Jump to a key chapter
What is Reflection Seismology?
Reflection seismology is a fascinating technique used in geophysical surveys to map and study subsurface structures. It has a wide range of applications, especially in the fields of oil and gas exploration, mineral exploration, and earthquake research. This method involves sending seismic waves into the Earth and analyzing the waves that are reflected back to the surface. Let's explore how it works and its various components.
How Reflection Seismology Works
The basic principle behind reflection seismology is similar to echo-sounding. When seismic waves travel through different subsurface layers, they are reflected back to the surface. These reflections are due to variations in the acoustic impedance of the layers. Acoustic impedance is a property that depends on the density and the velocity of seismic waves in the material. The seismic waves are usually generated using controlled sources such as explosives or specialized mechanical devices. Receivers, known as geophones or hydrophones, detect and record the reflected waves. This data is then processed to create an image of the subsurface, which can reveal vital information about its structure and composition.
Acoustic Impedance: The product of the density of a medium and the speed of sound within it. It plays a key role in determining the reflection and transmission of seismic waves at material boundaries.
Mathematical Representation
The reflection of seismic waves can be described using mathematical equations. A fundamental concept is the reflection coefficient, which quantifies how much of the seismic wave is reflected when it encounters a boundary between two different media. The reflection coefficient (R) is given by the equation: \[ R = \frac{Z_2 - Z_1}{Z_2 + Z_1} \] where \(Z_1\) and \(Z_2\) are the acoustic impedances of the first and second medium, respectively. This equation helps predict how much seismic energy is reflected at boundaries with different acoustic properties.
Consider two layers beneath the Earth's surface, Layer A with an acoustic impedance \(Z_A = 3500 \, \text{kg/m}^2\) and Layer B with \(Z_B = 3800 \, \text{kg/m}^2\). The reflection coefficient can be calculated as follows: \[ R = \frac{3800 - 3500}{3800 + 3500} = \frac{300}{7300} \approx 0.041 \] This positive reflection coefficient indicates that Layer B reflects a portion of the seismic wave back to the surface.
Applications of Reflection Seismology
Reflection seismology has numerous practical applications. Some of its primary uses include:
- Oil and Gas Exploration: Identifying subsurface structures to locate potential hydrocarbon reservoirs.
- Mineral Exploration: Mapping ore deposits.
- Earthquake Research: Understanding fault lines and the Earth's crustal structures.
- Engineering Geology: Assessing subsurface conditions for construction projects.
Reflection seismology is also used to study the seafloor and the entire oceanic crust, aiding in oceanographic research.
Advanced Processing Techniques
To obtain high-quality images of the subsurface, advanced data processing techniques are applied to seismic data. These techniques include:
- Stacking: Combining multiple seismic records to enhance signal quality and reduce noise.
- Migrating: Correcting the positions of reflections to accurately represent their subsurface locations.
- Deconvolution: Improving the sharpness of seismic records by minimizing distortions.
In the realm of reflection seismology, the advent of 3D seismic surveys has revolutionized the field. Unlike traditional 2D surveys, 3D surveys provide a comprehensive view of the subsurface from all angles. This is achieved by deploying a grid of geophones over a large area and capturing seismic waves in three dimensions. The 3D technique enhances the resolution and accuracy of subsurface images, allowing for the detection of smaller structures and anomalies that might be missed in 2D surveys. By using cutting-edge computational algorithms, such as inversion and finite-difference modeling, the complex data gathered from 3D surveys can be processed to predict subsurface properties with higher fidelity.This level of detail is particularly beneficial in regions with intricate geological settings, offering scientists and engineers the ability to conduct more detailed and reliable analyses for resource exploration and risk assessments.
Reflection Seismology Method
Reflection seismology is a technique used to investigate Earth's subsurface by analyzing seismic waves that are reflected back to the surface. This method is especially valuable in geoscience for mapping subsurface geometry and identifying natural resources.
Techniques in Reflection Seismology
In reflection seismology, a variety of techniques are employed to optimize data collection and interpretation:
- Controlled Source Seismology: Uses artificial sources like explosives or vibroseis trucks to generate clear seismic signals.
- Detection with Geophones: These sensors are distributed across the survey area to detect reflections.
- Seismic Recording: Captures seismic signals over time, producing data sheets known as seismograms.
Seismogram: A record produced by a seismograph, documenting seismic signals over a set period.
Imagine conducting a survey over a known sedimentary basin. Controlled vibrations are sent into the ground, and geophones record the returning reflections. The differences in wave travel times indicate changes in subsurface properties, allowing the creation of a geological map that highlights stratification and potential resource pockets.
A deeper insight into reflection seismology is found in advanced processing techniques such as Full Waveform Inversion (FWI). This method uses the entire seismic wave spectrum instead of just reflections. FWI provides a more detailed model of subsurface properties like velocity and density with greater accuracy. It involves iteratively adjusting a subsurface model until the observed data and synthetic data are in close agreement, achieved through minimization techniques.
Acoustic Impedance in Reflection Seismology
Acoustic impedance is a key concept in reflection seismology that dictates how seismic waves interact with subsurface layers. It influences both the reflection and transmission of waves at material boundaries. When seismic waves hit a boundary between two materials with different acoustic impedances, part of the wave is reflected back and part is transmitted into the next layer. The efficacy of this reflection is quantified by the reflection coefficient. This coefficient is calculated using:\[ R = \frac{Z_2 - Z_1}{Z_2 + Z_1} \]where \(Z_1\) and \(Z_2\) are the acoustic impedances of the upper and lower layers, respectively.
Consider a simple model with a sandstone layer (\(Z_1 = 2500 \, \text{kg/m}^2\)) overlaying a limestone layer (\(Z_2 = 3000 \, \text{kg/m}^2\)). The reflection coefficient is calculated as follows:\[ R = \frac{3000 - 2500}{3000 + 2500} = \frac{500}{5500} \approx 0.091 \]This result signifies that roughly 9.1% of the seismic wave's energy is reflected at this boundary.
Acoustic impedance contrast is often visualized using seismic color blips on seismograms, showing how distinct rock layers interface.
In addition to basic reflections, reflection seismology also investigates multiple reflections. These are seismic waves that bounce back and forth multiple times between subsurface layers before being recorded. The occurrences of multiple reflections can complicate the interpretation of seismic data, but they also offer additional information regarding the thickness and properties of the intervening layers. Advanced processing technologies can filter out or utilize these reflections to improve data accuracy.
Reflection Seismology Data Interpretation
In the field of reflection seismology, data interpretation is crucial to understanding the Earth's subsurface structures. By analyzing how seismic waves travel and are reflected through different geological layers, geoscientists can build detailed models of underground formations. This process is key for applications like natural resource exploration, earthquake research, and archaeological investigations.Interpreting seismic data involves several steps, including analyzing waveforms, evaluating reflection patterns, and using mathematical models. With sophisticated software, these data can be translated into comprehensible subsurface images that highlight various stratigraphic features.
Analyzing Seismic Waves
Seismic wave analysis is a fundamental aspect of reflection seismology. These waves, generated by a source, penetrate the Earth and are reflected back by subterranean layers with varying elastic properties. By examining seismic recordings, it's possible to determine:
- The velocity of seismic waves in various layers
- The thickness and dip of geological strata
- The presence of faults or anticlinal structures
Elastic Properties: Characteristics of materials that describe how they deform and return to their original shape when subjected to stress.
Consider a seismic survey over a basin composed of sedimentary rocks. The initial seismic wave travels through multiple layers, and each reflection is analyzed. Suppose the first reflection arrives in 1 second and the velocity is assumed to be 2000 m/s.Applying the formula:\[ h = \frac{v \times t}{2} = \frac{2000 \times 1}{2} = 1000 \, \text{meters} \]This indicates the depth of the first reflector is 1000 meters.
Seismic waves can be divided into several types such as P-waves and S-waves, which provide different insights into subsurface characteristics. P-waves, or primary waves, travel faster and are the first to arrive at the receivers. They move in a compressional motion, enabling them to propagate through solids, liquids, and gases. S-waves, or secondary waves, travel slower and move in a shear motion, only through solids. By examining the travel times and amplitudes of these waves, it is possible to infer additional details about subsurface conditions, such as fluid content and lithology.
Using Acoustic Impedance for Data Insights
Acoustic impedance plays a pivotal role in reflection seismology, being the parameter that affects how seismic waves are reflected at boundaries between different geological layers.When seismic waves encounter a boundary with contrasting acoustic impedances, the amplitude of the reflected wave is determined by the reflection coefficient. The equation for reflection coefficient \(R\) is:\[ R = \frac{Z_2 - Z_1}{Z_2 + Z_1} \]Acoustic impedance \(Z\) is the product of rock density \(\rho\) and wave velocity \(v\):\[ Z = \rho \times v \]These equations are critical in determining the reflection interface properties and their impact on interpretation.
A subsurface investigation reveals a shale layer with an acoustic impedance of 4000 \(\text{kg}/\text{m}^2\) and underlying sandstone with 5000 \(\text{kg}/\text{m}^2\). The reflection coefficient is calculated as:\[ R = \frac{5000 - 4000}{5000 + 4000} = \frac{1000}{9000} \approx 0.111 \]The result indicates a clear reflection, useful for delineating subsurface features.
Higher reflection coefficients often correspond to more distinct seismic reflections, signaling significant contrasts between layer properties.
In recent years, advancements in seismic inversion techniques have allowed geoscientists to better extract acoustic impedance from seismic data, offering detailed models of the subsurface. Seismic inversion transforms seismic reflection data into a quantitative rock-property description, enabling a detailed interpretation of reservoir characteristics. By using variations in impedance, identifiable through seismic signature, it is possible to infer the lithological properties and fluid contents of the subsurface layers, greatly enhancing the accuracy of geological forecasts.
Difference Between Reflection and Refraction Seismology
Understanding the techniques of reflection seismology and refraction seismology is crucial for interpreting subsurface structures. Both methods are integral in geophysical surveys but operate based on different principles. Reflection seismology focuses on the energy waves that are reflected back to the surface, while refraction seismology studies the bending of waves as they pass through different layers.
Refraction Seismology: A technique where seismic waves are analyzed based on how they bend or refract when passing through subsurface layers, primarily used to study deeper geological structures.
Consider a seismic study where the goal is to map a fault beneath the surface. Reflection seismology might reveal the horizontal layers and their discontinuities, indicating the presence of a fault. Alternatively, refraction seismology could help determine the fault's depth by analyzing how seismic waves bend and travel through the layers.
Key Characteristics and Applications
Both reflection and refraction seismology have unique characteristics and applications in the study of Earth’s subsurface.
- Reflection Seismology:
- Used for detailed analysis of shallow to medium-depth structures.
- Highly effective in hydrocarbon exploration and sedimentary layer mapping.
- Relies on the reflection coefficient calculated from acoustic impedance contrasts.
- Refraction Seismology:
- Ideal for analyzing deeper structures, up to several kilometers.
- Commonly applied in earthquake studies and large-scale geological investigations.
- Utilizes the bending behavior of seismic waves to infer subsurface characteristics.
Method | Characteristics | Applications |
Reflection Seismology | Analyzes reflections, uses acoustic impedance | Oil exploration, shallow surveys |
Refraction Seismology | Analyzes bending/refracting waves | Deep studies, earthquake research |
Reflection seismology is more commonly used in oil exploration due to its ability to locate and map potential oil reservoirs with high precision.
Comparing Data Response and Interpretation
Data response and interpretation vary significantly between reflection and refraction seismology. In reflection seismology, data interpretation involves analyzing the amplitudes and time-delays of reflected signals to determine layer composition and depth. The interpretation often results in seismic images illustrating horizontal layers.In contrast, refraction seismology focuses on interpreting the bending path and arrival times of seismic waves. This can reveal information about the velocity structures of deeper layers. The interpretation produces velocity profiles rather than direct images of the subsurface.
Both methods employ specific mathematical models to assist with data interpretation. In reflection seismology, the formula for calculating travel time for waves reflected from a horizontal layer is:\[ t = \frac{2h}{v} \]where \(t\) is travel time, \(h\) is layer depth, and \(v\) is wave velocity.For refraction seismology, the calculation involves critical angles and Snell's Law. Snell's Law is instrumental in describing how waves change direction at boundaries:\[ \frac{\sin \theta_1}{v_1} = \frac{\sin \theta_2}{v_2} \]where \(\theta_1\) and \(\theta_2\) are the angles of incidence and refraction, and \(v_1\) and \(v_2\) are the wave velocities in different media. By applying these formulas and analyzing wave paths, better interpretations of the subsurface are achieved.
Examples of Reflection Seismology Applications
Reflection seismology is a powerful technique with various applications, from natural resource exploration to academic research into Earth's subsurface structures.
Oil and Gas Exploration
In the oil and gas industry, reflection seismology is an essential tool used to identify and locate potential hydrocarbon reservoirs. By sending seismic waves into the ground and analyzing the reflected signals, geophysicists can map subsurface formations and pinpoint structures like anticlines and faults which may trap hydrocarbons.The estimate of oil reserves is enhanced by examining the acoustic impedance contrasts of rocks - the difference often reveals the presence of oil or gas. As waves reflect off boundaries between layers, their travel times and amplitudes help create subsurface images.The process involves:
- Generating seismic waves using explosives or vibroseis trucks
- Collecting reflected waves with geophones
- Processing data to produce subsurface images
Anticline: A geological fold that is convex up and has its oldest beds at its core, significant in trapping oil and gas in fold structures.
Imagine a large geophysical survey over an unexplored basin. Using reflection seismology, seismic waves indicate a potential trap with an anticline structure. Data analyze suggest high acoustic impedance contrast, a sign of possible hydrocarbons trapped below.
Advances in technology such as 3D and 4D seismic imaging allow more accurate predictions of oil and gas reservoir locations.
Understanding Earth’s Subsurface Structures
Beyond oil and gas, reflection seismology plays a crucial role in understanding Earth's subsurface structures. It provides insights into crustal and mantle's composition, helping researchers infer geological processes and tectonic history.This application extends to:
- Mapping faults and folds
- Investigating volcanic regions
- Assessing potential areas for geothermal energy
Consider a tectonically active region where researchers use reflection seismology to map underground faults and lithological variations. These seismic images allow geologists to predict earthquake-prone areas and guide urban planning accordingly.
In the study of subsurface structures, advancements in seismic inversion have added significant depth to the field. This approach transforms seismic data into a detailed subsurface model by estimating physical properties like density and velocity. This process helps decipher complex geological features and resource distribution, proving invaluable for both academic research and practical applications. By applying these techniques, geoscientists can achieve a nuanced understanding of Earth's subsurface dynamics.
reflection seismology - Key takeaways
- Reflection Seismology: A geophysical technique used to map and study subsurface structures by analyzing seismic waves reflected back to the surface.
- Reflection Seismology Method: Involves generating seismic waves and detecting their reflections using geophones, to construct images of subsurface formations.
- Acoustic Impedance in Reflection Seismology: Crucial for understanding how seismic waves are reflected at layer boundaries; it's the product of a medium's density and the speed of sound within it.
- Reflection Seismology Data Interpretation: Involves analyzing wave travel times and amplitudes to infer geological structures, such as faults or resource deposits.
- Difference between Reflection and Refraction Seismology: Reflection studies waves bounced back to the surface, refraction studies bending of waves through subsurface layers.
- Examples of Reflection Seismology Applications: Widely used in oil and gas exploration, mineral deposit mapping, earthquake research, and engineering geology.
Learn faster with the 10 flashcards about reflection seismology
Sign up for free to gain access to all our flashcards.
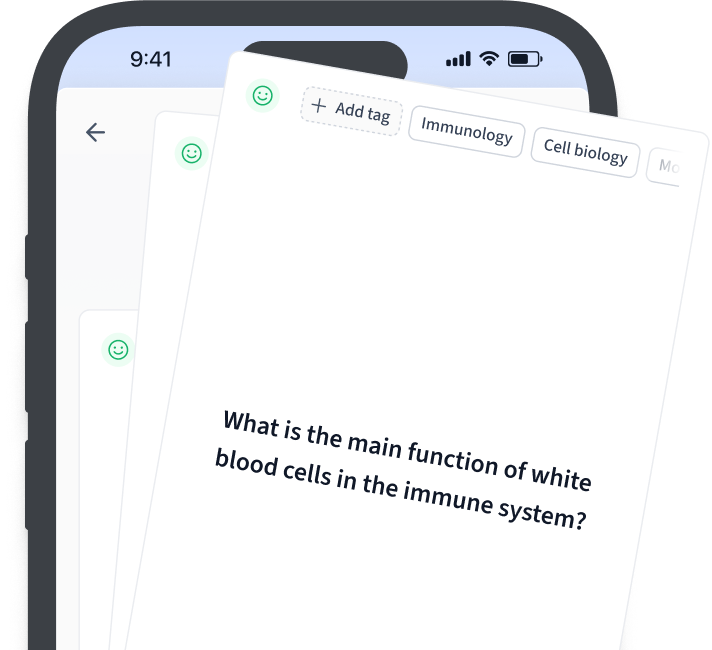
Frequently Asked Questions about reflection seismology
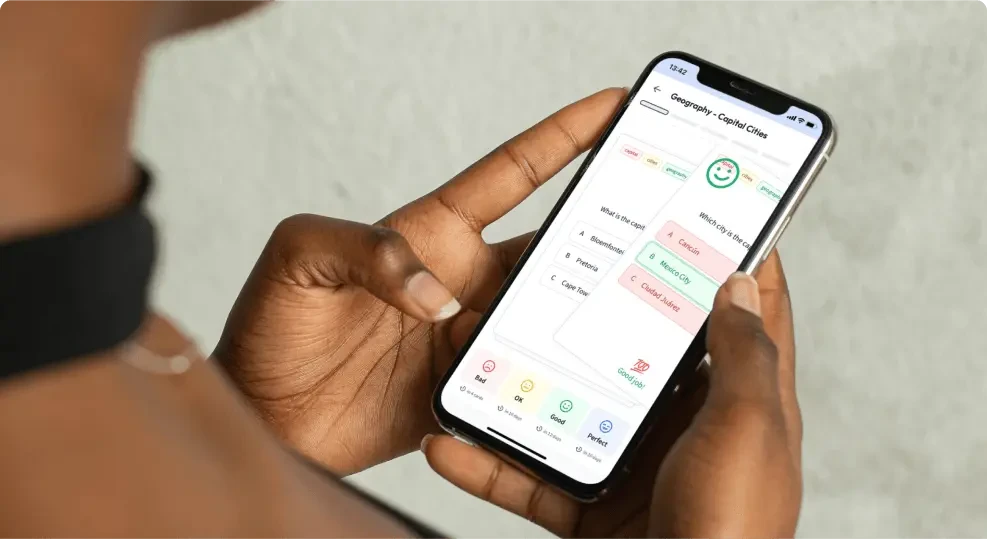
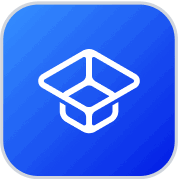
About StudySmarter
StudySmarter is a globally recognized educational technology company, offering a holistic learning platform designed for students of all ages and educational levels. Our platform provides learning support for a wide range of subjects, including STEM, Social Sciences, and Languages and also helps students to successfully master various tests and exams worldwide, such as GCSE, A Level, SAT, ACT, Abitur, and more. We offer an extensive library of learning materials, including interactive flashcards, comprehensive textbook solutions, and detailed explanations. The cutting-edge technology and tools we provide help students create their own learning materials. StudySmarter’s content is not only expert-verified but also regularly updated to ensure accuracy and relevance.
Learn more