Jump to a key chapter
Definition of Strain Partitioning
Understanding strain partitioning is crucial when studying geological and environmental sciences. Strain partitioning refers to the way in which different parts of a rock mass or geological feature deform under stress. The effect of strain partitions often leads to distinct areas within a rock mass experiencing different types of strain or deformation based on the rock’s inherent weaknesses, structures, and the external forces applied. Strain partitioning is significant in understanding geological formations and predicting natural phenomena such as earthquakes and volcanic activity.
Factors Affecting Strain Partitioning
Several factors influence strain partitioning in geological formations. Understanding these factors helps in predicting the behavior of rocks under stress. Here are some key factors that contribute to strain partitioning:
- Composition and Material Properties: Different minerals and compositions within rock strata respond differently to stress, affecting how strain is distributed.
- Pre-existing Structures: Faults, folds, and joints within the rock can guide and concentrate stress in specific directions.
- External Stress Fields: The orientation and magnitude of external stress, such as tectonic forces, play a crucial role in determining the patterns of strain partitioning.
- Temperature and Pressure Conditions: Elevated temperatures and pressures at depth can alter the mechanical properties of rocks, influencing strain distribution.
Strain: In the context of geology and environmental science, strain refers to the deformation or displacement that occurs in a material when it is subjected to stress.
Imagine a block of rock subjected to tectonic movements. As the external forces act on the rock, layers within it may fold, fracture, or shear in various ways. These diverse responses to the same stress are clear evidence of strain partitioning. Observing these effects can provide valuable insights into seismic activity and guide engineering projects, such as tunnel construction or oil extraction.
In regions prone to earthquakes, understanding strain partitioning can help scientists develop better models to assess potential risks and the likelihood of seismic events.
Techniques for Strain Partitioning
Strain partitioning is a crucial concept in environmental science. Understanding how geological formations respond to stress through various techniques can provide important insights. Here, you will explore different techniques used to study strain partitioning in rocks.Recognizing the techniques effectively involves combining fieldwork, laboratory analysis, and computational modeling. Each technique has its own strengths and is often used in conjunction to give a comprehensive understanding of strain behaviors.
Field Techniques
Field techniques are foundational in studying strain partitioning. They involve direct examination and measurement of rock formations in their natural settings. Some primary field techniques include:
- Geological Mapping: Involving the documentation of rock types, structures, and deformational features on maps.
- Structural Analysis: Observing and measuring orientations of faults, folds, and fractures to interpret stress and strain patterns.
- Strain Markers: Use features like fossils or mineral veins as reference points to measure strain quantitatively.
Geological mapping has been pivotal since the 19th century when the idea of mapping geological features gained prominence. It helps in not only understanding strain partitioning but also provides a historical context about the Earth's development. This long-standing technique continues to evolve with technology, using digital tools and satellite data to enhance traditional mapping methods.
Laboratory Methods
Laboratory methods are essential for gaining a deeper understanding of the physical properties of rocks and how they deform under various conditions. Key laboratory techniques include:
- X-ray Diffraction (XRD): Used to identify mineralogical compositions and any changes due to strain.
- Thin Section Analysis: Microscopic examination of rock slices to study mineral alignment and deformation textures.
- Mechanical Testing: Simulating stress conditions to observe how rocks deform, including compressive, tensile, and shear tests.
For thin section analysis, a small rock piece is cut, glued to a slide, and polished thin enough for light to pass through. Under a polarizing microscope, this allows for the observation of crystalline structures and deformations, providing insights into the rock's history of strain.
Computational Modeling
Computational modeling is increasingly important in understanding complex strain partitioning scenarios. Models help simulate tectonic forces and predict how they may influence geological formations. Techniques in computational modeling include:
- Finite Element Analysis (FEA): Breaking down structures into discrete elements to calculate deformation responses.
- Geophysical Simulation: Combining physics-based models with data to understand subsurface strain distribution.
Combining all three techniques—field, laboratory, and computational—yields the most comprehensive understanding of strain partitioning, as each method complements the others by filling in different types of data.
Examples of Strain Partitioning in Geography
In geography and environmental science, observing examples of strain partitioning can provide significant insights into how Earth's surface reacts to various stresses. These examples help us understand the complex interactions within the Earth's crust and predict potential environmental changes.
Tectonic Plate Boundaries
Tectonic plate boundaries are prime examples of strain partitioning. These regions showcase how strain is divided among different geological structures. At tectonic margins, plate movements apply enormous stress to rocks, leading to diverse deformations. Examples include:
- Transform Boundaries: Here, plates slide past one another, showing shear strain along faults like the San Andreas Fault in California.
- Convergent Boundaries: At these locations, plates collide, resulting in compressive strain that forms mountain ranges such as the Himalayas.
- Divergent Boundaries: Plates move apart, producing extensional strain evident at mid-ocean ridges like the Mid-Atlantic Ridge.
The Himalayas demonstrate strain partitioning as the Indian Plate converges with the Eurasian Plate. The motion results in both thrust faults where rocks are pushed upwards to form mountains and strike-slip faults running parallel to the collision zone.
Studying active tectonic regions not only provides insight into the current geological activity but also offers clues about past tectonic events that shaped the Earth's surface.
Volcanic Regions
Volcanic regions also illustrate strain partitioning due to the stress exerted by magma movement. The way strain is distributed can affect volcanic eruptions and landform creation.
- Caldera Formation: Massive volcanic eruptions can cause calderas, where the ground collapses due to the evacuation of magma.
- Lava Flows and Dykes: These are formed from extensional strain as magma forces its way through crustal weaknesses.
- Tuff Rings: Created by explosive volcanic activity, they show compressive strain from rapid gas expansion.
Yellowstone National Park is an example of a volcanic region where strain partitioning can be seen in features like geysers, hot springs, and the caldera itself, all influenced by underlying magma dynamics.
Calderas represent some of the largest volcanic features on Earth and form a crucial aspect of volcanic studies. Some calderas, like Yellowstone, host geothermal features due to the heat remaining from volcanic activity. Studying these helps scientists understand the repeated cycles of volcanic super-eruptions and their global impacts.
Sedimentary Basins
Sedimentary basins present a different aspect of strain partitioning where the stress of sediment accumulation and tectonic forces create a unique set of conditions. Examples include:
- Flexural Basins: Forming at thrust belts where the crust bends under the weight of stacked sediments.
- Rift Basins: Created due to crustal extension and subsequent deposition of sediments in the resultant trough.
- Sag Basins: Occurring where the crust sags due to regional subsidence, influenced by broader tectonic or isostatic adjustments.
Causes and Applications of Strain Partitioning in Geography
The concept of strain partitioning plays a vital role in understanding geological processes and their implications in geography. By dissecting how different areas within the Earth's crust react differently to stress, you can better predict geological activity and its effects on the environment. Strain partitioning leads to diverse forms of deformation such as folding, faulting, and fracturing, each influenced by the structure and properties of the Earth's layers.
Strain Partitioning and Strain Ellipse
Understanding the relationship between strain partitioning and the strain ellipse is crucial for interpreting geological formations. The strain ellipse is a conceptual tool used to depict how a material deforms, exhibiting changes in length and orientation in response to stress. In strain partitioning, different sections of rock may experience varying degrees of deformation. The strain ellipse graphically represents this by showing how the original circular shape becomes an ellipse, demonstrating the intensity and direction of strain. This visualization aids geologists in mapping out regions of stress concentration and eventual deformation patterns.
Strain Ellipse: A graphical representation used to describe the distortion of a material subjected to stress, highlighting the principal axes of stretch and compression.
The axes of the strain ellipse can be mathematically described by calculating the principal strains. Consider a general orthogonal coordinate system: - Principal strain directions can be determined using the eigenvectors of the strain tensor. - The principal strains, also the radii of the strain ellipse, are given by the eigenvalues. The equations below illustrate this reflection: The 2D strain state can be described by the transformation of circle into an ellipse where: \[\text{Principal strains} = \frac{\text{change in length}}{\text{original length}}\]
Imagine a clay surface subjected to pressure from different directions. When flattened, it adopts a new shape where crossed lines become ellipses. This transformation is analogous to the geological concept of the strain ellipse, used to understand the deformation changes in large-scale rock formations.
The strain ellipse is a simplification, but a powerful one. It encapsulates complex 3D deformations into a more accessible 2D model for clearer understanding of rock behavior under stress.
Delving deeper into the mathematics of strain partitioning, the analysis of the strain tensor is key. The strain tensor (\textbf{e}) for 2D deformations is given as: \[\textbf{e} =\begin{pmatrix} e_{xx} & e_{xy} \ e_{xy} & e_{yy} \end{pmatrix}\]\The matrix components represent normal strains \(e_{xx}, e_{yy}\) and shear strain \(e_{xy}\). By finding the eigenvalues of this matrix, you determine the magnitude of the principal strains, representing the transformation of the original geometry into the strain ellipse. Such deepened understanding can aid in more precise geological mappings and predictions.
- Mathematical analysis allows for precise predictions in geotechnical engineering and seismic risk assessments.
strain partitioning - Key takeaways
- Definition of Strain Partitioning: Refers to how different parts of a geological feature deform differently under stress due to inherent weaknesses and external forces.
- Techniques for Strain Partitioning: Includes field techniques (geological mapping, structural analysis), laboratory methods (X-ray diffraction, mechanical testing), and computational modeling (finite element analysis).
- Examples in Geography: Tectonic plate boundaries, volcanic regions, and sedimentary basins showcase various types of strain partitioning under geological stresses.
- Causes of Strain Partitioning: Influenced by composition, pre-existing structures, external stress fields, and temperature/pressure conditions.
- Applications in Geography: Understanding strain partitioning assists in predicting earthquakes, volcanic activity, and helps in engineering projects like tunnel construction.
- Strain Partitioning and Strain Ellipse: The strain ellipse visually represents how geological formations deform, aiding geologists in mapping stress distribution and deformation.
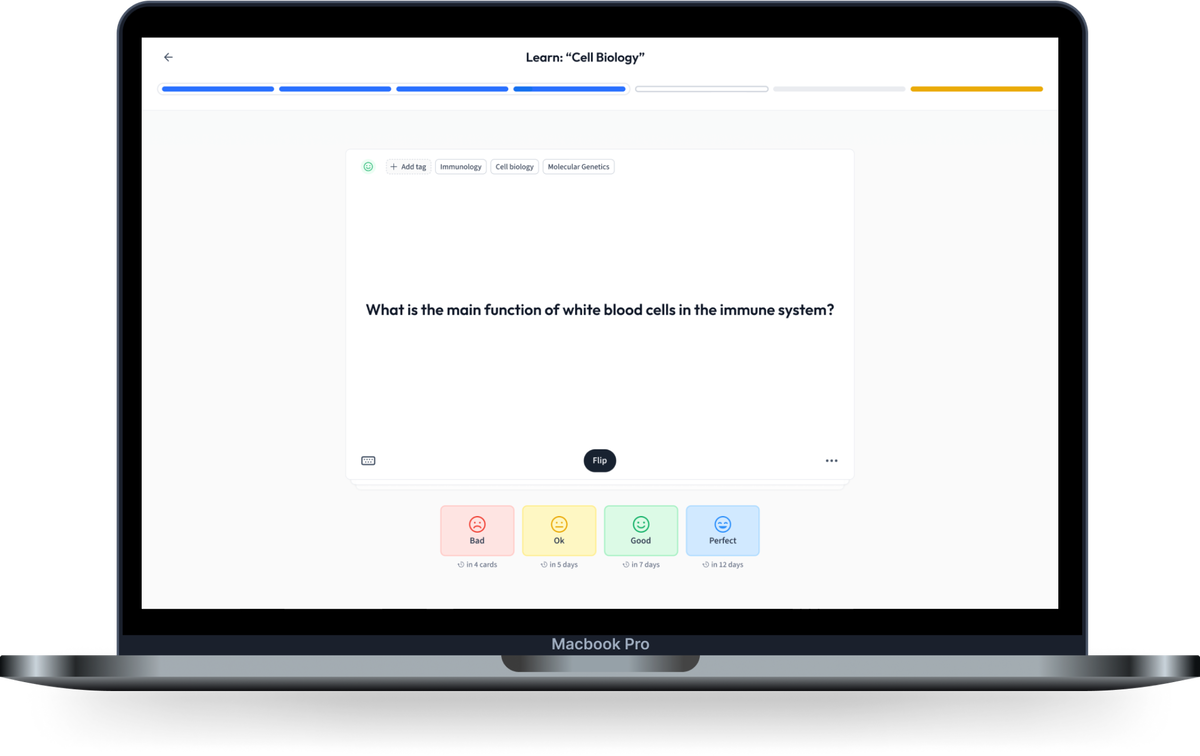
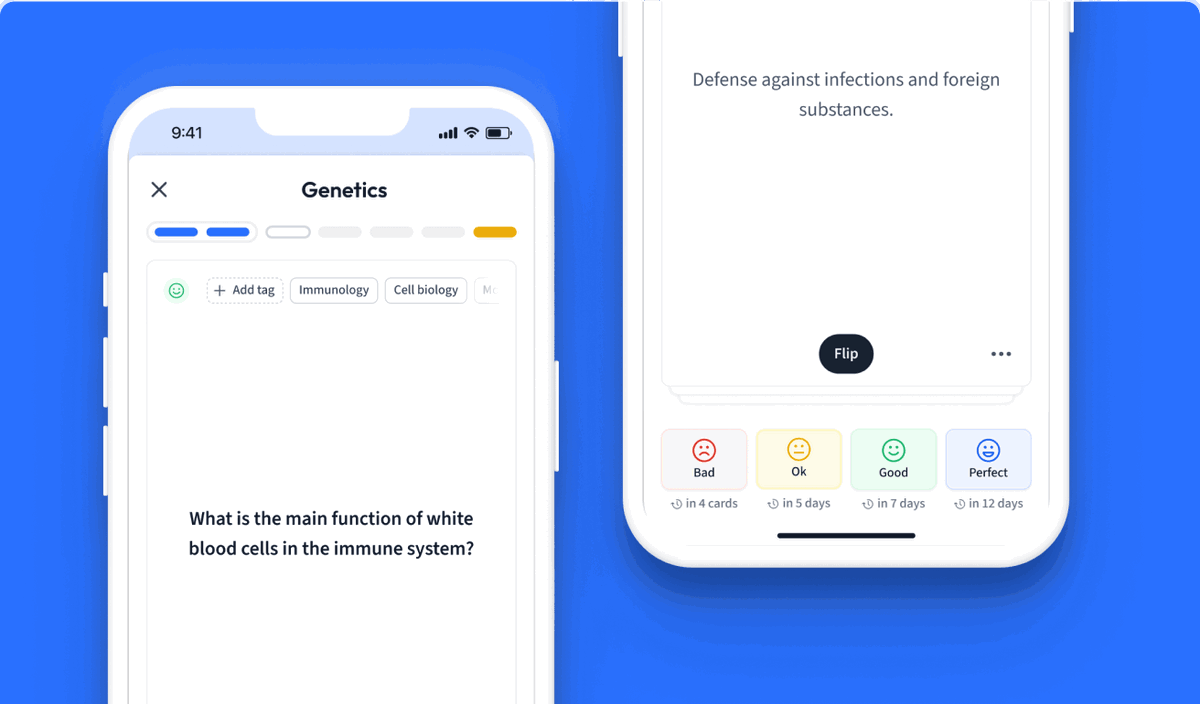
Learn with 12 strain partitioning flashcards in the free StudySmarter app
Already have an account? Log in
Frequently Asked Questions about strain partitioning
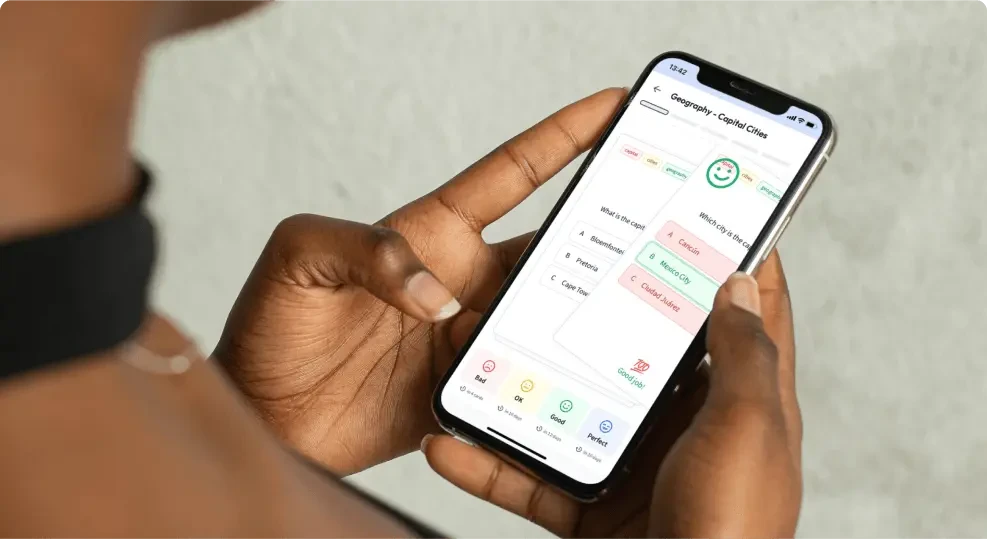
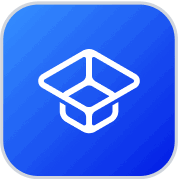
About StudySmarter
StudySmarter is a globally recognized educational technology company, offering a holistic learning platform designed for students of all ages and educational levels. Our platform provides learning support for a wide range of subjects, including STEM, Social Sciences, and Languages and also helps students to successfully master various tests and exams worldwide, such as GCSE, A Level, SAT, ACT, Abitur, and more. We offer an extensive library of learning materials, including interactive flashcards, comprehensive textbook solutions, and detailed explanations. The cutting-edge technology and tools we provide help students create their own learning materials. StudySmarter’s content is not only expert-verified but also regularly updated to ensure accuracy and relevance.
Learn more