Jump to a key chapter
Thermodynamics in Geochemistry Definition
Thermodynamics in Geochemistry is the study of the energy transformations and material reactions that occur within the Earth's crust and other planetary environments. It integrates principles from both thermodynamics and geochemistry to understand geological processes, including mineral reactions, fluid-rock interactions, and the stability of geological materials.
Basic Concepts of Thermodynamics in Geochemistry
To begin with, thermodynamics in geochemistry revolves around the laws of thermodynamics, which govern energy transformations. Let's delve into these fundamental laws:
- The First Law of Thermodynamics, or the law of energy conservation, states that energy cannot be created or destroyed in an isolated system. Mathematically, it is expressed as \( \Delta U = Q - W \), where \( \Delta U \) is the change in internal energy, \( Q \) is the heat added to the system, and \( W \) is the work done by the system.
- The Second Law of Thermodynamics introduces the concept of entropy, a measure of disorder or randomness. It states that in any energy exchange, if no energy enters or leaves the system, the potential energy of the state will always be less than that of the initial state, often expressed as \( \Delta S \geq 0 \).
- The Third Law of Thermodynamics states that as temperature approaches absolute zero, the entropy of a perfect crystal approaches a constant minimum.
Thermodynamics also involves the use of phase diagrams which are graphical representations of physical states of a substance under different conditions of temperature and pressure. Phase diagrams can help determine the stability fields of minerals and predict changes in mineral assemblages over geological timescales. These diagrams are often constructed using the principles of Gibbs free energy (\(G\)), which combines enthalpy (\(H\)) and entropy (\(S\)) into the formula: \( G = H - TS \, \ \text{where } T \text{ is temperature}.\) Thus, changes in phase can be predicted by analyzing how \( G \) varies with temperature and pressure, allowing geochemists to assess the stability of various minerals and infer the conditions under which they formed.
Importance of Thermodynamics in Geochemistry
Thermodynamics plays a critical role in understanding geochemical processes on Earth. Some of the key areas where thermodynamics is applied include:
- Mineral Stability: Predicting the stability and transformations of minerals under varying temperature and pressure conditions is essential for understanding geological formations and metamorphic processes.
- Fluid-Rock Interaction: Thermodynamics helps in analyzing interactions between fluids and rocks, crucial for understanding hydrothermal systems and ore deposit formations.
- Environmental Applications: Understanding the thermodynamics of pollutant interactions with natural minerals can aid in predicting and mitigating environmental contamination.
Remember, thermodynamics is not just about heat. It's a fundamental tool used to describe many geological phenomena.
Laws of Thermodynamics in Geochemistry
Understanding the laws of thermodynamics is essential for grasping how energy transformations drive geological processes. Let's explore how each law is specifically applied within the realm of geochemistry.
First Law of Thermodynamics in Geochemistry
First Law of Thermodynamics is also known as the law of energy conservation, which states that energy in an isolated system can neither be created nor destroyed, only transformed from one form to another. In the context of geochemistry, this law helps in studying the energy changes that occur during chemical reactions and phase changes in geological materials.
In geochemical processes, the internal energy change (\( \Delta U \)) of a system is equal to the heat (\(Q\)) added to the system minus the work (\(W\)) done by the system, expressed as:\[ \Delta U = Q - W \]This principle is particularly important when analyzing mineral formation and dissolution reactions. For instance, during the formation of a mineral from its constituent elements, energy is consumed, while the dissolution process releases energy.
An example of applying the first law is when calculating the energy required to precipitate calcite (CaCO3) from aqueous solutions. By knowing the initial and final states of the system, geochemists can determine how much energy is absorbed or released.
Second Law of Thermodynamics in Geochemistry
Second Law of Thermodynamics introduces the concept of entropy, which is the measure of disorder or randomness. This law predicts that the total entropy of an isolated system can only increase over time.
The second law is pivotal in geochemistry because it dictates the direction of energy transformations and chemical reactions. Processes that increase the system's entropy are naturally favored, as seen in spontaneous chemical reactions or phase changes.The mathematical representation is:\[ \Delta S \geq 0 \]Where \( \Delta S \) is the change in entropy. This principle is used to predict the stability of minerals and their transformations under different environmental conditions. For instance, the recrystallization of minerals during metamorphism is guided by entropy changes.
Entropy can be thought of as nature's tendency to become disordered, driving reactions towards greater randomness.
Third Law of Thermodynamics in Geochemistry
Third Law of Thermodynamics states that as the temperature approaches absolute zero, the entropy of a perfect crystal approaches zero. This concept is crucial for studying entropy at very low temperatures.
While primarily theoretical, the third law provides insight into how entropy and thermodynamic properties of substances behave at extremely low temperatures. It helps refine calculations of entropies of various substances, which in turn assists in predicting geochemical processes.For example, the standard entropies of many crystalline minerals are often derived from extrapolating values at low temperatures using data obtained near absolute zero. This can be essential in thermodynamic modeling of natural systems to understand the favorability of certain reactions over others as temperatures change.
Application of Thermodynamics in Geochemistry
The application of thermodynamics in geochemistry provides crucial insights into the processes that shape the Earth's crust. By using thermodynamic principles, geochemists can predict the behavior of minerals, fluids, and gases under various conditions.
Thermodynamics in Geochemistry Techniques
Techniques in thermodynamics applied to geochemistry involve various methods to predict and understand geological processes. Key techniques include:
- Gibbs Free Energy Calculations: This technique evaluates the spontaneity of a reaction through Gibbs free energy \( \Delta G \), calculated by the formula \( \Delta G = \Delta H - T \Delta S \), where \( \Delta H \) is the change in enthalpy and \( \Delta S \) is the change in entropy.
- Phase Diagrams: These diagrams display stability fields of minerals under different temperature and pressure conditions, helping to predict phase transformations.
- Activity-Composition Relationships: This involves understanding how the chemical activity of different components in a geochemical system affects mineral stability.
Calculating the stability of quartz (SiO2) involves using its Gibbs free energy data at various temperatures and pressures. By determining where \( \Delta G \) is minimized, we can delineate the conditions under which quartz is stable.
A deeper exploration into Gibbs Free Energy reveals its vital role in connecting thermodynamics with chemical equilibria. In geochemistry, Gibbs free energy not only dictates reaction direction but also provides insights into mineral solubility and electrochemical processes. For instance, in aqueous geochemistry, the solubility product (Ksp) is directly tied to Gibbs free energy changes. By calculating \( \Delta G = -RT \ln Ksp \), where \( R \) is the gas constant and \( T \) is the temperature in Kelvin, geochemists can deduce the solubility limits of minerals in water, which is crucial for predicting mineral precipitation and dissolution in natural waters.
Real-world Examples of Thermodynamics Applications
Thermodynamics in geochemistry isn't just theoretical; it finds practical application across numerous contexts. These real-world examples illustrate its impact:
- Ore Formation: Thermodynamic principles elucidate how temperature and pressure conditions facilitate the concentration of metals, aiding in the discovery and extraction of mineral deposits.
- Petroleum Geochemistry: Understanding thermodynamic reactions helps in modeling the generation, migration, and accumulation of hydrocarbons.
- Soil Chemistry: Thermodynamics informs the stability and mobility of nutrients and pollutants, crucial for agriculture and environmental remediation strategies.
In Earth sciences, thermodynamics serves as an invaluable tool for predicting the past and present conditions of geological processes.
Thermodynamics in Geochemistry: The Equilibrium Model
Thermodynamics plays a pivotal role in geochemistry, especially when it comes to understanding equilibrium models, which describe the behavior of minerals and fluids under varying conditions. These models help predict the chemical distribution in geological environments.
In geology, a state of equilibrium is achieved when the rate of the forward reaction equals the rate of the reverse reaction, resulting in a stable system. This concept is fundamental in evaluating natural geochemical cycles, such as the carbon cycle, where carbonate formation and dissolution reach a balance. The equilibrium constant \( K \) is related to Gibbs free energy change (\( \Delta G \)) by the equation:\[ \Delta G = -RT \ln K \]where \( R \) is the gas constant and \( T \) is the temperature in Kelvin. This equation becomes essential in quantifying the position of equilibrium in various mineral reactions.
Understanding Equilibrium in Geochemical Processes
In geochemistry, achieving equilibrium implies that a system's chemical composition remains constant over time at a given set of conditions. Understanding equilibrium is crucial for predicting mineral stability and the fate of dissolved species in natural waters.
- Phase Equilibrium: Refers to the balance between different mineral phases. The behavior of minerals at equilibrium is often represented in phase diagrams which illustrate the stability of different phases across temperature and pressure ranges.
- Solution Equilibrium: Involves both the dissolution and precipitation of minerals. At equilibrium, the rate of minerals dissolving is equal to the rate of precipitation, which defines their solubility limits.
Consider the dissociation of calcite (CaCO3) in water:\[ \text{CaCO}_{3(s)} \rightleftharpoons \text{Ca}^{2+}_{(aq)} + \text{CO}_{3(aq)}^{2-} \]The equilibrium constant for this reaction is \( K_{sp} = [\text{Ca}^{2+}][\text{CO}_{3}^{2-}] \). This constant helps geochemists determine the solubility of calcite under various environmental conditions.
Thermodynamic Calculations in Geochemical Equilibrium
Thermodynamic calculations are essential tools in geochemistry for predicting the outcome of reactions at equilibrium. By incorporating parameters such as Gibbs free energy, enthalpy, and entropy, calculations can determine the favorability of processes.Gibbs Free Energy (\( \Delta G \)): Determines the spontaneity of reactions. A negative \( \Delta G \) indicates a reaction proceeds spontaneously. The relationship to equilibrium is expressed by:\[ \Delta G = \Delta G^\circ + RT \ln Q \]Here, \( \Delta G^\circ \) is the standard Gibbs energy change, \( R \) is the universal gas constant, \( T \) is the temperature, and \( Q \) is the reaction quotient.Calculating Equilibrium Constants: Use the standard Gibbs energy of formation to calculate \( \Delta G^\circ \), then determine \( K \) using the equation:\[ K = e^{-\Delta G^\circ / RT} \]These calculations enable geochemists to model processes such as mineral saturation states and the solubility equilibrium of compounds in aquatic systems.
Thermodynamic data tables for mineral phases provide crucial insights, ensuring accurate calculations of equilibrium constants.
An intriguing aspect of thermodynamic equilibrium in geochemistry is the concept of metastability. Some minerals can exist in a stable state that is not the most stable configuration. For example, diamonds are metastable; they will eventually convert to graphite under Earth's surface conditions, but this reaction is kinetically slow. Metastable states reflect an important nuance where kinetics can inhibit the transformation to the true equilibrium state.
thermodynamics in geochemistry - Key takeaways
- Thermodynamics in Geochemistry Definition: Study of energy transformations and material reactions within Earth's crust and planetary environments.
- Laws of Thermodynamics in Geochemistry: Energy conservation, entropy increase, and entropy at absolute zero govern geological processes and chemical reactions.
- Thermodynamics Principles in Geochemistry: Use of phase diagrams and Gibbs free energy to predict mineral stability and geological material transformation.
- Application of Thermodynamics in Geochemistry: Critical for mineral stability, fluid-rock interaction, and environmental applications.
- Thermodynamics in Geochemistry Techniques: Include Gibbs free energy calculations, phase diagrams, and activity-composition relationships.
- Thermodynamics in Geochemistry: The Equilibrium Model: Understanding equilibrium models to predict chemical distribution and mineral stability under varying conditions.
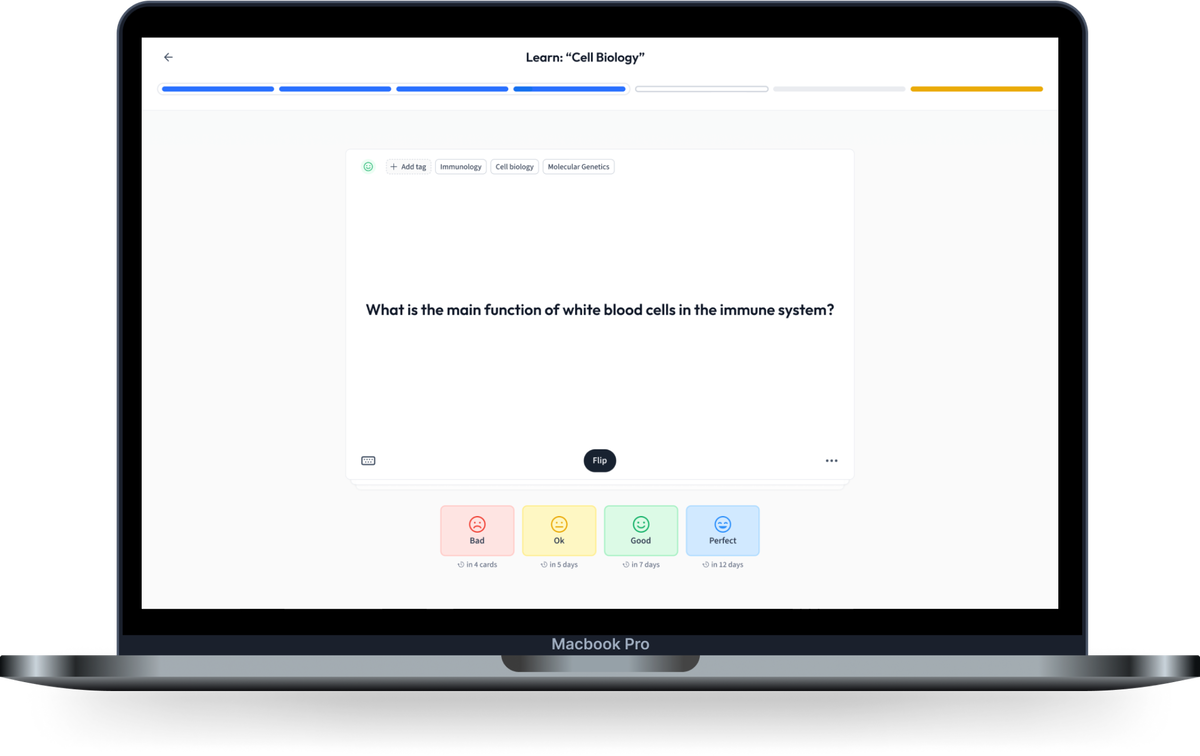
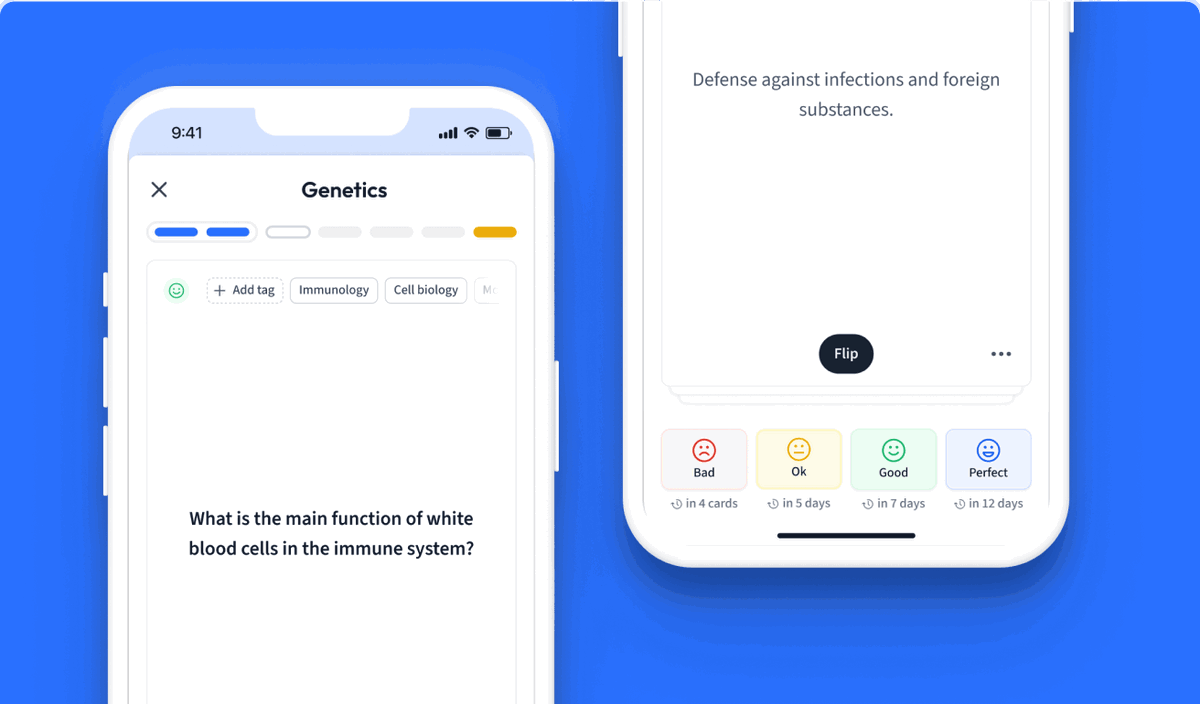
Learn with 12 thermodynamics in geochemistry flashcards in the free StudySmarter app
Already have an account? Log in
Frequently Asked Questions about thermodynamics in geochemistry
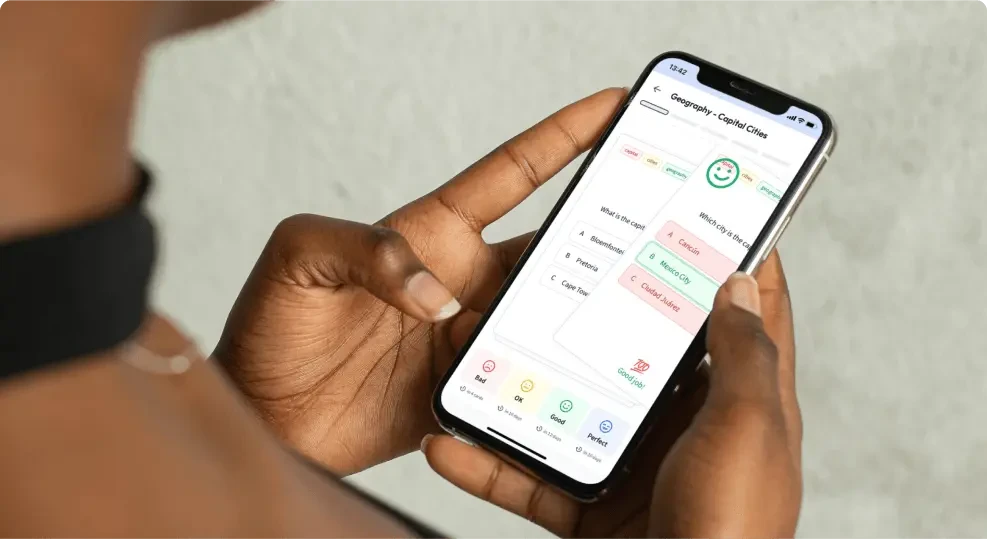
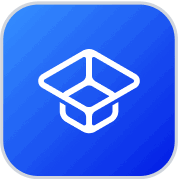
About StudySmarter
StudySmarter is a globally recognized educational technology company, offering a holistic learning platform designed for students of all ages and educational levels. Our platform provides learning support for a wide range of subjects, including STEM, Social Sciences, and Languages and also helps students to successfully master various tests and exams worldwide, such as GCSE, A Level, SAT, ACT, Abitur, and more. We offer an extensive library of learning materials, including interactive flashcards, comprehensive textbook solutions, and detailed explanations. The cutting-edge technology and tools we provide help students create their own learning materials. StudySmarter’s content is not only expert-verified but also regularly updated to ensure accuracy and relevance.
Learn more