Jump to a key chapter
Understanding Geophysical Fluid Dynamics
Geophysical Fluid Dynamics (GFD) explores the flow of fluids on Earth and other planets. Through understanding GFD, one can unravel the complexities of the atmosphere, oceans, and even the mantle's behaviour. This field is quintessential for studying climate change, weather forecasting, and natural hazards.
The Basics: Fundamentals of Geophysical Fluid Dynamics
At its core, Geophysical Fluid Dynamics involves studying fluid motion on the planet's surface and its atmosphere. This encompasses various scales, from small eddies to large ocean currents and atmospheric weather systems. The principles of fluid mechanics, combined with the rotating reference frame of the Earth, lay the foundation for understanding these dynamic systems.
Fluid mechanics: The study of fluids (liquids, gases, and plasmas) and the forces on them. It is a branch of physics that includes the study of fluid motion, fluid statics, and the application of these principles in engineering.
Key equations, such as the Navier-Stokes equations, provide the mathematical framework for modelling fluid flows within the geophysical context. However, due to the Earth's rotation, additional forces such as the Coriolis effect and centrifugal forces come into play, further complicating these models.
Diving into the Deep: Key Concepts and Theories
Grasping the key concepts and theories in Geophysical Fluid Dynamics enables one to understand the diverse behaviours of planetary fluids. Among these are the concepts of vorticity, waves in fluids, buoyancy, and thermodynamics, each playing a critical role in fluid movement and energy transfer.
Vorticity: A measure of the rotation of fluid elements in a flow field. It's fundamental in the study of turbulent flows and is a core concept in understanding cyclones, anticyclones, and other atmospheric phenomena.
Example of vorticity in action: The spiralling winds of a hurricane demonstrate high vorticity, with air rotating rapidly around a low-pressure centre.
The theory of waves in fluids explains the propagation of different types of waves through oceans and atmospheres, impacting weather patterns and ocean currents. Understanding these waves requires a firm grasp on the principles of buoyancy, which describes the upward force that keeps objects afloat, and thermodynamics, explaining how energy converts within the fluid.
Geophysical Fluid Dynamics in the Natural World
The manifestation of Geophysical Fluid Dynamics in the natural world is profound and ubiquitous. From the formation of clouds to the circulation of the world's oceans, GFD principles are at play. Additionally, the dynamics of volcanic eruptions and mantle convection beneath Earth's crust are explored within this discipline, linking it closely with geological sciences.
Mantle convection: A process by which heat from Earth’s interior is transported to the surface through the convective movement of mantle rocks. This is crucial for understanding plate tectonics, volcanic activity, and the thermal evolution of the Earth.
The global ocean circulation, often referred to as the "global conveyor belt," exemplifies large-scale fluid dynamics driving heat and salt across tremendous distances, impacting climate worldwide. Atmospheric circulation patterns, influenced by both oceanic and terrestrial factors, dictate weather patterns and climatic zones across the globe.
The study of GFD not only demystifies the natural processes on Earth but also aids in predicting future environmental changes and natural disasters, showcasing the immense practical importance of this field.
Lectures on Geophysical Fluid Dynamics
Lectures on Geophysical Fluid Dynamics play an invaluable role in the education of students and researchers alike. These sessions provide a structured way to navigate through the complex principles and applications of the discipline, making the subject more accessible and engaging.
Structuring Learning: How Lectures Enhance Understanding
Lectures serve as a cornerstone for learning Geophysical Fluid Dynamics, offering a structured format that facilitates understanding. By systematically breaking down concepts, theories, and methodologies, lectures help demystify the subject matter.Lecturers often utilise a variety of teaching aids, including slideshows, diagrams, and mathematical derivations, to convey the intricate details of fluid dynamics. Through these aids, abstract concepts become tangible, making complex topics like the Navier-Stokes equations or the Coriolis effect more comprehensible.
One of the key benefits of structured lectures in GFD is the opportunity for real-time interaction. Questions posed during lectures spark dialogue, giving rise to deeper understanding and clarification of complex concepts. This interactive element can be especially beneficial for applying theoretical knowledge to practical scenarios, such as environmental modelling and climate prediction.
From Theory to Practice: Exploring Real-Life Applications
Understanding the practical applications of Geophysical Fluid Dynamics breathes life into the theoretical concepts discussed in lectures. For instance, examining the ocean's thermohaline circulation or the dynamics of atmospheric storms illustrates how fluid dynamics principles operate in real-world situations.Through case studies and project work, students are encouraged to apply their knowledge, tackling challenges in weather forecasting, climate modelling, and even the study of planetary atmospheres. Such activities not only reinforce learning but also underscore the relevance of GFD in addressing critical environmental issues.
Real-life application example: The use of satellite data and GFD principles in tracking and predicting the path of hurricanes. By analysing atmospheric pressure patterns, temperature gradients, and fluid movement, scientists can forecast a hurricane's trajectory and intensity, potentially saving lives and reducing property damage.
Field trips to coastal areas or research facilities can also serve as an extension to classroom lectures, offering hands-on experience with the physical processes studied in geophysical fluid dynamics.
Moreover, advancements in computational fluid dynamics have ushered in a new era of interactive learning. Students can now simulate fluid movement under various conditions, providing a dynamic way to visualise and understand the flows within Earth's atmospheres and oceans.Such practical exercises are crucial for bridging the gap between theory and application, preparing students for careers in environmental science, meteorology, oceanography, and related fields.
Numerical Methods in Geophysical Fluid Dynamics
Numerical Methods in Geophysical Fluid Dynamics provide the tools for simulating and understanding the complex behaviour of fluids within Earth's atmosphere, oceans, and beyond. These methods are crucial for advancing research in meteorology, oceanography, and even in the study of astrophysical phenomena.
Cracking the Code: Introduction to Numerical Methods for Wave Equations
Numerical methods for wave equations are designed to simulate waves within fluids, a fundamental aspect of geophysical dynamics. These equations, complex in nature, describe how waves propagate through different mediums, such as air or water.The most common numerical method used is the finite difference method (FDM), which discretises the continuous domain into a grid of finite points. By approximating differential terms, FDM translates wave equations into solvable algebraic equations.
Finite Difference Method (FDM): A numerical technique used for approximating solutions to differential equations by using finite difference approximations for the derivatives.
Application example: The finite difference method applied to the wave equation \[\frac{\partial^2 u}{\partial t^2} = c^2 \frac{\partial^2 u}{\partial x^2}\] to model seismic waves passing through Earth’s layers.
For solving wave equations numerically, it's essential to ensure stability and accuracy of the numerical method to prevent errors from overwhelming the solution.
Advanced Techniques: Numerical Methods for Fluid Dynamics with Applications to Geophysics
Advanced numerical methods, including Finite Volume and Spectral Methods, have broadened the scope of simulating fluid dynamics in geophysical settings. Finite Volume Methods (FVM) conserves fluxes across control volumes, making it particularly suited for conserving mass, momentum, and energy in fluid flows.Spectral Methods, relying on the transformation of equations into the frequency domain, excel in handling problems with periodic boundary conditions, offering higher accuracy in certain scenarios.
Spectral Method: A numerical technique that solves differential equations by decomposing the solution into its Fourier series and computing the coefficients of the series.
Code example for FVM: for each controlVolume in domain: calculateFluxes(controlVolume) updateProperties(controlVolume)This pseudo-code outlines the basic steps in a finite volume method applied in fluid dynamics simulations.
Understanding the computational effort and complexity of these advanced methods is critical. While they offer enhanced accuracy, they also demand significantly more computational resources, making the choice of method dependent on the problem's requirements and available computational power.
Bridging Disciplines: Geophysical & Astrophysical Fluid Dynamics
The study of Geophysical Fluid Dynamics does not only apply to Earth. Its principles and numerical methods extend to astrophysical fluid dynamics, exploring the movement of fluids in stars and around planets in other solar systems. This interdisciplinary approach aids in understanding celestial phenomena, such as stellar atmospheres and protoplanetary disks.Methods like Magnetohydrodynamics (MHD) are pivotal in studying the behaviour of ionised gases in magnetic fields, crucial for both geophysical and astrophysical applications.
Magnetohydrodynamics (MHD): The study of the magnetic properties and behaviour of electrically conducting fluids, such as plasmas, liquid metals, and salt water.
Applying numerical methods in astrophysical fluid dynamics opens vistas for understanding not only the Earth and its immediate environment but also the broader universe, showcasing the versatility of Geophysical Fluid Dynamics.
Practical Applications of Geophysical Fluid Dynamics
Geophysical Fluid Dynamics (GFD) is a pivotal tool in understanding and solving practical problems related to Earth's atmosphere, oceans, and beyond. The applications of GFD range from improving weather forecasts to exploring the atmospheric conditions of other planets in our universe.
Understanding Earth's Climate: A Geophysical Fluid Dynamics Approach
Geophysical Fluid Dynamics offers a framework to dissect and understand the complexities of Earth's climate system. By applying GFD principles, scientists dissect the intricate interactions between the atmosphere, oceans, and land surfaces.Through the use of numerical modeling, researchers can simulate climate dynamics over decades, helping to predict changes in climate patterns, assess the impacts of global warming, and develop strategies for mitigation and adaptation.
Numerical modeling: A mathematical tool used in GFD to simulate the physical processes of fluid flows on Earth and other celestial bodies by solving equations that describe these processes.
Simulation example: Using GFD models to simulate the melting of polar ice caps and its subsequent effect on global sea levels and ocean currents.
Forecasting and Modelling: Geophysical Fluid Dynamics in Action
Forecasting weather and modeling environmental systems are among the most tangible applications of Geophysical Fluid Dynamics. Through complex mathematical models, meteorologists predict weather patterns, such as storms, heatwaves, and hurricanes, with increasing accuracy.Environmental hazard models, another GFD application, allow for the simulation of tsunamis, floods, and landslides. These models are indispensable for early warning systems, helping to save lives and reduce economic losses.
The process of improving predictive models is ongoing, with scientists regularly incorporating real-time data from satellites, weather stations, and ocean buoys into GFD models. This continuous refinement enhances model accuracy, making predictions more reliable over time.
Exploring the Universe: Geophysical Fluid Dynamics in Astrophysics
Beyond Earth, Geophysical Fluid Dynamics extends its reach to the field of astrophysics, aiding in the exploration of atmospheric phenomena within our solar system and beyond. GFD principles are used to study the atmospheric conditions on other planets, the gas and dust dynamics in accretion disks around stars, and the behaviour of stellar winds.The study of planetary atmospheres, particularly, provides insights into the fluid dynamics of alien worlds, such as Jupiter's Great Red Spot, a massive storm that has been raging for centuries. By understanding such phenomena, scientists can infer the physical properties and climate conditions of distant planets.
GFD's application in astrophysics demonstrates its importance not only in solving Earth-based problems but also in uncovering the mysteries of the universe, highlighting the interdisciplinary nature of the field.
Geophysical Fluid Dynamics - Key takeaways
- Geophysical Fluid Dynamics (GFD): Studies fluid flow on Earth and other planets, crucial for understanding climate change, weather patterns, and natural hazards.
- Fundamentals of Geophysical Fluid Dynamics: Involves principles of fluid mechanics with Earth's rotation, incorporating forces like the Coriolis effect in fluid flow models.
- Navier-Stokes Equations: Core mathematical framework in GFD, modelling fluid flows which are influenced by Earth’s rotation.
- Numerical Methods for Wave Equations in GFD: Techniques like the finite difference method, used to simulate fluid waves and understand their propagation through mediums.
- Practical Applications of GFD: Wide-ranging uses from improving weather forecasts and understanding Earth's climate to exploring atmospheric conditions on other planets.
Learn faster with the 0 flashcards about Geophysical Fluid Dynamics
Sign up for free to gain access to all our flashcards.
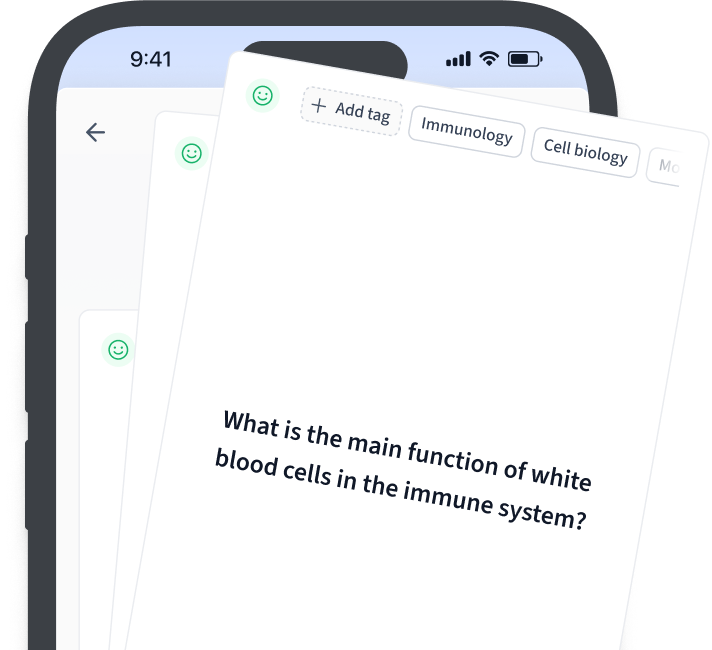
Frequently Asked Questions about Geophysical Fluid Dynamics
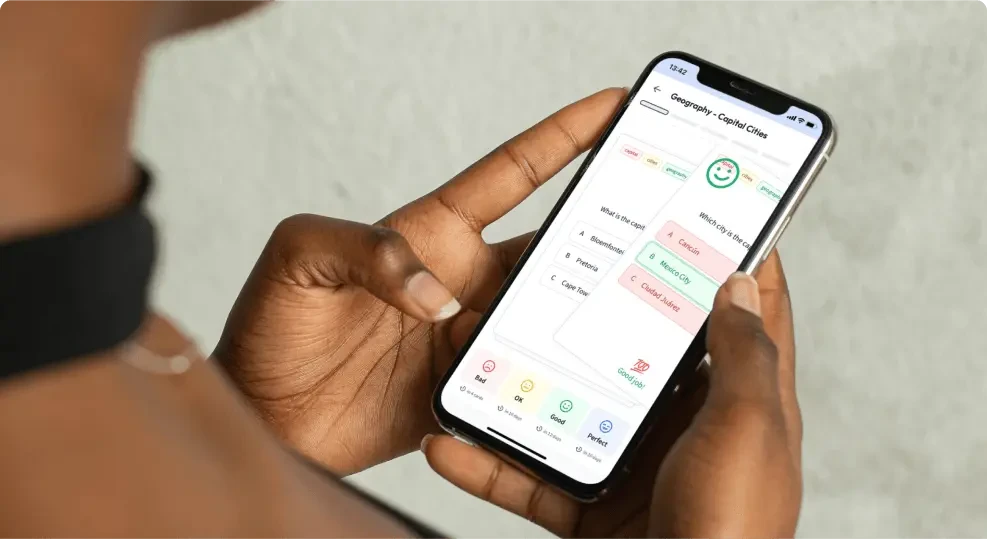
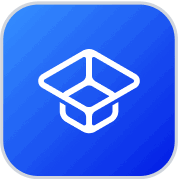
About StudySmarter
StudySmarter is a globally recognized educational technology company, offering a holistic learning platform designed for students of all ages and educational levels. Our platform provides learning support for a wide range of subjects, including STEM, Social Sciences, and Languages and also helps students to successfully master various tests and exams worldwide, such as GCSE, A Level, SAT, ACT, Abitur, and more. We offer an extensive library of learning materials, including interactive flashcards, comprehensive textbook solutions, and detailed explanations. The cutting-edge technology and tools we provide help students create their own learning materials. StudySmarter’s content is not only expert-verified but also regularly updated to ensure accuracy and relevance.
Learn more