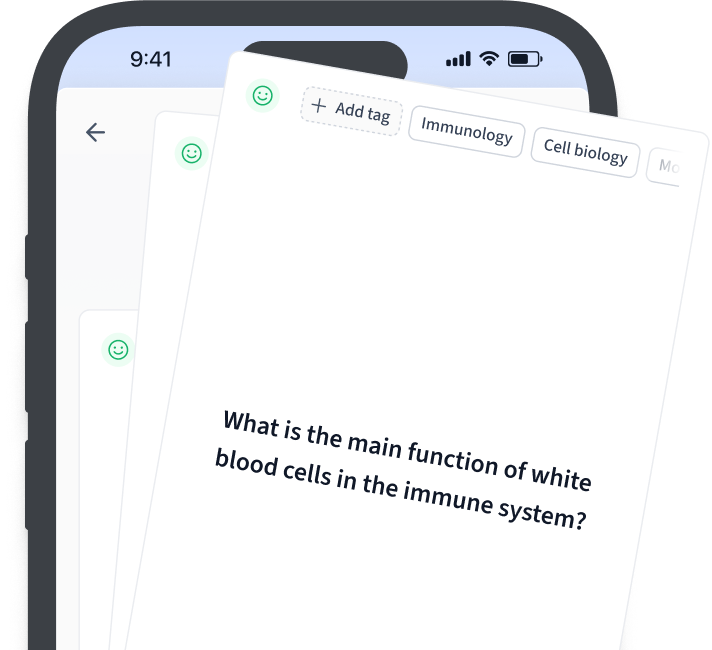
Frequently Asked Questions about mineral spectroscopy
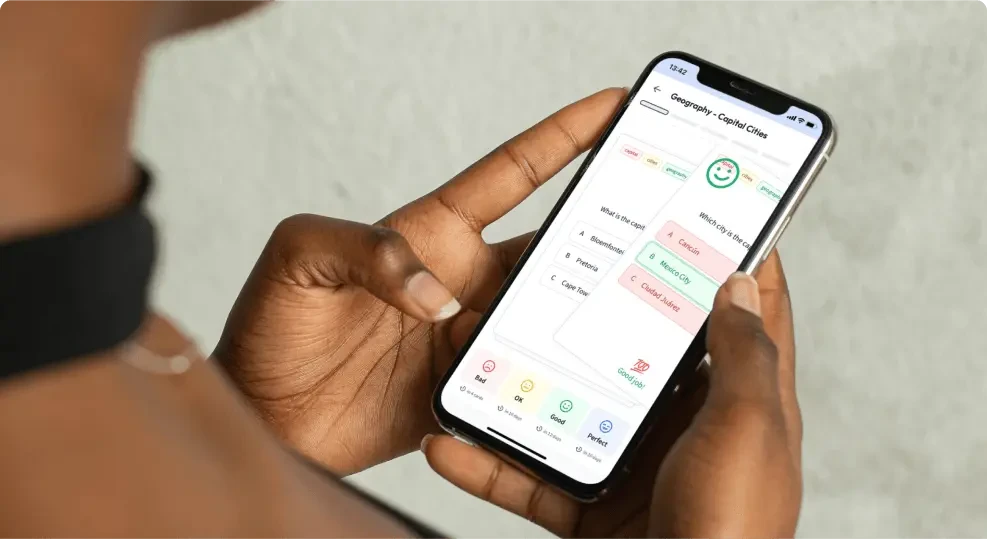
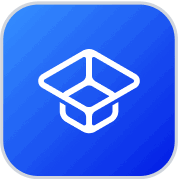
About StudySmarter
StudySmarter is a globally recognized educational technology company, offering a holistic learning platform designed for students of all ages and educational levels. Our platform provides learning support for a wide range of subjects, including STEM, Social Sciences, and Languages and also helps students to successfully master various tests and exams worldwide, such as GCSE, A Level, SAT, ACT, Abitur, and more. We offer an extensive library of learning materials, including interactive flashcards, comprehensive textbook solutions, and detailed explanations. The cutting-edge technology and tools we provide help students create their own learning materials. StudySmarter’s content is not only expert-verified but also regularly updated to ensure accuracy and relevance.
Learn more