Jump to a key chapter
Introduction to Advanced Genetics
Advanced Genetics explores the intricate complexities of the genetic code and the technologies that allow you to understand and manipulate it. It provides insights into how genes function and interact, leading to advancements in medicine, agriculture, and various fields.
Understanding the Genetic Code
The genetic code is a set of rules used by living cells to translate information encoded in genetic material (DNA or RNA) into proteins. Proteins are essential for the structure, function, and regulation of the body's cells, tissues, and organs. The universal nature of the genetic code is a key area of study in advanced genetics.
Genetic Code: The genetic code consists of nucleotide triplets called codons, each specifying a particular amino acid. This mechanism is universal across almost all organisms.
Consider a string of DNA with the sequence 5'-ATG-GGC-TAA-3'. Here, ATG is the start codon that specifies the amino acid methionine. The sequence GGC corresponds to glycine, and TAA is a stop codon signaling the end of protein synthesis.
Beyond the basic codon to amino acid translation, there are exceptions and variations known as genetic code anomalies. These include cases like non-standard amino acids such as selenocysteine and pyrrolysine which are incorporated into proteins via unique recognition mechanisms.
Not all genes directly code for proteins. Some genes are involved in regulatory or structural roles, producing RNA molecules like tRNA and rRNA.
Role of DNA Sequencing
DNA sequencing is a technology that allows you to determine the precise order of nucleotides within a DNA molecule. Understanding the sequence gives insights into genetic makeup, disease susceptibility, and more. This is crucial for advanced genetics as it uncovers functions and malfunctions within the genome.
DNA Sequencing: A laboratory technique used to determine the sequence of bases (adenine, guanine, cytosine, and thymine) in a DNA molecule.
- Sanger Sequencing: First developed DNA sequencing method that helps in decoding short DNA sequences.
- Next-Generation Sequencing (NGS): A modern high-throughput method that allows rapid sequencing of large stretches of DNA.
- Whole Genome Sequencing: Provides a comprehensive view of an individual's entire genetic makeup.
Through DNA sequencing, researchers were able to map the entire human genome. This monumental achievement has paved the way for personalized medicine, where treatments can be tailored to an individual's genetic profile.
The data obtained from DNA sequencing can also reveal epigenetic modifications, which do not change the DNA sequence but affect gene expression. These modifications can be influenced by external factors like diet and environment, playing a role in health and disease.
Exploring Genetic Variation
Genetic variation refers to the differences in DNA sequences among individuals within a population. These variations are crucial for the survival and adaptation of species over time. By examining genetic variation, you can gain insights into the biological diversity that fuels evolution and ecological changes.
Causes of Genetic Variation
Genetic variation arises due to several mechanisms. Understanding these causes is essential to grasp how diversity is maintained in natural populations.
Mutation: A random change in a DNA sequence that can lead to new genetic variants. Mutations can be beneficial, neutral, or harmful depending on their impact on an organism's fitness.
- Gene Flow: The transfer of genetic material between separate populations, increasing genetic diversity overall.
- Genetic Recombination: During sexual reproduction, genes are shuffled through processes like crossing over during meiosis, leading to new combinations.
An example of genetic variation due to recombination can be seen in pea plants where different combinations of alleles lead to variation in traits such as flower color and seed shape.
Sometimes genetic drift causes significant variation in smaller populations. This random fluctuation in allele frequencies is more pronounced in small groups and can lead to the fixation or loss of alleles irrespective of their utility to the organism.
Environmental factors like radiation and chemicals can increase the rate of mutations, contributing to genetic variation.
Impacts of Genetic Variation on Populations
Genetic variation is a catalyst for evolution and has critical implications for species survival. Different levels of genetic diversity can influence how populations respond to environmental pressures and changes.
Natural Selection: A process where organisms better adapted to their environment tend to survive and reproduce more than those less adapted.
Impact | Description |
Adaptation | Populations with high genetic diversity are more adaptable to changing environments. |
Survival | Genetic variation can make populations more resistant to diseases. |
The peppered moth is a classic example of natural selection. During the Industrial Revolution, darker moths became more prevalent in polluted areas because their coloring provided better camouflage against predators.
Genetic variation also plays a role in maintaining ecosystem stability. Populations with diverse genetic backgrounds are better at fulfilling different ecological roles, thus contributing to the health and robustness of an ecosystem.
Advanced Genetics: Genetic Disorders
In the realm of advanced genetics, understanding genetic disorders is crucial. These conditions are caused by abnormalities in an individual's DNA and can have wide-ranging effects on health and development. Genetic disorders are typically inherited, but some arise from environmental factors or random mutations.
Common Genetic Disorders
There are numerous genetic disorders, each affecting the body in unique ways. Some of the most common genetic disorders include:
Sickle Cell Anemia: This genetic condition results from a mutation in the hemoglobin gene, leading to the production of abnormally shaped red blood cells. These sickle-shaped cells can cause blockages in blood vessels and lead to severe pain and complications.
- Cystic Fibrosis: A disorder in which the CFTR gene mutation causes severe respiratory and digestive problems.
- Down Syndrome: Caused by the presence of an extra copy of chromosome 21, affecting physical and cognitive development.
- Huntington's Disease: A progressive brain disorder that affects movement, mood, and cognitive functioning due to mutations in the HTT gene.
It is fascinating to note that some disorders, like Huntington's disease, show symptoms later in life. This means individuals may unknowingly pass the disorder to the next generation before exhibiting any signs themselves. Such diseases raise important ethical and medical considerations in genetic counseling and testing.
Genetic Disorders and Inheritance Patterns
Understanding how genetic disorders are inherited enables you to better predict and manage these conditions within families. The inheritance patterns of genetic disorders can vary:
Autosomal Dominant: Only one mutated copy of the gene is needed for a person to be affected by the disorder. An example is Marfan syndrome, where individuals have a 50% chance of passing the mutated gene to their offspring.
- Autosomal Recessive: Two copies of the mutated gene are needed for the inheritance of the disorder. Examples include cystic fibrosis and sickle cell anemia.
- X-linked Inheritance: Disorders are associated with genes found on the X chromosome. Hemophilia, commonly seen in males, is one such disorder.
Not all genetic disorders have clear inheritance patterns due to the influence of multiple genes or environmental factors, which can complicate predictions.
With advances in genetic testing and biotechnology, it's now possible to detect potential genetic disorders even before symptoms appear. This opens doors to preventive healthcare strategies, but also poses ethical challenges related to privacy and genetic discrimination.
Genetic Engineering in Advanced Genetics
Genetic engineering is a pivotal aspect of advanced genetics, involving the direct manipulation of DNA to alter an organism's characteristics. By modifying genetic material, scientists can achieve outcomes not possible through traditional breeding methods. This powerful technology plays a crucial role in medicine, agriculture, and various industries.
Techniques in Genetic Engineering
Several techniques are utilized in genetic engineering to precisely alter genetic sequences. These methods enable precise, targeted changes in the DNA of organisms.
- CRISPR-Cas9: A revolutionary tool enabling highly accurate and efficient gene editing. It works by targeting specific DNA sequences and inducing breaks that allow for modifications.
- Gene Cloning: Involves copying specific genes to produce identical duplicates, often used in research and medicine.
- Recombinant DNA Technology: Combines DNA from different organisms, enabling the creation of genetically modified organisms (GMOs).
CRISPR-Cas9: A powerful, precise genome-editing method that allows scientists to cut DNA at desired locations, enabling the addition or removal of genetic material.
An example of genetic engineering is the development of Bt crops. These are genetically modified to express Bacillus thuringiensis toxin, providing resistance against certain pests and reducing the need for chemical pesticides.
One intriguing application of CRISPR is in the potential treatment of genetic disorders. Scientists are exploring its use to correct faulty genes responsible for conditions such as cystic fibrosis and muscular dystrophy. However, ethical concerns and technical challenges remain crucial aspects of ongoing research.
Gene editing technologies continue to advance, offering the potential to not only treat but also prevent genetic diseases by targeting them at their source.
Applications of Genetic Engineering
The applications of genetic engineering are vast, impacting numerous fields. This technology extends beyond traditional boundaries, influencing healthcare, agriculture, and environmental conservation.
Field | Application |
Medicine | Production of human insulin through genetically engineered bacteria, offering a reliable and scalable source of this essential hormone for diabetes management. |
Agriculture | Development of genetically modified crops that exhibit enhanced resistance to pests, diseases, and environmental stresses. |
Environment | Bioremediation techniques using genetically engineered microorganisms to clean up oil spills and neutralize pollutants. |
Scientists are working on genetically engineered mosquitoes to reduce the spread of diseases such as malaria and dengue fever, showing how genetic engineering can address public health issues.
Beyond immediate applications, genetic engineering holds promise for sustainable development. By creating crops requiring fewer resources, incorporating stress resistance traits, it may offer solutions to food security challenges in an era of climate change. However, the deployment of such technologies must be balanced with ecological considerations and public ethics.
Mendelian Inheritance in Advanced Genetics
Mendelian inheritance refers to the set of primary principles associated with the transmission of genetic material, formulated by Gregor Mendel through his work on pea plants. These principles form the foundation of understanding how traits are passed from parents to offspring and are important for your study of advanced genetics.
Principles of Mendelian Inheritance
The fundamental principles of Mendelian inheritance include the law of segregation, the law of independent assortment, and the concept of dominant and recessive alleles. These guide how you understand and predict the inheritance patterns for different traits.
- Law of Segregation: Each individual has two alleles for a trait, which segregate during meiosis so that each gamete carries only one allele.
- Law of Independent Assortment: Alleles of different genes assort independently during gamete formation, leading to the potential for different combinations in offspring.
- Dominant and Recessive Alleles: Dominant alleles mask the expression of recessive alleles in heterozygous combinations.
In Mendel's experiments with pea plants, he observed that when crossing a homozygous dominant tall plant with a homozygous recessive short plant, all the offspring in the F1 generation were tall, showcasing the dominance of the tall allele.
These principles apply to traits controlled by single genes with clear dominant-recessive relationships, often seen in simple genetic traits.
While Mendel's laws provide a basic framework, modern genetics has identified exceptions and extensions to these laws, such as the discovery of linked genes. These genes may not assort independently due to their physical proximity on chromosomes, which was a significant addition beyond Mendel's initial observations.
Mendelian Inheritance vs Non-Mendelian Patterns
Non-Mendelian inheritance patterns include phenomena that do not follow Mendel's laws entirely. The identification of these patterns has expanded the understanding of genetic inheritance beyond Mendel's models. Key categories of these include:
Incomplete Dominance: In cases of incomplete dominance, the heterozygous phenotype is a blend of both homozygous phenotypes, as neither allele is completely dominant.
- Codominance: Both alleles in a heterozygote are fully expressed, leading to phenotypes that display characteristics of both alleles.
- Pleiotropy: A single gene influences multiple phenotypic traits.
- Polygenic Traits: Traits controlled by two or more genes, often showing a continuum of phenotypes, such as human skin color.
A classic example of codominance is the ABO blood group system, where alleles A and B are both equally expressed in individuals with blood type AB.
Epigenetic factors also contribute to non-Mendelian inheritance patterns. These involve changes that affect gene expression without altering the DNA sequence itself. Environmental factors, lifestyle, and nutrition can play significant roles in epigenetic modifications, influencing inheritance patterns in a way that Mendel's original models did not account for.
Non-Mendelian patterns can be complex and involve multiple genes and interactions, requiring careful analysis to understand their roles in trait inheritance.
Advanced Genetics - Key takeaways
- Advanced Genetics: Focuses on the complexities of the genetic code, genetic engineering, and technologies manipulating genes to advance fields like medicine and agriculture.
- Genetic Code: Composed of nucleotide triplets called codons that translate genetic material into proteins, essential for cellular function.
- DNA Sequencing: A technology to determine nucleotide sequences, essential for understanding genetic makeup and variations; key to personalized medicine and understanding genetic disorders.
- Genetic Variation: Differences in DNA sequences among individuals within a population, crucial for species adaptation and evolution; caused by mutations, gene flow, and recombination.
- Genetic Disorders: Abnormalities in DNA leading to health conditions, often inherited; significant in studying genetic inheritance, including Mendelian and non-Mendelian patterns.
- Mendelian Inheritance: Principles describing how traits are passed from parents to offspring, involving laws of segregation and independent assortment, with modern genetics expanding on these laws.
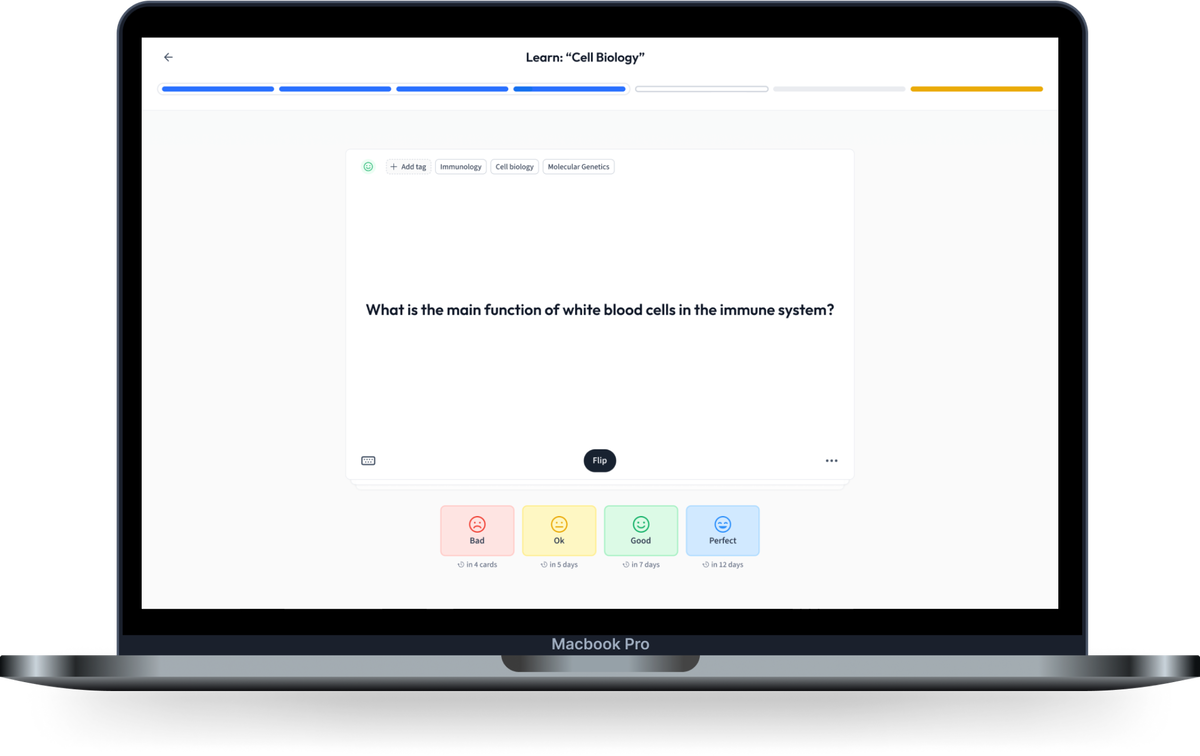
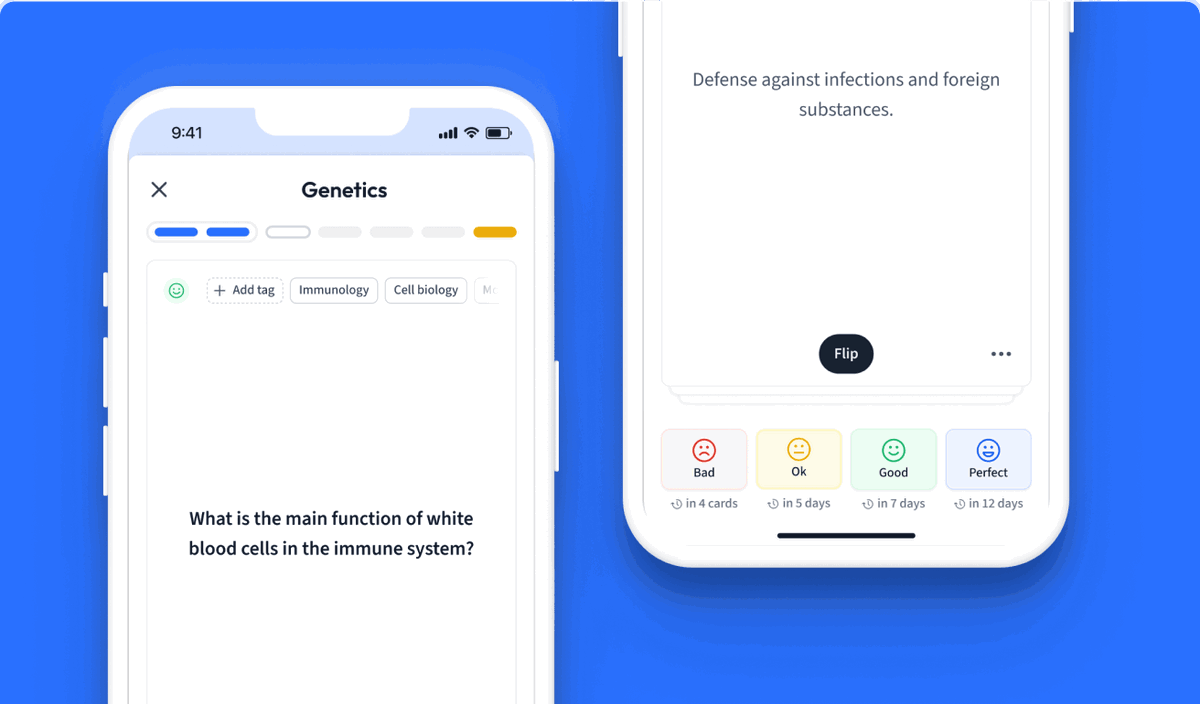
Learn with 10 Advanced Genetics flashcards in the free StudySmarter app
We have 14,000 flashcards about Dynamic Landscapes.
Already have an account? Log in
Frequently Asked Questions about Advanced Genetics
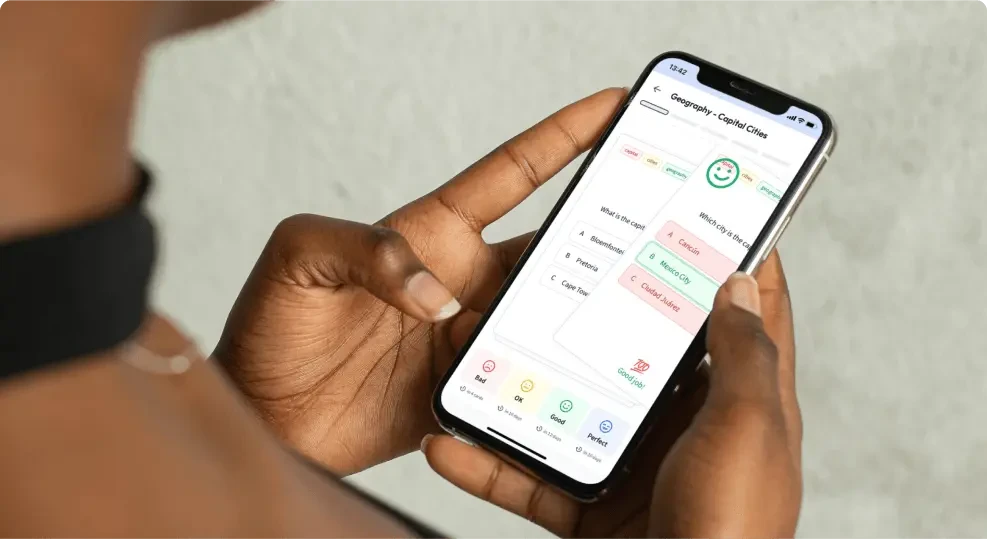
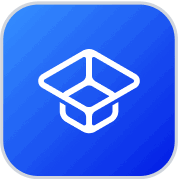
About StudySmarter
StudySmarter is a globally recognized educational technology company, offering a holistic learning platform designed for students of all ages and educational levels. Our platform provides learning support for a wide range of subjects, including STEM, Social Sciences, and Languages and also helps students to successfully master various tests and exams worldwide, such as GCSE, A Level, SAT, ACT, Abitur, and more. We offer an extensive library of learning materials, including interactive flashcards, comprehensive textbook solutions, and detailed explanations. The cutting-edge technology and tools we provide help students create their own learning materials. StudySmarter’s content is not only expert-verified but also regularly updated to ensure accuracy and relevance.
Learn more