Jump to a key chapter
What is Quantum Decoherence?
Quantum Decoherence is a fascinating phenomenon in the realm of quantum mechanics that elegantly describes how quantum systems seem to lose their quantum nature when they interact with the environment. This concept is crucial in understanding the transition from quantum to classical behaviour, bridging the gap between two seemingly incompatible frameworks of physics.
Quantum Decoherence Definition
Quantum Decoherence refers to the process by which a quantum system's coherent superposition state decays into a mixture of states because of the interaction with its environment. This transition is what makes quantum systems appear to adopt definite states as observed in the classical world.
Imagine a quantum particle that exists in a superposition of being in two places at once. When not observed, it behaves like a wave, showing the probabilities for its position. However, once it interacts with the environment, for instance, by hitting a detector, the superposition is destroyed, and the particle appears to have been in only one place all along. This is an example of quantum decoherence in action.
How Quantum Decoherence Works in Quantum Mechanics
In quantum mechanics, quantum decoherence operates through the inevitable interaction of a quantum system with its surroundings. These interactions are not uniform and can involve countless degrees of freedom, leading to the rapid dispersion of quantum coherence. This dispersion mechanism explains why quantum effects like superposition and entanglement are so difficult to observe in larger, macroscopic systems.To understand the concept better, let's break down the dynamics involved in quantum decoherence:
- The environment acts as a reservoir of countless particles, each creating minuscule disturbances.
- A quantum system in a superposition state interacts with these disturbances.
- Through these interactions, information about the superposition spreads over the environment, leading to observable effects that are consistent with classical physics.
For those wanting to delve deeper, the mathematical underpinnings of quantum decoherence involve describing the quantum system and its environment using what's known as the 'density matrix' formalism. This approach allows for a detailed account of how interactions with the environment cause a pure quantum state to evolve into a mixed state. In formulas, the evolution of a system's density matrix, \(\rho\), is governed by the Lindblad equation, which includes terms accounting for these interactions.At the macroscopic level, this results in a rapid transition of quantum behaviour to classical mechanics, explaining phenomena like why cats are not found in a superposition of dead and alive states, à la Schrödinger’s cat thought experiment.
Quantum decoherence doesn't solve the measurement problem in quantum mechanics but it explains how classical realities emerge from quantum rules.
Quantum Decoherence Explained
Quantum Decoherence is a key concept in quantum mechanics that describes the transition of quantum systems from a state of superposition into one where classical physics can take over. This process is central for understanding why the quantum world, with its particles being in multiple states at once, seemingly simplifies to the deterministic world we observe daily.
The Principles Behind Quantum Coherence and Decoherence
At the heart of quantum mechanics lies the principle of quantum coherence, where particles exist in a combination of all possible states simultaneously, a superposition. However, when a quantum system interacts with its environment, this coherence breaks down over time through a process called quantum decoherence. It is through decoherence that quantum probabilities collapse into the distinct outcomes observed in the classical world.Understanding decoherence requires a grasp of two fundamental aspects:
- Interaction with the environment: The inevitable and uncontrollable interaction of quantum systems with external particles or fields.
- Loss of information: The dispersal of quantum information into the environment, making it practically irretrievable.
For instance, consider a photon passing through a double-slit apparatus. Initially, it exhibits quantum coherence, with probabilities for passing through both slits. Yet, upon measurement or interaction with the environment, decoherence occurs, and the photon appears to have chosen a particular path, reflecting a classical particle-like behaviour.
Quantum decoherence helps to clarify why quantum weirdness doesn't manifest in the macroscopic world we live in.
The Role of Decoherence in Quantum Mechanics
Decoherence plays a pivotal role in quantum mechanics by providing a natural bridge between the quantum and classical realms. It's through this process that the peculiarities of quantum mechanics, such as superposition and entanglement, are reconciled with our everyday experiences.Key implications of quantum decoherence in quantum mechanics include:
- Elimination of superpositions: It effectively filters out superpositions, leading to a state where quantum systems behave more predictably, similar to classical particles.
- Deciphering classicality: It offers a mechanism through which the classical world emerges from quantum mechanics. Macroscopic objects don't show quantum behaviour because of rapid decoherence.
Diving deeper, decoherence is not just a theoretical construct but has practical implications in areas such as quantum computing and cryptography. Quantum computers, for example, rely on maintaining coherence to perform calculations that classical computers find intractable. Decoherence presents a significant challenge in this field, as it can lead to errors and loss of quantum computational power.In quantum cryptography, decoherence can potentially be used to detect eavesdropping. Since any measurement or interaction leading to decoherence can alter the state of quantum systems, it can reveal the presence of an interceptor in quantum key distribution processes.
Quantum Decoherence Examples
Understanding quantum decoherence through real-world examples provides a tangible way to grasp how quantum mechanics scales to the classical physics we observe around us. Here, you'll explore instances where the abstract concept of quantum decoherence manifests in practical, everyday scenarios and advanced technological applications.
Observing Quantum Decoherence in Real-World Scenarios
Quantum decoherence is a concept that might seem distant from everyday experiences due to its roots in quantum mechanics. However, this phenomenon can be observed and has implications for real-world scenarios, enhancing our understanding of both the micro and macro cosmos.Examples include the precision of atomic clocks and the functioning of MRI machines. Both rely on quantum mechanics principles, where decoherence must be carefully managed to ensure accurate and reliable results.
The process of quantum decoherence helps explain why we do not observe quantum behaviour, like superposition, in our everyday, macroscopic world.
Practical Examples of Quantum Decoherence
The principles of quantum decoherence play a pivotal role in the design and development of quantum technologies, particularly in quantum computing and encryption.For instance, in quantum computing, qubits (quantum bits) are susceptible to decoherence. This contributes to computational errors. Engineers must devise ways to isolate qubits from their environment or correct for decoherence effects to preserve their superposition and entanglement states.In the field of quantum cryptography, the inherent sensitivity of quantum states to decoherence is utilised for secure communication. Any attempt to intercept a quantum key distribution would induce decoherence, thus alerting the communicating parties of the intrusion.
Consider a simple quantum computing operation where two qubits in a superposition state are entangled. If one qubit interacts with the environment, causing decoherence, the entangled pair's superposition collapses, affecting computational outcomes:
if (qubit1.entangledWith(qubit2)) { qubit1.observe(); // Simulates interaction with the environment // The entanglement collapses due to decoherence }This code example simplifies how decoherence can disrupt quantum computations by collapsing superpositions and entanglement.
In quantum computing, the challenge of decoherence extends to error correction schemes. These strategies, vital for realising practical, scalable quantum computers, involve encoding information across multiple qubits. Through clever arrangements, known as quantum error-correcting codes, the effects of decoherence can be detected and corrected. This requires sophisticated manipulation of quantum states that not only shield against the environment but also enable recovering the original quantum information despite decoherence.
The Impact of Quantum Decoherence on Theoretical and Mathematical Physics
The concept of quantum decoherence provides a profound insight into the fundamental aspects of theoretical and mathematical physics. It not only bridges the gap between quantum mechanics and classical physics but also plays a pivotal role in the understanding and development of quantum technologies.
Quantum Decoherence and Its Significance in Physics
Quantum decoherence addresses one of the most perplexing questions in physics: how does the probabilistic nature of quantum mechanics give rise to the deterministic world observed at the macroscopic level? By explaining the mechanics behind the loss of coherence in quantum systems when interacting with their environment, quantum decoherence has revolutionised our understanding of the quantum-to-classical transition.This process is essential for explaining why superposition, a fundamental aspect of quantum mechanics where particles exist in multiple states simultaneously, seems to 'collapse' into a single state upon observation or interaction.
Quantum Decoherence is formally defined as the process by which coherence, the property of particles to exist in superpositions of states, is lost due to the interaction of a quantum system with its environment. This interaction leads to a dispersion of phases between the components of the superposition, making the quantum behaviour less apparent.
The transition from a coherent to a decohered state is not a physical 'collapse' of the wave function but rather a redistribution of probabilities.
How Quantum Decoherence Shapes Our Understanding of the Quantum World
Quantum decoherence has profound implications for how we understand the quantum world and its relationship with the observable classical world. This process helps to clarify why quantum phenomena, such as entanglement and superpositions, do not have direct analogs in our everyday experiences.Decoherence explains the emergence of classical properties through the degradation of quantum properties when quantum systems become entangled with their environments. This sheds light on the nature of measurement in quantum mechanics and the emergence of 'classical' objects in a fundamentally quantum universe.
An example of quantum decoherence can be seen in the double-slit experiment with electrons. When the electrons are not observed, they display wave-like behaviour, creating an interference pattern on a detector screen, indicative of their being in a superposition of paths. However, when their path is observed (and they effectively interact with the environment), the interference pattern disappears, and they behave more like classical particles, with each electron going through one slit or the other.
From a theoretical perspective, the significance of quantum decoherence extends beyond merely explaining the quantum-classical transition. It influences the foundation of quantum mechanics and has implications for the many-worlds interpretation, where decoherence plays a critical role in branching quantum probabilities into distinct 'worlds' or realities. Furthermore, in mathematical physics, decoherence is modelled using complex Hilbert space mathematics and density matrices to describe the statistical mixtures of states resulting from the interaction with the environment.Understanding these mathematical models provides insights into possible strategies to mitigate decoherence in quantum computing, where maintaining coherence is paramount for computational power.
Quantum Decoherence - Key takeaways
- Quantum Decoherence: The process of transition in which a quantum system loses its coherent superposition state and appears to adopt definite, classical states due to interaction with the environment.
- Decoherence and Measurement: Quantum decoherence does not solve the measurement problem but explains the emergence of classical realities from quantum probabilities.
- Coherence vs Decoherence: Quantum coherence refers to particles in a superposition of all states simultaneously. Decoherence occurs when interaction with the environment disperses this superposition, leading to observable classical outcomes.
- Practical Implications: Quantum decoherence is crucial for technologies such as quantum computing, where it poses challenges by causing errors, and in quantum cryptography, where it can detect eavesdropping.
- Theoretical Importance: Decoherence helps explain the transition from quantum to classical mechanics, influencing theoretical models and providing understanding for mitigating decoherence in quantum computing.
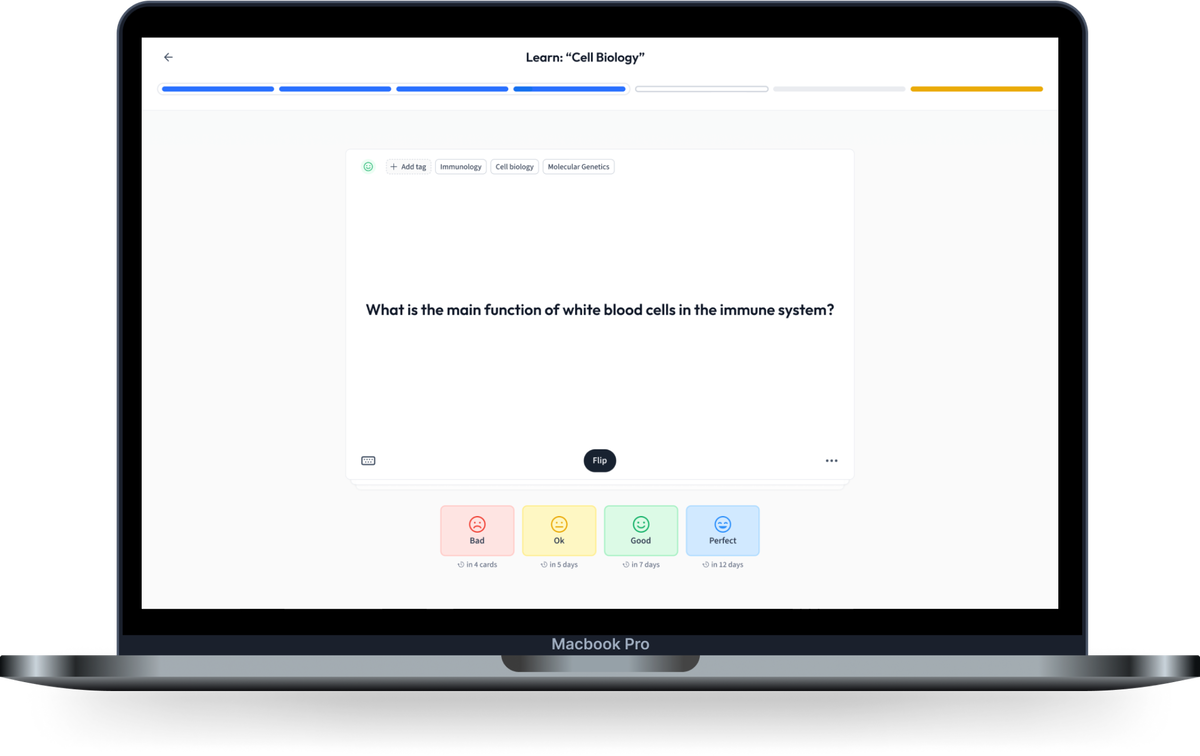
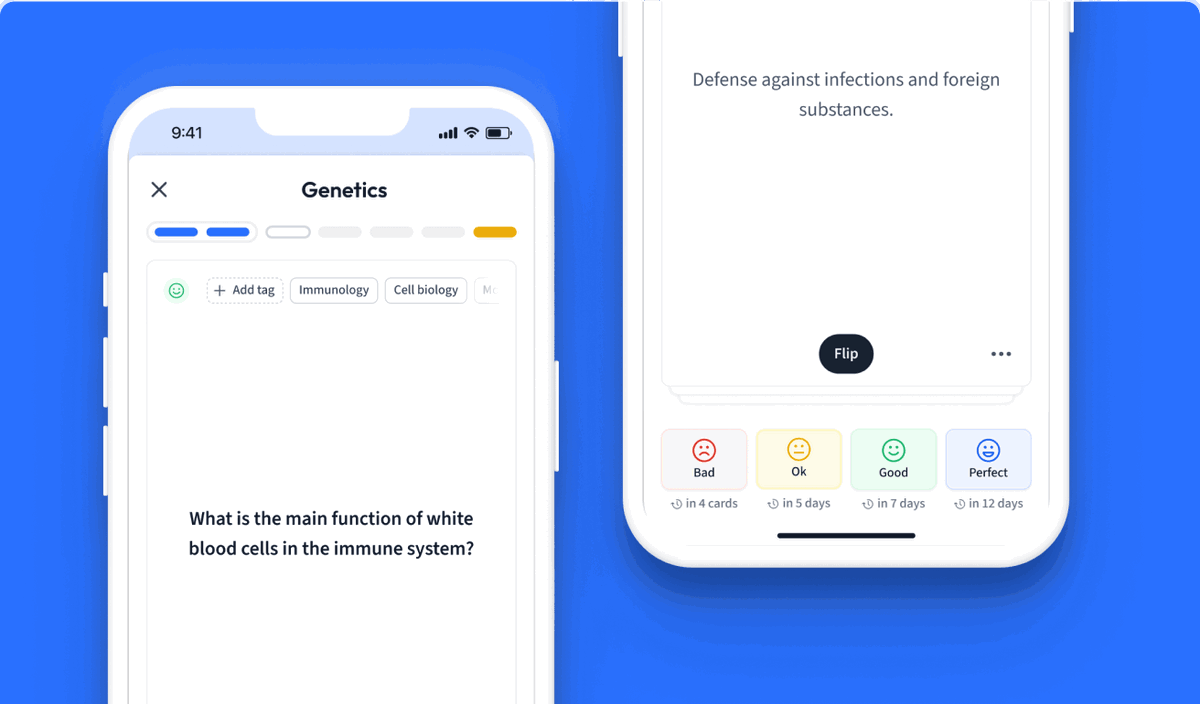
Learn with 12 Quantum Decoherence flashcards in the free StudySmarter app
Already have an account? Log in
Frequently Asked Questions about Quantum Decoherence
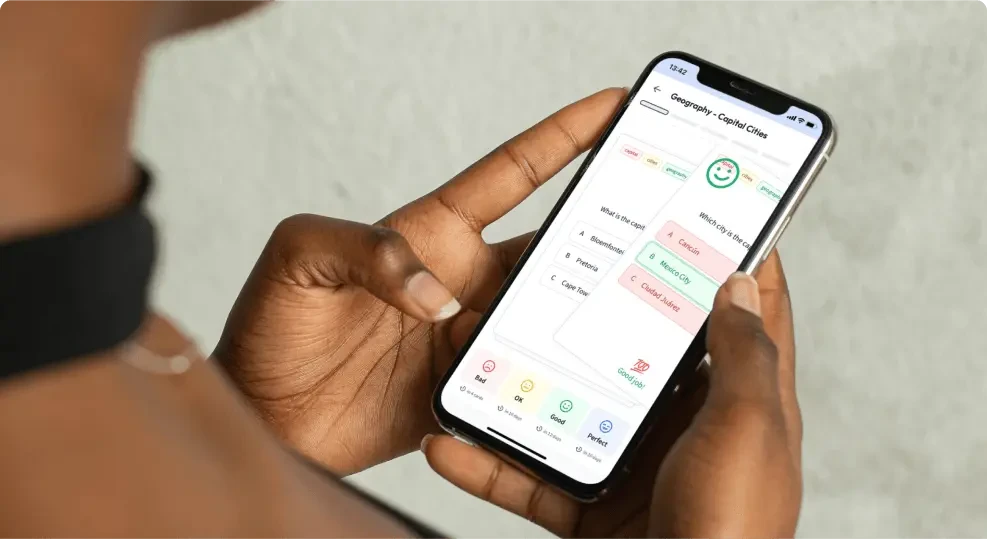
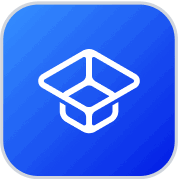
About StudySmarter
StudySmarter is a globally recognized educational technology company, offering a holistic learning platform designed for students of all ages and educational levels. Our platform provides learning support for a wide range of subjects, including STEM, Social Sciences, and Languages and also helps students to successfully master various tests and exams worldwide, such as GCSE, A Level, SAT, ACT, Abitur, and more. We offer an extensive library of learning materials, including interactive flashcards, comprehensive textbook solutions, and detailed explanations. The cutting-edge technology and tools we provide help students create their own learning materials. StudySmarter’s content is not only expert-verified but also regularly updated to ensure accuracy and relevance.
Learn more