Jump to a key chapter
Splicing Definition and Overview
In the field of molecular biology, splicing is a crucial process that occurs in the nucleus during gene expression. This involves the modification of the pre-mRNA (precursor messenger RNA) where non-coding regions, known as introns, are removed, and coding regions called exons are joined together. Proper splicing is essential for the production of functional proteins, which are crucial for various cellular functions. The process is carried out by a dynamic complex known as the spliceosome.
Splicing: The process of removing introns and joining exons in pre-mRNA to produce mature mRNA, which is then translated into proteins.
Mechanism of Splicing
The mechanism of splicing involves several steps, primarily guided by the spliceosome, a large and complex molecular machinery. The spliceosome facilitates the accurate removal of introns and the ligation of exons. Here's a brief overview of the steps involved:1. **Recognition and Binding:** The process begins with the recognition of specific nucleotide sequences at the exon-intron boundaries. The spliceosome identifies these sequences and binds to the pre-mRNA.2. **Branch Site Formation:** A specific point known as the branch site within the intron is activated. This is where a series of complex interactions occur to prepare the intron for removal.3. **Lariat Formation:** A loop-like structure, called a lariat, is formed when the branch site attacks the 5' end of the intron. This cuts the intron at the 5' splice site and attaches it to the branch site.4. **Exon Ligation:** After the lariat is formed, the exons flanking the intron are joined together. The intron is then excised as a lariat and later degraded, resulting in a mature mRNA ready for translation.The efficiency and accuracy of this process are vital, as errors can lead to dysfunctional proteins which may cause genetic disorders.
Let's consider an example of splicing in the human beta-globin gene, which undergoes splicing in the cell nucleus. This gene consists of three exons and two introns. During splicing, the two introns are removed, and the three exons are joined, forming a continuous sequence that codes for the beta-globin protein.
The spliceosome is among the most complex molecular machines in the cell, composed of five different RNA molecules and a range of proteins working in concert.
While most splicing events occur within the nucleus, there exists a form of splicing known as alternative splicing, which allows a single gene to produce multiple protein variants. Alternative splicing can occur in different ways, such as exon skipping, mutually exclusive exons, or alternative donor/acceptor sites. This process increases the diversity of proteins that can be synthesized and is a key factor in the complexity of higher organisms. In fact, it is estimated that over 95% of human genes undergo alternative splicing. Errors in this regulation can lead to diseases such as cancer and neurodegenerative disorders, highlighting the importance of precise splicing mechanisms.
RNA Splicing Process
RNA splicing is a critical step in the processing of pre-messenger RNA (pre-mRNA) in eukaryotic cells. During this process, non-coding sequences called introns are removed, and the remaining coding sequences known as exons are joined together. This results in the formation of mature messenger RNA (mRNA) that is ready for translation into a protein. The precision of this process is essential as it influences the genetic information relayed to form proteins.
RNA Splicing Techniques
There are several RNA splicing techniques that occur in cells, predominantly orchestrated by the spliceosome. Understanding these techniques can help in grasping how RNA processing impacts protein synthesis:
- Constitutive Splicing: This is the basic form of splicing where every intron in the pre-mRNA is removed, and all exons are combined in a linear sequence to form a single mRNA transcript.
- Alternative Splicing: This complex technique allows for the generation of multiple mRNA variants from the same pre-mRNA. Through mechanisms like exon skipping, mutually exclusive exons, or alternate 5’ or 3’ splice sites, a single gene can code for several proteins.
- Trans-splicing: Unlike the other techniques that involve a single pre-mRNA, trans-splicing occurs when exons from two different pre-mRNA molecules are joined together, forming a chimeric RNA.
Alternative Splicing: A regulatory mechanism by which different combinations of exons are joined, resulting in multiple mRNAs from the same gene.
Consider a gene with four exons labeled E1, E2, E3, and E4. Through alternative splicing, the pre-mRNA can be processed in various ways:1. **E1 + E2 + E3 + E4**2. **E1 + E3 + E4** (skipping exon E2)3. **E1 + E2 + E4** (skipping exon E3)
Alternative Splicing
Alternative splicing is a sophisticated mechanism that broadens the potential of gene expression in eukaryotic cells. By selectively assembling exons from a single pre-mRNA, this process allows a single gene to code for multiple proteins, thus enhancing protein diversity and regulating various biological functions.
Alternative Splicing Significance
The significance of alternative splicing is profound, as it impacts several critical biological and cellular processes. Here are some key points:
- Protein Diversity: By enabling a single gene to produce different protein isoforms, alternative splicing exponentially increases the protein repertoire of cells.
- Regulation of Gene Expression: Alternative splicing plays a crucial role in the regulation of gene expression and can influence cellular responses to external stimuli.
- Development and Differentiation: It is pivotal during development and differentiation, as it allows cells to produce specific proteins necessary at various stages.
A classic example of alternative splicing is the dscam gene in fruit flies (Drosophila melanogaster). This gene can potentially produce over 38,000 different protein isoforms due to extensive alternative splicing of its exons. This diversity is crucial for the fly's nervous system development.
It is estimated that over 95% of human genes undergo alternative splicing, highlighting its importance in mammalian biology.
In some cases, alternative splicing can be tissue-specific. For instance, the calcitonin gene undergoes different splicing events in the thyroid and neuronal tissues, resulting in different end products with distinct functions. In the thyroid, it produces calcitonin, while in neurons, it results in a different protein, CGRP (Calcitonin Gene-Related Peptide), highlighting the adaptive nature of alternative splicing. Errors in alternative splicing can lead to numerous disorders, including cystic fibrosis and certain types of cancer, underscoring the need for precise regulation of this process.
Gene Splicing
Gene splicing is a technique used in biotechnology and genetic engineering where DNA is cut and rejoined to alter the genetic makeup of organisms. This process involves the modification of genetic sequences to either remove or introduce specific genes, facilitating the study of gene function and the development of genetically modified organisms (GMOs). Gene splicing plays a crucial role in research, medical, and agricultural advancements.
Gene Splicing Methods
Several techniques are utilized in gene splicing, each with specific applications and benefits. These methods include:
- Restriction Enzymes: These are proteins that cut DNA at specific sequences, known as restriction sites. By using restriction enzymes, scientists can isolate specific DNA segments for further experimentation.
- Ligation: After cutting DNA with restriction enzymes, the DNA fragments can be joined or spliced together using an enzyme called DNA ligase. This enzyme helps form bonds between the DNA fragments, facilitating gene assembly or modification.
- Polymerase Chain Reaction (PCR): PCR is a method used to amplify specific DNA segments, creating numerous copies. It is used to prepare DNA for further splicing or cloning experiments.
- CRISPR-Cas9: This advanced method allows for precise gene editing by creating double-strand breaks at specific locations in the genome. The breaks are then repaired, allowing for the insertion, deletion, or modification of genes.
Restriction Enzymes: Proteins that recognize specific DNA sequences and cut the DNA at or near these sequences.
Imagine a scenario where you need to splice a gene responsible for drought resistance into a plant's genome. Using restriction enzymes, you can isolate the drought-resistant gene from its original source. Following this, DNA ligase would help incorporate this gene into the plant's DNA, resulting in a genetically modified plant with enhanced drought resistance.
CRISPR-Cas9 is often more efficient and precise compared to traditional splicing methods, due to its targeted approach in modifying genes.
The advent of CRISPR-Cas9 technology has revolutionized gene editing and splicing. Unlike traditional methods relying solely on restriction enzymes and ligases, CRISPR-Cas9 allows for highly specific and efficient genetic alterations. For example, by designing a guide RNA sequence that matches a target DNA sequence, CRISPR can pinpoint the exact location in the genome that needs editing. Paired with the Cas9 protein, which acts as molecular scissors, scientists can create precise double-stranded breaks. After these breaks are made, the cell’s natural repair mechanisms can be exploited to either introduce changes through non-homologous end joining (NHEJ) or to precisely modify the gene using homologous recombination (HR). This powerful method has vast implications for treating genetic diseases, improving agricultural crops, and understanding complex genetic networks. However, ethical considerations and potential off-target effects remain a topic of ongoing research and discussion.
splicing - Key takeaways
- Splicing is the process of removing introns and joining exons in pre-mRNA to produce mature mRNA, crucial for protein formation.
- The mechanism of splicing primarily involves the spliceosome, which ensures accurate removal of introns and ligation of exons.
- RNA splicing is a critical step in pre-mRNA processing, involving techniques like constitutive, alternative, and trans-splicing.
- Alternative splicing allows a single gene to produce multiple protein variants and is pivotal for protein diversity in eukaryotic cells.
- Gene splicing, a biotechnology technique, alters the genetic makeup by cutting and rejoining DNA sequences.
- CRISPR-Cas9 is an advanced gene splicing method offering precise gene editing capabilities by creating targeted genome modifications.
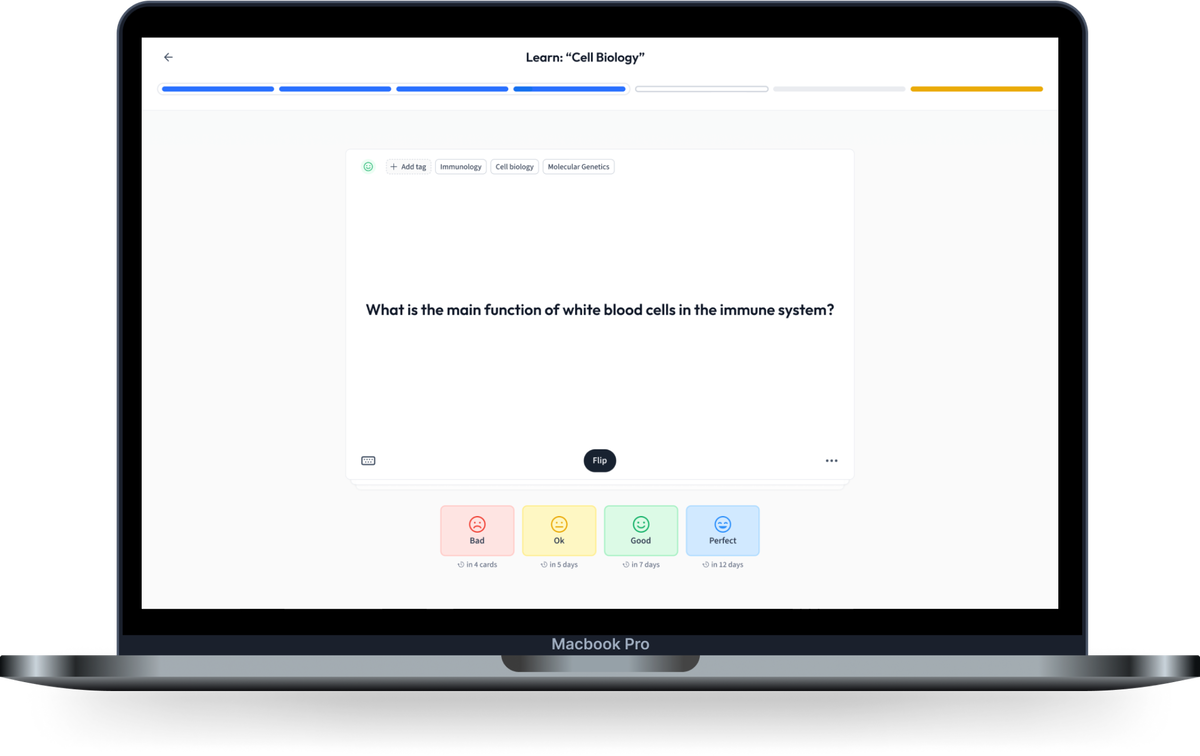
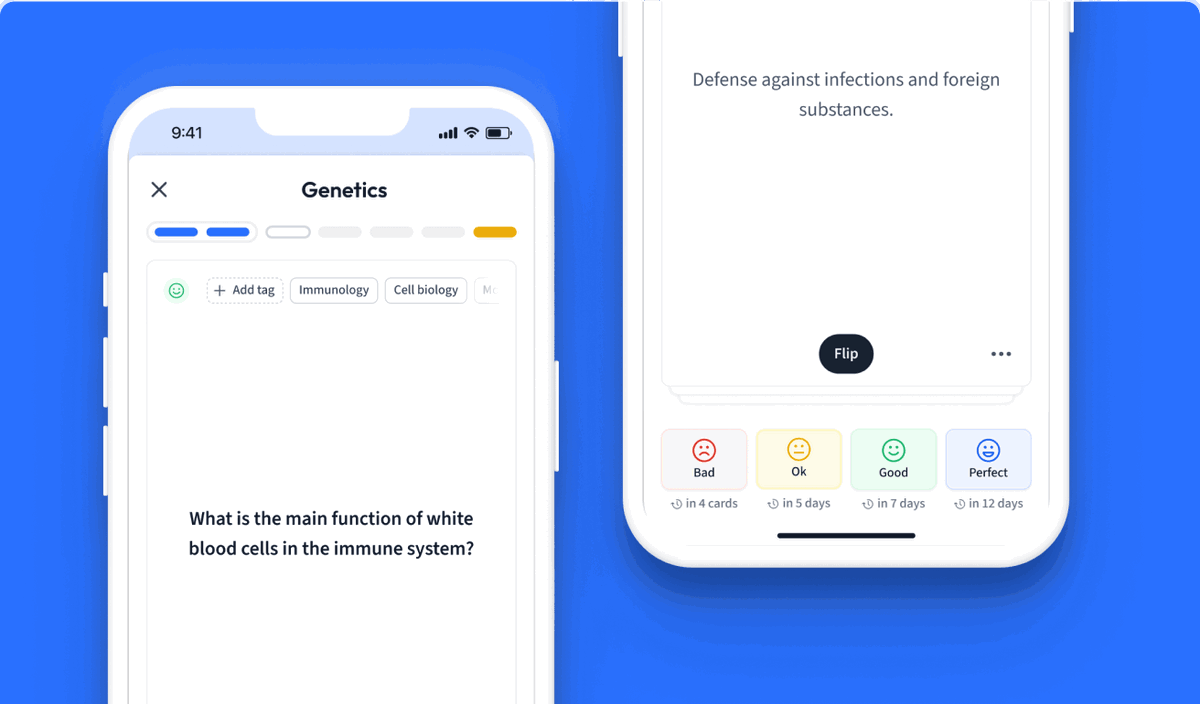
Learn with 12 splicing flashcards in the free StudySmarter app
Already have an account? Log in
Frequently Asked Questions about splicing
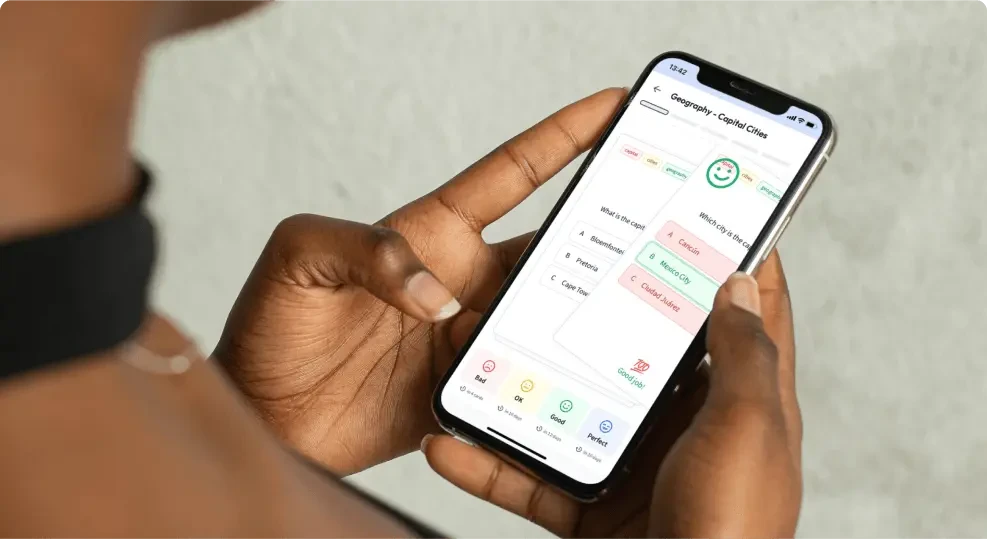
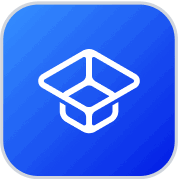
About StudySmarter
StudySmarter is a globally recognized educational technology company, offering a holistic learning platform designed for students of all ages and educational levels. Our platform provides learning support for a wide range of subjects, including STEM, Social Sciences, and Languages and also helps students to successfully master various tests and exams worldwide, such as GCSE, A Level, SAT, ACT, Abitur, and more. We offer an extensive library of learning materials, including interactive flashcards, comprehensive textbook solutions, and detailed explanations. The cutting-edge technology and tools we provide help students create their own learning materials. StudySmarter’s content is not only expert-verified but also regularly updated to ensure accuracy and relevance.
Learn more