Jump to a key chapter
Forensic Metallurgy Definition
Forensic Metallurgy involves the analysis of metals and metallic components to determine their origins, causes of failure, or involvement in crimes. This field utilizes various scientific methods to draw conclusions about metallic objects that might be evidence in legal cases.
Forensic Metallurgy: A branch of science that studies metals and metallic objects to uncover evidence pertaining to legal investigations and failures.
Example of Forensic Metallurgy in Action: Suppose a component of a bridge has failed suddenly, resulting in a collapse. Engineers may use forensic metallurgy to examine fractures in the metal, identifying fatigue, corrosion, or flawed manufacturing processes as potential causes.
Remember, forensic metallurgy often deals with both civil and criminal cases, such as accidents or deliberate sabotage.
To effectively analyze a metallic sample, forensic metallurgists use a variety of techniques and tools:
- Microscopy: Allows the observation of microstructural properties.
- Spectroscopy: Offers information about the chemical composition.
- X-ray diffraction: Provides data on crystalline structures.
Forensic metallurgy is not limited to metals; it may extend to all sort of alloys and composite materials. This field delves into the understanding of how materials react under different stress conditions like temperature and load. For instance, understanding phase diagrams can provide invaluable insights into temperature-dependent structural changes:The Iron-Carbon Phase Diagram is a crucial tool, showing how different percentages of carbon in steel influence its properties. The eutectoid point, represented by pearlite in steels with 0.77% carbon, illustrates a perfect balance of hardness and ductility. Whenever materials undergo sudden changes, such as the transition from ferrite to austenite in the iron lattice at 912°C, forensic metallurgists can explore whether external factors like thermal stress or chemical exposure might have influenced these transitions. This knowledge is critical when evaluating failures that occur under specific environmental conditions. Through these analyses, forensic metallurgists can answer critical questions, such as was the failure caused by natural fatigue or was there an element of malice, such as sabotage?
Forensic Metallurgy Techniques
Forensic metallurgy employs a variety of scientific techniques to analyze metals and metallic components. This analysis helps in deducing the origins, characteristic properties, or causes of failure of the metallic evidence that might play a role in legal cases.
Common Techniques in Forensic Metallurgy
Common techniques in forensic metallurgy involve both qualitative and quantitative analyses to identify the properties and composition of metallic samples. Some of these techniques include:
- Microscopy: Uses electron or optical microscopes to observe metal surface structures, grain sizes, and fracture surfaces.
- Spectroscopy: Determines the elemental composition through methods like X-ray fluorescence or atomic absorption spectroscopy.
- X-ray Diffraction (XRD): Provides information on crystalline structures and phase composition by measuring the diffraction pattern of X-rays passing through the material.
An example of these techniques in practice is solving a structural failure case. Suppose a bridge collapses, and forensic metallurgists must determine the cause. By using microscopy, they may discover fatigue cracks indicative of prolonged stress. Spectroscopy might reveal chemical elements inconsistent with the material's intended use, suggesting contamination or exposure to damaging substances. X-ray diffraction analysis may show inappropriate phase structures, pointing to a possible heat treatment error during manufacturing.
Metallography is another term often used in forensic metallurgy, involving studying the microstructure of metallic samples with the help of microscopes.
Advanced Techniques and Tools
Beyond basic techniques, advanced methodologies in forensic metallurgy provide deeper insights into metallic evidence. Methods such as:
- Scanning Electron Microscopy (SEM): Offers higher magnification and resolution compared to conventional microscopy, useful for detailed surface examinations.
- Energy Dispersive X-ray Spectroscopy (EDS): Often coupled with SEM for precise elemental analysis.
- Laser Ablation Inductively Coupled Plasma Mass Spectrometry (LA-ICP-MS): Facilitates trace element analysis at very low concentrations.
The application of advanced mathematical models in forensic metallurgy enhances accuracy in failure predictions. For example, the Paris' Law is a critical contribution to crack growth analysis:\[ \frac{da}{dN} = C (\Delta K)^m \]This equation models the rate of crack growth per cycle (\frac{da}{dN}), where \(C\) is a material-specific constant, \(\Delta K\) is the stress intensity factor range, and \(m\) is the material constant. Understanding these parameters allows forensic experts to predict how quickly cracks might propagate under cyclic loading conditions.By employing numerical software, metallurgists can simulate different loading conditions and environmental factors. The convergence of physics-based models and actual forensic evidence can reveal timeline approximations for when materials might fail, thereby providing critical insights for preventative structural integrity assessments. Advanced metallurgical techniques continually evolve by leveraging cross-disciplinary tools and methods to solve complex forensic cases.
Forensic Metallurgy Failure Analysis
Forensic metallurgy failure analysis is the examination of metal components to determine the reasons behind their failure. Understanding these failures often requires a comprehensive evaluation that involves multiple scientific principles and analytical techniques.
Steps in Failure Analysis
The process of failure analysis in forensic metallurgy generally follows several key steps:
- Collection of evidence: Gather samples and data from the failure site for detailed examination.
- Visual inspection: Conduct a thorough visual analysis to identify obvious signs of failure, such as cracks or discoloration.
- Non-destructive testing: Use techniques like ultrasonics or radiography to examine internal flaws without altering the material.
- Metallographic examination: Analyze the microstructure using microscopy to identify metallurgical faults.
- Chemical analysis: Perform spectroscopy to determine the elemental composition and identify any discrepancies or contaminants.
Failure Analysis: A systematic investigation of the root causes leading to the failure of a component or structure.
To illustrate failure analysis, consider a case where a pressure vessel has ruptured unexpectedly during operation. A metallurgist might start with a visual inspection to note any visible corrosion or deformation. Next, non-destructive testing could reveal internal voids or cracks. Further microscopic examination might show fatigue striations indicative of cyclic loading failure. Finally, chemical analysis could detect unexpected sulfur content, suggesting contamination during manufacturing.
Failure analysis can also include computational simulations to predict how similar failures might occur under various operational conditions.
Quantitative Approach in Failure Analysis
A quantitative approach is vital in forensic metallurgy to assess material properties and predict the conditions under which failure might occur. Methods often used include:
- Fracture Mechanics: Evaluation of crack propagation using linear elastic fracture mechanics (LEFM) principles, such as computing stress intensity factors \(K\).
- Fatigue Analysis: Determining the fatigue life of materials using the S-N curve, a plot of stress against the number of cycles to failure.
Analysis Type | Formula |
Stress Intensity Factor \(K\) | \[ K = Y \sigma \sqrt{\pi a} \] |
Paris' Law for Crack Growth | \[ \frac{da}{dN} = C (\Delta K)^m \] |
A deep dive into the field of forensic metallurgy failure analysis reveals its extensive applications, including:
- Aerospace Industry: Regular failure analyses help improve safety standards by investigating component breakdowns.
- Automotive Sector: Metallurgists are involved in failure analysis to enhance the durability and safety of vehicles.
- Structural Engineering: Analysis of building material failures can inform better designs and materials for construction projects.
Forensic Metallurgy Case Study
Forensic metallurgy plays a crucial role in understanding the failure or involvement of metals in various situations. By examining metals thoroughly, forensic metallurgists can uncover details that significantly affect the outcome of legal cases. Below are insights from notable case studies and lessons learned in forensic metallurgy.
Notable Case Studies in Forensic Metallurgy
Highlighted case studies demonstrate the far-reaching impact of forensic metallurgy in solving complex issues involving metal failures.
- Silver Bridge Collapse (1967): A critical failure analysis revealed that the bridge's collapse was due to a defect in a single eyebar, exacerbated by stress corrosion cracking.
- Stainless Steel Surgical Instrument Case: Forensic analysis showed contamination by corrosive agents, causing premature failure of instruments.
- Aircraft Failure Investigation: Metallurgical assessment identified cracks in turbine blades, initially invisible, which led to catastrophic engine failure.
- Cultural Heritage Artifact Analysis: Metallurgists employed non-destructive techniques to study ancient coins, determining their composition and manufacturing methods.
An example of forensic metallurgy's application is the Silver Bridge collapse. Lasers and scanning electron microscopes (SEMs) were used to identify minuscule cracks and defects. The results indicated that poor material choice and lack of maintenance precipitated the failure, underscoring the importance of regular inspections.
Forensic metallurgists in the Silver Bridge case used failure mechanics equations to understand the stress conditions. Applying fracture mechanics:\[ \sigma = \frac{P}{A} \quad \text{and} \quad K = Y \sigma \sqrt{\pi a} \]Where \( \sigma \) is the stress applied, \( P \) is the load, \( A \) is the cross-sectional area, \( K \) is the stress intensity factor, \( Y \) is a geometric factor, and \( a \) is the crack length. These formulas helped in determining that the materials used could not bear the calculated stress values, pinpointing the failure.
Many forensic cases involve interdisciplinary collaboration, merging knowledge from areas like chemistry, engineering, and metallurgy.
Lessons Learned from Case Studies
From forensic metallurgy case studies, several key lessons have emerged:
- Regular Maintenance: Consistent inspection and maintenance can prevent failures, evident from the Silver Bridge case.
- Comprehensive Analysis: Using multiple techniques provides a more complete understanding, as seen in the aircraft turbine analysis.
- Material Selection: Choosing the right materials for specific environments is crucial to prevent failures due to conditions like stress corrosion.
- Continuous Innovation: Adopting new technologies can enhance the accuracy and scope of analysis.
In aircraft failure cases, forensic metallurgists often employ Paris' Law to predict fatigue crack growth rates:\[ \frac{da}{dN} = C (\Delta K)^m \]This formula helps determine the lifespan of materials under cyclic loads, which is crucial for ensuring that aircraft components can withstand operational stresses.
Metallurgical analysis is crucial in the design phase to prevent potential failures.
Further exploration into failure analysis reveals the integration of computational methods for predictive modeling. Numerical simulations, using principles from fluid dynamics and thermodynamics, can anticipate the effects of environmental stressors on metal structures. In the aircraft industry, finite element analysis (FEA) is extensively used to simulate stress distributions and deformation patterns. Such simulations help defensible decisions about material design and safety protocols before manufacturing, leading to better-informed manufacturing processes and reduced risk of operational failures. As computational power increases, the potential for even more precise predictions in forensic metallurgy continues to grow, proving invaluable in the prevention of catastrophic failures across various industries.
Forensic Metallurgy Educational Importance
Understanding forensic metallurgy is important for anyone interested in materials science, engineering, and legal studies. This field bridges the gap between engineering and law, providing critical insights into how metals behave under various conditions and how their failures can be analyzed to provide evidence in legal cases.
Why Learn Forensic Metallurgy?
Learning forensic metallurgy equips you with tools and knowledge to:
- Analyze metal failures that can have significant safety implications in industries like aviation, construction, and manufacturing.
- Understand how materials are selected and processed to ensure safety and longevity.
- Gain skills for forensic analysis that can aid in legal cases involving crime scene evidence or industrial failures.
Consider a case where a high-rise building experiences a catastrophic failure. A forensic metallurgist might be called to analyze the steel beams used in construction. By examining the grain structure of the steel using microscopy techniques, they could determine if the failure was due to material defects or external stressors, such as earthquakes or fire exposure.
Forensic metallurgy is increasingly important as new materials are developed, and existing infrastructures age.
Forensic Metallurgy Explained for Students
At its core, forensic metallurgy is concerned with understanding why metal components fail and what evidence can be gathered from these failures. Here's how you can break it down:
- Observation: Initial inspection of the metal for visible signs of distress or failure.
- Testing: Employing techniques such as x-ray diffraction (XRD) or energy-dispersive x-ray spectroscopy (EDS) to explore the composition and potential defects within the metal.
- Analysis: Using data gathered from tests to determine causes of failure, whether from fatigue, corrosion, or manufacturing flaws.
Stress \( \sigma \) | \( \sigma = \frac{F}{A} \) |
Strain \( \epsilon \) | \( \epsilon = \frac{\Delta L}{L_0} \) |
To further illustrate, imagine an aircraft turbine blade failure attributed to high-cycle fatigue. Forensic metallurgists use concepts like the S-N Curve, which plots stress (S) vs. the number of cycles to failure (N), to predict when and how such components might fail under repeated stress. By understanding the endurance limit from the curve, engineers and metallurgists can set safe operational thresholds for materials. Forensic metallurgy thus combines scientific methodology with practical applications to enhance safety, optimize materials, and support justice.
forensic metallurgy - Key takeaways
- Forensic Metallurgy Definition: A branch of science analyzing metals and metallic components for legal investigations and failure analysis.
- Forensic Metallurgy Techniques: Key methods include microscopy, spectroscopy, and x-ray diffraction for characterizing metal properties.
- Forensic Metallurgy Failure Analysis: Involves steps like evidence collection, visual inspections, non-destructive testing, and chemical analysis to determine failure causes.
- Forensic Metallurgy Case Study: Notable cases like the Silver Bridge collapse highlight the role of forensic metallurgy in identifying failure causes.
- Forensic Metallurgy Explained: The field blends engineering and law, using metal investigations to uncover evidence about failures or crimes.
- Educational Importance: Learning forensic metallurgy builds insights into metal behavior, failure prevention, and contributes to legal case resolutions.
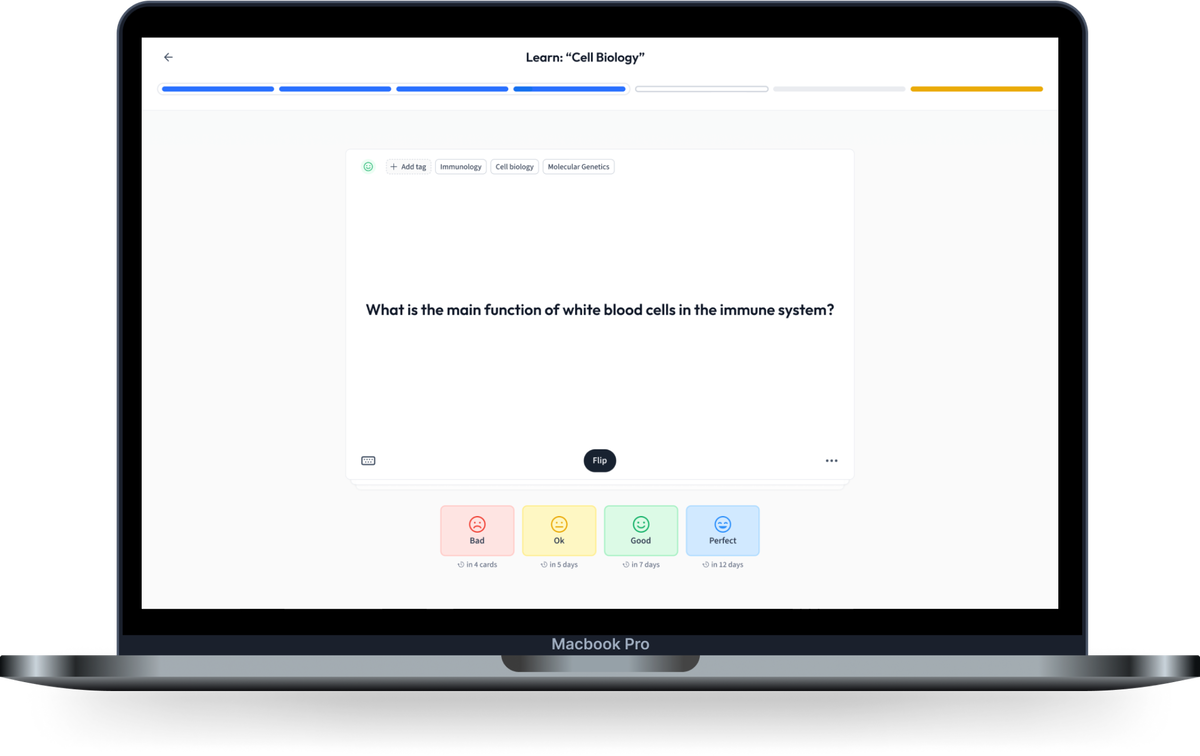
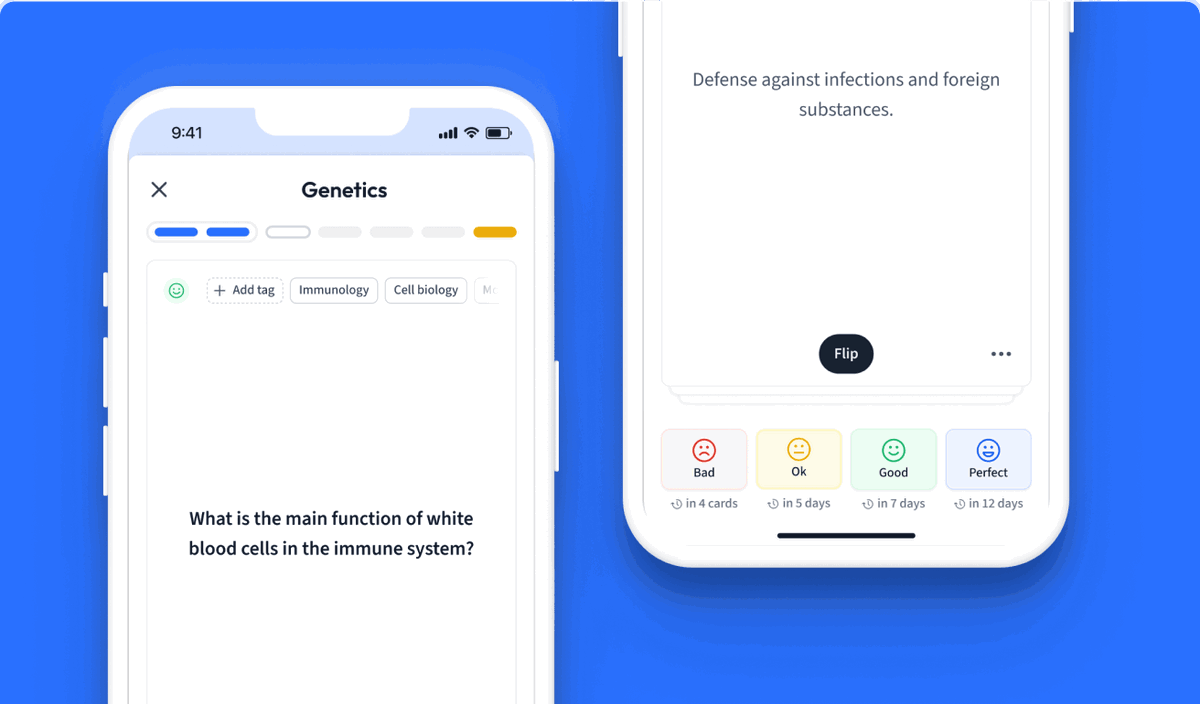
Learn with 10 forensic metallurgy flashcards in the free StudySmarter app
Already have an account? Log in
Frequently Asked Questions about forensic metallurgy
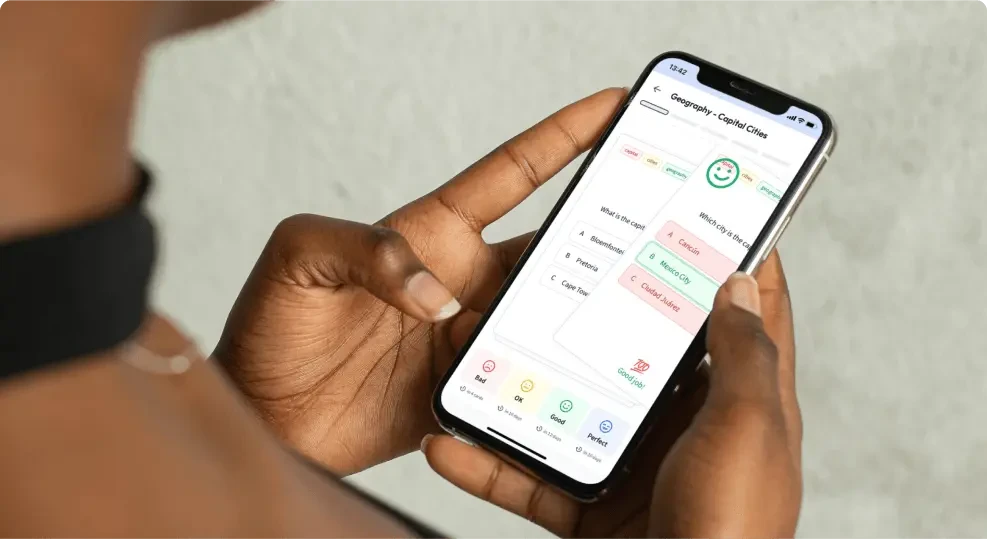
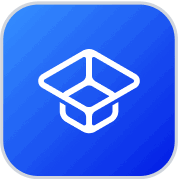
About StudySmarter
StudySmarter is a globally recognized educational technology company, offering a holistic learning platform designed for students of all ages and educational levels. Our platform provides learning support for a wide range of subjects, including STEM, Social Sciences, and Languages and also helps students to successfully master various tests and exams worldwide, such as GCSE, A Level, SAT, ACT, Abitur, and more. We offer an extensive library of learning materials, including interactive flashcards, comprehensive textbook solutions, and detailed explanations. The cutting-edge technology and tools we provide help students create their own learning materials. StudySmarter’s content is not only expert-verified but also regularly updated to ensure accuracy and relevance.
Learn more