Jump to a key chapter
Seismic Anisotropy Explained
Seismic anisotropy is a crucial concept in environmental science that refers to the variation of seismic wave velocities in different directions. It can significantly affect how seismic waves are interpreted and understood, particularly in the context of geophysical exploration and seismology.
Understanding Seismic Anisotropy
Seismic anisotropy arises due to the directional dependency of seismic wave speeds as they travel through various geological structures. This phenomenon can be observed in materials that have an aligned internal structure, such as crystals, or in layered geological formations. The variations in wave propagation are essential in understanding subsurface characteristics that impact numerous applications like oil exploration and earthquake studies. In a simple scenario, consider a wave propagating in an isotropic medium. The velocity of these waves remains constant regardless of the direction. However, in an anisotropic medium, different directions will yield different velocities due to the intrinsic properties of the materials.A mathematical representation of seismic anisotropy can be seen in the variation of wave velocities expressed as: \[ v(\theta) = v_0 (1 + \frac{a}{2} \cdot \theta^2) \]In this equation, \( v_0 \) represents the reference velocity, \( \theta \) is the angle of wave propagation relative to a chosen axis, and \( a \) is a coefficient representing the degree of anisotropy. This formula illustrates how the seismic velocity changes with direction, highlighting the anisotropic nature of the medium.
Structural geology, mineral alignment, and stress fields are common contributors to seismic anisotropy.
Importance of Thomsen Parameters in Seismic Anisotropy
Thomsen parameters are critical when quantifying the level of seismic anisotropy within a material. Developed by Leon Thomsen, these parameters allow you to assess the anisotropy in practical applications, particularly when analyzing vertical transverse isotropy (VTI), which is prevalent in many geological formations.The most relevant Thomsen parameters are:
- \( \varepsilon \): Describes the difference in velocity between horizontal and vertical wave propagation.
- \( \gamma \): Indicates the anisotropy in shear wave splitting.
- \( \delta \): Reflects the variability linked to near-vertical wave propagation.
Accurate estimation of Thomsen parameters improves seismic imaging quality and aids in hydrocarbon exploration.
Factors Influencing Seismic Anisotropy
Seismic anisotropy can be influenced by various factors inherent to the Earth's materials. Recognizing these factors is crucial for interpreting seismic data accurately and effectively. The key factors include:
- Mineral Alignment: Crystallographic alignment within rocks can lead to directional dependence in wave velocity.
- Layering: Sedimentary layers with varying compositions and orientations significantly affect wave speeds.
- Stress Fields: Tectonic and local stress conditions can create anisotropy by deforming materials and changing their wave propagation characteristics.
- Pore Fluid Saturation: The presence and type of fluids in rock pores impact seismic wave velocity, which in turn affects anisotropy.
- Cracks and Fractures: Pre-existing cracks and fractures within the rocks can cause seismic waves to disperse anisotropically.
In-depth studies reveal that seismic anisotropy is not only a tool for understanding subsurface dynamics but also a critical factor in earthquake prediction and hazard assessment. Advanced models integrating seismic anisotropy have improved our ability to forecast seismic activity and understand fault mechanics better. For instance, VTI and horizontal transverse isotropy (HTI) models have been instrumental in decoding the anisotropic characteristics of fault zones, providing more accurate simulations of potential seismic events. These developments underscore the importance of continued research and technological advancement in the field of seismic anisotropy.
Seismic Anisotropy in the Earth
Seismic anisotropy occurs when seismic waves travel at different speeds depending on their propagation direction within the Earth's structure. This phenomenon is critical for geophysical exploration and understanding subsurface geology.
Earth's Layers and Seismic Anisotropy
The Earth is composed of several layers, including the crust, mantle, and core, each having unique characteristics that influence seismic anisotropy. These layers cause variations in seismic wave velocities which are pivotal in understanding geological features.In the crust, for example, the presence of stratified rock layers and varying mineral compositions can lead to significant seismic anisotropy. This impacts seismic waves, particularly their velocity and amplitude.Consider a situation where seismic waves pass through the mantle. In this section, the differing mineral orientations and high pressure conditions result in directional velocity changes. This can be formulated as:\[ v(\theta) = v_0 + b \cdot \sin^2(\theta) \]where \( b \) is a coefficient related to mantle properties and \( \theta \) is the angle of wave propagation.
Seismic Anisotropy: Variation in the velocity of seismic waves depending on their direction of travel through the Earth's layers.
Anisotropy in the mantle is often due to the alignment of olivine crystals.
Seismic Anisotropy and Attenuation in the Earth's Crust
In the crust, seismic anisotropy is influenced by rock formations, mineral alignment, and micro-fractures. These factors also contribute to attenuation, where seismic energy decreases as waves travel.Attenuation varies based on the crust's properties such as:
- Pore fluid content – Fluids in the rock can absorb seismic energy, thereby increasing attenuation.
- Crack distribution – The orientation and density of cracks affect both anisotropy and attenuation.
- Temperature changes – Fluctuations in temperature can alter material properties, impacting wave transmission.
High attenuation in regions may indicate the presence of gases or fluids, impacting exploratory decisions.
Studying Seismic Anisotropy for Earthquake Analysis
Understanding and analyzing seismic anisotropy is essential for earthquake research and hazard prediction. By examining wave patterns, scientists can infer stress distribution within tectonic plates, aiding in the prediction of seismic events.Investigations involve:
- Waveform modeling – Simulating wave propagation through anisotropic media to decode stress directions.
- Shear wave splitting analysis – Studying the delay between fast and slow shear waves to map regional stress fields.
- Deploying seismometers – Using networked sensors to gather detailed seismic data over wide areas for better accuracy.
Seismic Anisotropy of Fractured Rock
Understanding seismic anisotropy in fractured rock helps in deciphering the complexities of the Earth's subsurface. This concept is vital for various applications, including natural resource exploration and earthquake studies. Seismic anisotropy describes how seismic waves vary in speed and direction as they interact with fractures within rocks.
Characteristics of Fractured Rocks
Fractured rocks are characterized by a network of cracks and fissures, which significantly influence their geophysical properties. These fractures can develop from natural occurrences like tectonic stress or human activities such as mining. They have several notable characteristics such as:
- Irregular shapes and sizes, which can range from microscopic cracks to large faults.
- Variable orientations, often aligned with the prevailing stress field.
- Filled or partially filled with fluids, which can alter the rock's physical and chemical properties.
Consider a seismic survey where waves are sent through two types of rock: one is homogeneous and the other fractured. In the homogeneous rock, seismic waves propagate uniformly. However, in the fractured rock, they refract and reflect irregularly, pointing out the anisotropic nature of the medium.
Fracture density is a key parameter affecting rock porosity and permeability, influencing resource extraction efficiency.
Measuring Seismic Anisotropy in Fractured Rocks
When it comes to measuring seismic anisotropy in fractured rocks, a detailed understanding of wave propagation and data collection techniques is essential. Methods often employed include:
- Surface seismic surveys: These involve deploying an array of geophones to record waves at the Earth's surface.
- Borehole measurements: Instruments are lowered into wells to capture data directly within the rock formations.
- Shear wave splitting: The analysis of shear waves splitting into two distinct paths due to anisotropy.
Advanced technologies like 3D seismic tomography are increasingly used to create detailed models of anisotropic properties in fractured rocks. These technologies offer high-resolution insights, which help in analyzing minor changes in wave speed and directionality caused by fracture patterns. They facilitate better resource estimation and geotechnical evaluations, enhancing our understanding of subsurface heterogeneities. Such advancements have significantly improved prediction accuracy in exploratory geophysics.
Implications for Resource Exploration
Seismic anisotropy in fractured rocks has profound implications for resource exploration, particularly in hydrocarbons and mineral deposits. By accurately assessing anisotropy, exploration geologists can:
- Identify reservoir properties such as porosity and permeability.
- Assess potential yields in oil & gas exploration by understanding subsurface formations.
- Make informed decisions on drilling practices, reducing risk and improving recovery rates.
Mantle Dynamics and Seismic Anisotropy
Understanding the relationship between mantle dynamics and seismic anisotropy is crucial in geophysics. The dynamic processes occurring within the mantle, such as convection and plate movements, significantly impact seismic anisotropy. These processes involve the large-scale flow of solid rocks, which can cause alignment of minerals and, consequently, affect how seismic waves travel through the Earth.
Role of Mantle Flows in Seismic Anisotropy
Mantle flows play a vital role in the development of seismic anisotropy. As mantle convection occurs, it causes the alignment of anisotropic minerals, such as olivine, which influences the propagation of seismic waves. This alignment leads to changes in wave speeds depending on their direction of travel relative to the flow direction.Key aspects of mantle flows contributing to seismic anisotropy include:
- Shear flow: It causes lattice-preferred orientation (LPO) in minerals, primarily affecting wave velocities.
- Temperature variations: They influence the mechanical behavior of the mantle material, impacting anisotropic properties.
- Partial melting: This process can alter both the composition and the anisotropy within the mantle material.
Recent studies using high-resolution seismic tomography have unveiled complex flow patterns in the mantle that are not only horizontal but also display vertical components. These findings suggest that mantle plumes and upwellings significantly contribute to anisotropic variations, offering new insights into mantle convection models. Such models could help in understanding hotspot dynamics and the intricate behavior of the mantle beneath tectonic plates.
Consider the Pacific region, where mantle flow beneath tectonic plates influences seismic imaging. The alignment of minerals due to flow direction causes fast and slow seismic velocities, helping geophysicists map convection patterns more clearly.
Techniques for Studying Mantle Dynamics
Studying mantle dynamics involves advanced techniques that rely on seismic waves to decipher subsurface activities. The primary methods include:
- Seismic Tomography: This technique uses seismic waves from earthquakes to create three-dimensional models of the Earth’s interior. It identifies variations in wave speed that indicate temperature and compositional differences.
- Shear-Wave Splitting Analysis: Observing the delay of shear waves splitting into fast and slow directions helps in understanding mantle flow and anisotropy. It is pivotal in analyzing directional wave propagation.
- Mineral Physics Experiments: Laboratory simulations of mantle conditions provide insights into mineral behavior under high-temperature and pressure, helping to interpret anisotropic signals.
Advances in machine learning are enhancing the accuracy of seismic tomography models by efficiently processing large datasets from global seismic networks.
Future Research on Mantle Dynamics and Seismic Anisotropy
The future of research in mantle dynamics and seismic anisotropy holds exciting prospects. With technological advancements and improved computational models, understanding of the mantle's behavior will enhance.Areas of focus include:
- Integrating datasets from global and regional seismological studies: This aids in creating comprehensive models that better capture anisotropic properties across various scales.
- High-resolution imaging techniques: Developing more precise tools for imaging mantle structures to clarify the relationship between flow patterns and anisotropic features.
- Interdisciplinary collaborations: Combining insights from geophysics, geology, and mineral physics to refine models of mantle dynamics.
seismic anisotropy - Key takeaways
- Seismic Anisotropy: Variation of seismic wave velocities in different directions due to aligned internal structures or geological formations. It is crucial for geophysical exploration and seismology.
- Thomsen Parameters: Quantify seismic anisotropy in materials. Key parameters include ε (velocity difference in horizontal/vertical propagation), γ (shear wave splitting), and δ (near-vertical propagation variability).
- Factors Influencing Anisotropy: Include mineral alignment, layering, stress fields, pore fluid saturation, and cracks/fractures. These affect wave propagation and anisotropic characteristics.
- Seismic Anisotropy in Earth's Layers: Occurs in the crust, mantle, and core, impacting seismic wave velocities. Mantle dynamics such as convection contribute to anisotropy through mineral alignment.
- Mantle Dynamics and Seismic Anisotropy: Mantle flows cause mineral alignment affecting seismic wave speeds. Techniques like seismic tomography and shear-wave splitting are used to study these dynamics.
- Seismic Anisotropy of Fractured Rocks: Fractures impact wave speed and direction, affecting geophysical properties. Measurement techniques include seismic surveys, borehole data, and shear wave analysis.
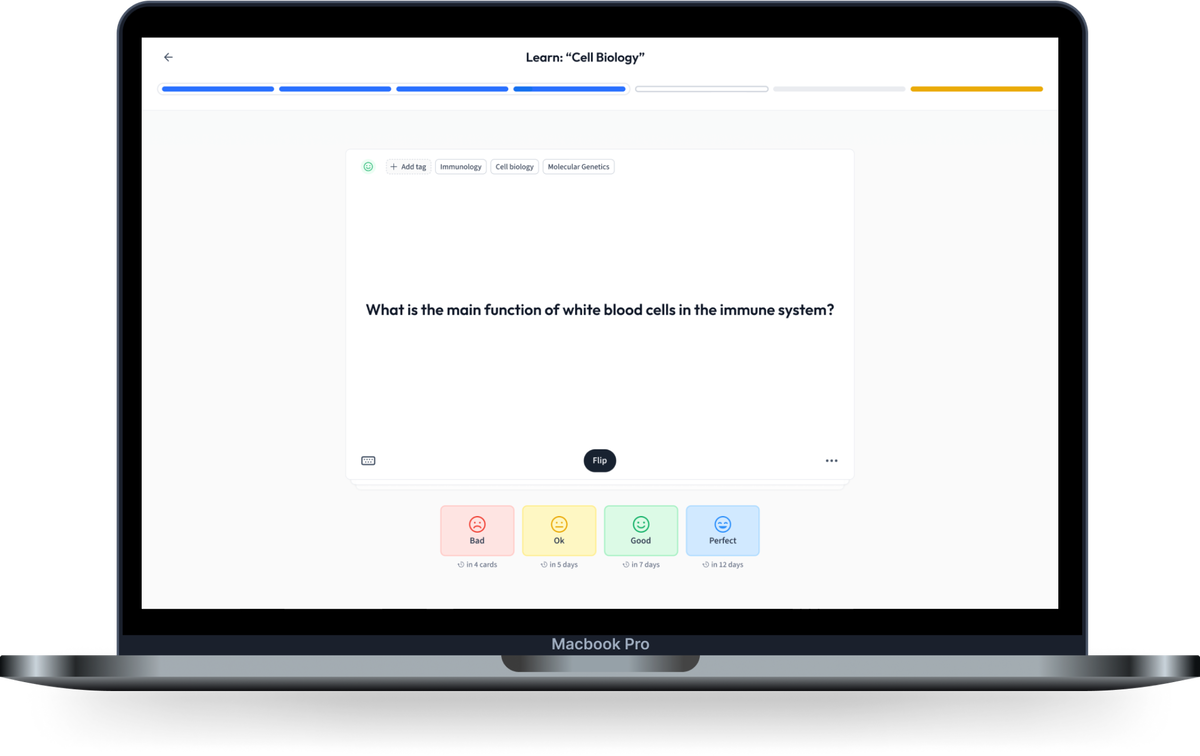
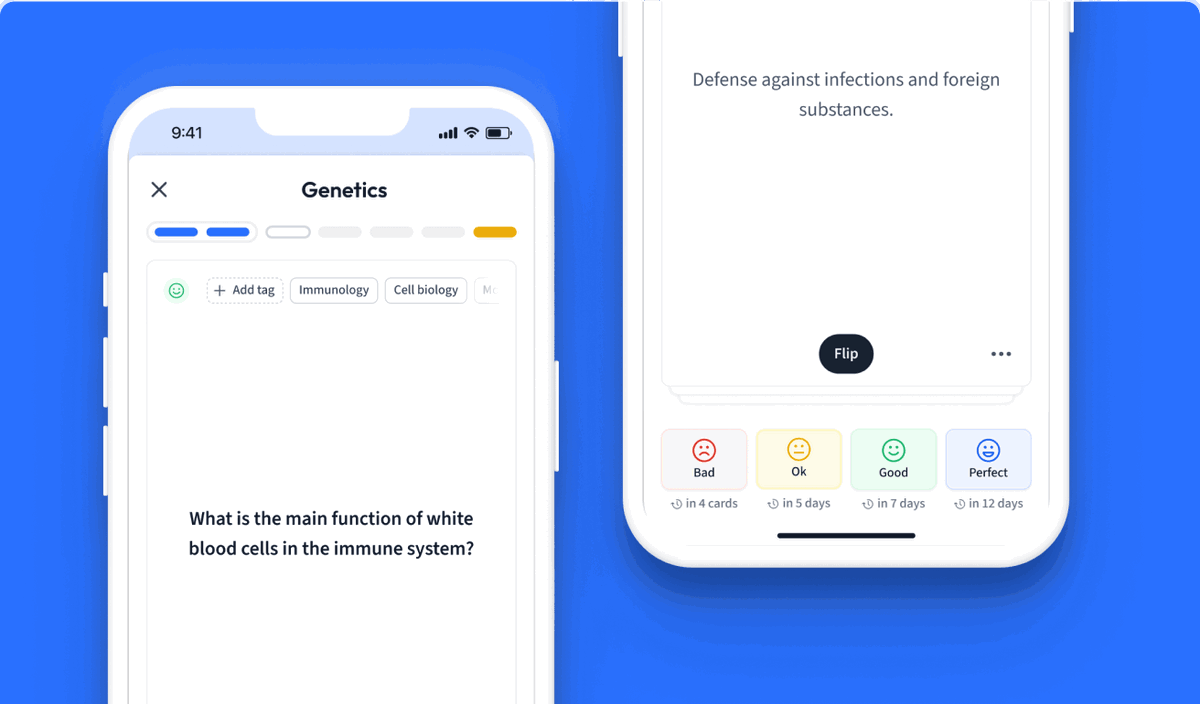
Learn with 12 seismic anisotropy flashcards in the free StudySmarter app
Already have an account? Log in
Frequently Asked Questions about seismic anisotropy
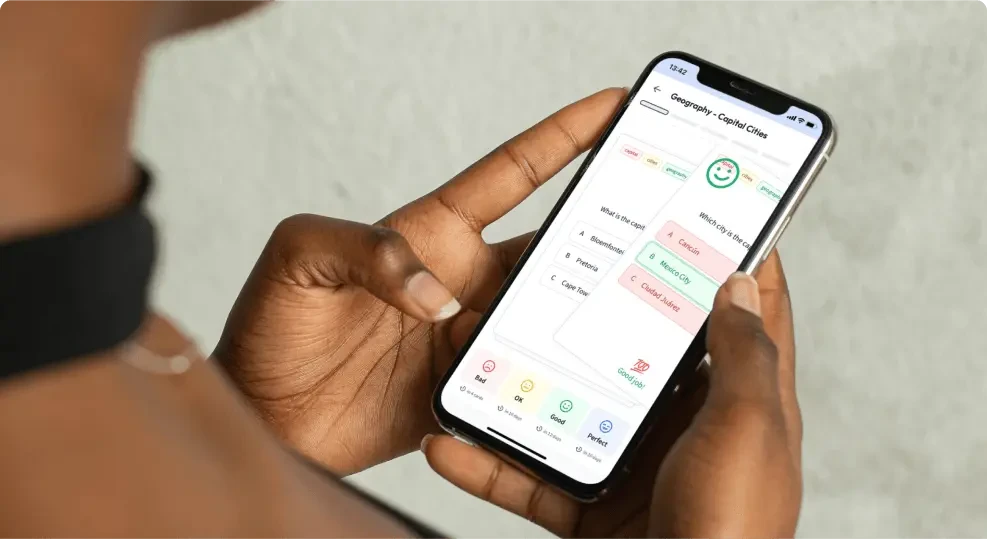
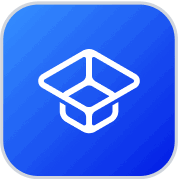
About StudySmarter
StudySmarter is a globally recognized educational technology company, offering a holistic learning platform designed for students of all ages and educational levels. Our platform provides learning support for a wide range of subjects, including STEM, Social Sciences, and Languages and also helps students to successfully master various tests and exams worldwide, such as GCSE, A Level, SAT, ACT, Abitur, and more. We offer an extensive library of learning materials, including interactive flashcards, comprehensive textbook solutions, and detailed explanations. The cutting-edge technology and tools we provide help students create their own learning materials. StudySmarter’s content is not only expert-verified but also regularly updated to ensure accuracy and relevance.
Learn more