Jump to a key chapter
Definition of Tissue Architecture
Tissue architecture is a fundamental concept in both biology and medicine. It encompasses the organization and arrangement of different tissue types in the body, contributing to their function and roles within the organism.
What is Tissue Architecture?
The term tissue architecture refers to the spatial arrangement and physical organization of cells and extracellular components in tissues. This organization is crucial for maintaining the functionality and health of organisms. The importance of tissue architecture lies in its ability to dictate how tissues perform specific functions, whether in muscle tissues enabling movement, or nerve tissues transmitting signals. Tissue architecture varies greatly between different tissues, adapting cells into structures that enable them to meet the demands of their specific environments. In an organism, tissue architecture involves complex interactions between cells and their surrounding extracellular matrix (ECM). The ECM provides structural and biochemical support to the surrounding cells, playing a key role in tissue morphology and behavior.
Extracellular Matrix (ECM) is a three-dimensional network of extracellular macromolecules such as collagen, enzymes, and glycoproteins that provide structural and biochemical support to cells.
Consider the architecture of epithelial tissue, which acts as a barrier covering the body and its organs. It has tightly packed cells with minimal ECM to form layers that protect and enclose organs.
The role of tissue architecture extends beyond simple structural support. In immune tissues, the specific configuration allows for efficient surveillance and response to pathogens. Disruptions in tissue architecture can lead to diseases, including cancer, where abnormal tissue organization can enhance tumor growth and metastasis. The study of tissue architecture also provides insights into developmental processes, aiding in understanding congenital disabilities and tissue regeneration.
Components of Tissue Architecture
Understanding the components of tissue architecture is essential for comprehending how tissues maintain their form and function. Here’s what you need to know about the primary components:
- Cells: The basic units of life form the bulk of tissues. Different cell types are adapted to perform specific roles within their respective tissues.
- Extracellular Matrix (ECM): A complex network that provides structural integrity and biochemical signals to the cells.
- Intercellular Junctions: These are connections between cells that ensure cohesion and communication. Tight junctions and gap junctions are examples.
- Basement Membrane: A specialized ECM layer providing a support base for epithelial and endothelial cells.
An imbalance in tissue architecture, such as an unhealthy ECM, may lead to diseases like fibrosis or cancer.
Techniques to Study Tissue Architecture
The study of tissue architecture is essential for understanding how tissues function and respond to various conditions. It involves several methodologies that provide insights into the intricate arrangements of cells and their extracellular environments.
Imaging Techniques in Tissue Architecture
Imaging techniques are vital tools in the study of tissue architecture as they allow visualization of the internal structures and arrangements within tissues. Here are some key imaging techniques used:
- Microscopy: This includes light, fluorescence, and electron microscopy, each offering varying levels of resolution to examine tissue samples.
- Magnetic Resonance Imaging (MRI): A non-invasive method providing detailed images of soft tissues, crucial for detecting abnormalities.
- Computed Tomography (CT): Utilized primarily for visualizing dense structures like bones, but also offers insights into soft tissue organization with the use of contrast agents.
Microscopy refers to the technique of using microscopes to view samples and objects that cannot be seen with the unaided eye.
Fluorescence microscopy is especially useful for tagging specific proteins within tissues, offering insights into the positioning and roles of different cellular components.
Role of Histology in Studying Tissue Architecture
Histology, the study of tissues at the microscopic level, is a fundamental method for examining tissue architecture. It provides detailed insights into the organization, structure, and function of cells and tissues.
- Staining Techniques: Histological studies often use special stains to highlight various tissue components. For example, hematoxylin and eosin (H&E) staining is widely used to differentiate cellular structures.
- Tissue Preparation: This involves fixing, embedding, sectioning, and staining the tissue, preparing it for microscopic examination.
In a typical histology lab, the use of H&E staining can differentiate between nuclei (stained blue by hematoxylin) and the cytoplasm and extracellular matrix (stained pink by eosin). This aids in the diagnosis of diseases like cancer, where tissue architecture often appears disrupted.
Histology doesn’t only offer insights into healthy tissue structure. It’s also crucial in pathology, where deviations from normal architecture can indicate disease. Advanced histological techniques, such as immunohistochemistry, allow for the detection of specific antigens in tissues by using antibodies tagged with a visible marker, thus offering more precise diagnostic capabilities.
Advanced Methods for Analyzing Tissue Architecture
Beyond traditional microscopy and histology, advanced methods have been developed to offer more comprehensive insights into tissue architecture. These methods include:
- 3D Bioprinting: This technique enables the creation of three-dimensional tissue models to study complex tissue interactions in a controlled manner.
- Spatial Transcriptomics: A cutting-edge method that combines spatial information with gene expression profiling within tissues.
- Confocal Microscopy: Offers enhanced imaging detail and depth within thick tissues, allowing visualization just beneath the surface.
Exploring Tissue Architecture Using Spatial Transcriptomics
Spatial transcriptomics is an innovative technique that combines molecular profiling with spatial information from tissues. This advanced technology allows scientists to map and understand gene expression patterns within the context of tissue architecture. By integrating spatial context with gene analytics, spatial transcriptomics uncovers new insights into the complex organization and function of biological tissues.This approach provides a comprehensive view of the cellular environment, revealing how cells interact within their native contexts. The ability to visualize these interactions within the spatial domain significantly enhances our understanding of tissue development and disease progression.
Spatial Transcriptomics: An Overview
Spatial transcriptomics represents a cutting-edge method used to study gene expression within tissues while maintaining information about the exact location of those genes. This method is an evolution beyond traditional techniques that lose spatial context by dissociating cells from their environments. Using spatial transcriptomics involves several key steps:
- Tissue Preparation: Tissues are carefully cryo-sectioned and placed on specialized slides.
- Slide Processing: These slides then undergo in situ reactions to capture RNA, maintaining spatial information across the tissue.
- Sequencing: Captured RNA is sequenced, and data analytics provide a map of gene expression patterns.
Gene Expression is the process by which information from a gene is used in the synthesis of a functional gene product, often proteins, which perform essential cellular functions.
Spatial transcriptomics can also identify rare cell types and states that might be missed by traditional bulk analysis methods.
Understanding the mechanisms behind spatial transcriptomics offers fascinating possibilities in both research and clinical medicine. This method allows researchers to observe tumor microenvironments' behavior and how certain gene expressions contribute to diseases. In neuroscience, it helps in exploring the distinct yet interconnected regions of the brain by mapping out active transcripts within specific neural pathways. Additionally, its application in plant sciences is providing insights into how plants respond to environmental changes at a molecular level. These diverse applications highlight the versatile and transformative impact spatial transcriptomics is having across multiple fields of biological research.
Applications of Spatial Transcriptomics in Tissue Research
Spatial transcriptomics is rapidly advancing the field of tissue research, providing valuable insights into how tissues function at a molecular level while retaining spatial context. Here are some applications of spatial transcriptomics in tissue research:
- Cancer Research: By mapping gene expression within tumor tissues, researchers can identify regulatory pathways and understand tumor heterogeneity, guiding targeted therapy strategies.
- Neuroscience: In brain tissues, spatial transcriptomics reveals how different brain regions communicate and function, potentially advancing our understanding of neurodevelopmental and degenerative diseases.
- Developmental Biology: Understanding how gene expression changes during various stages of development helps elucidate how complex tissues and organs form.
- Regenerative Medicine: Mapping gene expression in tissues aids in designing approaches for tissue repair and regeneration, important for tackling diseases and injuries that impair normal functions.
In a study using spatial transcriptomics, researchers were able to identify specific gene patterns linked to aggressive tumor regions in breast cancer tissue, allowing for a more nuanced understanding of cancer progression.
Spatial transcriptomics continues to evolve with the development of higher-resolution techniques, which allow even more detailed tissue mapping.
Unsupervised Discovery of Tissue Architecture in Multiplexed Imaging
Advancements in imaging technologies have opened new avenues for studying tissue architecture. Among these, multiplexed imaging stands out as a sophisticated approach, allowing researchers to explore intricate tissue structures through simultaneous visualization of multiple biological markers. This technique enhances the understanding of the spatial organization and heterogeneity of tissues, key factors in diagnosis and research of diseases.
Understanding Multiplexed Imaging
Multiplexed imaging is a powerful method used to visualize multiple components within a single tissue sample, such as proteins, nucleic acids, and other molecules. This technique uses different fluorescent markers or labels that bind specifically to target molecules, providing a comprehensive view of tissue architecture.The process of multiplexed imaging typically involves:
- Sample Preparation: Tissues are carefully fixed and sectioned to preserve their structural integrity.
- Labeling: Multiple fluorescent dyes or antibodies are applied to the tissue to bind to specific biomarkers.
- Image Acquisition: High-resolution imaging systems capture images for each fluorescent marker, resulting in a multi-layered dataset.
Example: In cancer research, multiplexed imaging can be used to simultaneously visualize tumor cells, immune cells, and stromal components within a tumor microenvironment, revealing interactions that could influence therapeutic responses.
Multiplexed Imaging is a process that enables the concurrent detection and analysis of multiple biological markers within a single tissue sample.
Multiplexed imaging can be further enhanced with computational tools to analyze complex datasets and extract biologically relevant information.
By integrating multiplexed imaging with machine learning, researchers can uncover patterns and correlations that are not immediately visible through traditional analysis. Machine learning algorithms can automatically classify tissue types, identify abnormalities, and even predict disease progression based on the multilayered dataset obtained from multiplexed imaging. This integration leads to a deeper understanding of tissue architecture and equips researchers with powerful tools for precision medicine. Looking ahead, the continued development of imaging technologies will likely expand these capabilities, offering even more precise and targeted insights into the architecture and pathology of tissues.
Unsupervised Techniques in Tissue Architecture Analysis
Unsupervised learning plays a pivotal role in the analysis of tissue architecture captured through multiplexed imaging. These techniques, which include clustering and dimensionality reduction methods, allow researchers to discover hidden patterns within data without predefined labels or categories.Key unsupervised techniques include:
- K-Means Clustering: This method groups data points into k clusters based on feature similarity, useful for identifying distinct cell types or states within complex tissues.
- Hierarchical Clustering: This approach builds a tree-like model of related clusters, offering insights into the hierarchical organization of tissue components.
- Principal Component Analysis (PCA): A dimensionality reduction technique that simplifies data complexity, highlighting key components driving variance in the dataset.
Unsupervised Learning is a type of machine learning that identifies patterns within data without the need for labeled outcomes or supervision from an external data source.
Example: In a study using unsupervised clustering, researchers identified unique immune cell populations in lung tissue that correlated with different disease states, improving understanding of immune responses in respiratory diseases.
While unsupervised techniques offer great potential, combining them with supervised learning methods can enhance the robustness and interpretability of outcomes.
Importance of Tissue Architecture in Disease Diagnosis
Tissue architecture plays a crucial role in disease diagnosis, providing insights into how tissues function and interact within the body. Its organization and patterns are essential for understanding the underlying mechanisms of various diseases.
Impact on Cancer Detection
The study of tissue architecture is integral to cancer detection. Cancerous tissues often display distinct architectural differences compared to healthy tissues, allowing pathologists to identify malignancies.Cancer diagnosis typically involves:
- Histological Analysis: Examining tissue samples under a microscope to observe abnormalities such as disorganized cell structures.
- Biomarker Identification: Detecting specific genetic or protein markers that indicate malignancy.
- Imaging Techniques: Utilizing MRI, CT, and PET scans to visualize tissue architecture non-invasively.
Histological Analysis involves the study of the microscopic structure of tissues, often used to detect cancerous changes.
Example: In breast cancer diagnosis, histological analysis often reveals disrupted tissue architecture, such as irregular gland formation and cell proliferation, distinguishing malignant from benign tissues.
Advancements in understanding tissue architecture have led to the development of personalized cancer treatments. By analyzing the specific structural alterations in tumor tissue, oncologists can tailor treatment strategies to target the distinctive features of a patient's cancer. Moreover, emerging technologies like AI-powered image analysis are enhancing the precision of cancer diagnosis by providing more accurate interpretations of tissue architecture. This specific approach not only improves early detection but also helps in predicting patient outcomes and monitoring treatment effectiveness.
Research is ongoing to explore how shifts in tissue architecture may signal the presence of early-stage cancer, potentially improving early detection rates.
Role in Understanding Genetic Disorders
Tissue architecture is vital in understanding genetic disorders, as the arrangement of cells and extracellular components affects how genetic abnormalities manifest within tissues.In genetic disorders, the changes in tissue architecture can include:
- Abnormal Cell Proliferation: Leading to atypical tissue growth, as seen in some developmental disorders.
- Disrupted Cellular Organization: Causing impaired tissue functions, as observed in connective tissue diseases.
- Altered Extracellular Matrix (ECM): Impacting structural integrity and intercellular signaling, crucial in disorders like Marfan syndrome.
Example: In cystic fibrosis, changes in the architecture of lung tissues—such as thickened mucus-lining and inflamed airways—aid in understanding and diagnosing the disorder.
Extracellular Matrix (ECM) is a network of macromolecules that provides structural and biochemical support to surrounding cells, crucial for tissue integrity.
An in-depth examination of tissue architecture in genetic disorders offers insights into potential therapeutic targets. Abnormalities in the cellular layout or ECM composition can be indicative of specific genetic mutations, allowing for targeted genetic therapies. Technologies such as CRISPR can potentially correct gene mutations that lead to architectural disruptions. Furthermore, 3D tissue models are being developed to simulate the impact of specific genetic changes on tissue architecture before implementing corrective therapies. These models serve as valuable tools in predicting the efficacy and safety of potential treatments.
Understanding the link between genetic mutations and tissue architecture can lead to the development of biomarkers for early disease detection and intervention.
Examples of Tissue Architecture in Human Organs
Tissue architecture varies widely across different organs in the body, each adapted for specific functions. The unique arrangement of cells and their extracellular components in each organ supports their distinct roles in maintaining health and homeostasis.
Tissue Architecture in the Heart
The heart, a muscular organ, consists of specialized tissue known as myocardium, essential for its pump function. The heart's tissue architecture is crucial for its ability to contract and propel blood efficiently through the circulatory system.Key features include:
- Cardiac Muscle Cells: Also known as cardiomyocytes, these cells are striated and interconnected by intercalated disks, allowing synchronized contractions.
- Intercalated Disks: Specialized connections that facilitate electrical impulses to ensure heartbeats are rhythmic and coordinated.
- Fibrous Skeleton: Provides structural support and separates different chambers of the heart, holding them in place and maintaining proper valve function.
Example: The presence of gap junctions within the intercalated disks enables the rapid transmission of electrical signals, crucial for the heart's synchronized contraction.
The heart's tissue architecture also includes abundant capillary networks to sustain its high metabolic demands. The close association between cardiomyocytes and capillaries ensures efficient oxygen and nutrient delivery, critical for energy production. Additionally, the heart's architecture changes in response to physiological demands, such as exercise, where muscle thickness may increase to accommodate enhanced output.
Tissue Architecture in the Liver
The liver is a complex organ with a unique tissue architecture that supports its diverse metabolic, detoxification, and synthetic functions. Liver tissue is composed of specialized structures that facilitate these processes.Core structural elements include:
- Hepatocytes: The main functional cells of the liver, organized in plates known as hepatic cords that facilitate surface area for metabolic exchange.
- Sinusoids: Highly permeable blood vessels that lie between hepatic plates. These allow easy access to blood for hepatocytes, facilitating nutrient exchange and detoxification.
- Biliary Canaliculi: Small ducts between hepatocytes where bile is secreted and transported to larger bile ducts.
Example: Hepatocytes have a high number of mitochondria, supporting the liver's role in metabolism and detoxification processes.
Liver architecture also enables its remarkable regenerative capacity. In response to acute damage, such as partial hepatectomy, remaining hepatocytes rapidly proliferate to restore liver mass. This regenerative capability is guided by a tightly regulated system of growth factors and cytokines, showcasing the liver's unique ability to maintain tissue integrity and function.
Tissue Architecture in the Brain
The brain is a highly specialized organ with a distinct tissue architecture that facilitates its role as the control center for the body. The brain's architecture reflects its complex functionality in processing and transmitting information.Primary components include:
- Neurons: The fundamental units of the brain responsible for transmitting information via electrical and chemical signals.
- Glial Cells: Supportive cells that aid in neuron function, supply nutrients, and maintain homeostasis.
- Gray Matter: Composed mainly of neuron cell bodies and is involved in muscle control and sensory perception.
- White Matter: Consists of myelinated axons connecting different brain regions, facilitating communication.
Example: The cerebral cortex is an example of gray matter, involved in functions like thought, memory, and decision-making.
Brain plasticity, or the ability to reorganize itself, is a unique feature facilitated by the dynamic nature of its tissue architecture.
tissue architecture - Key takeaways
- Definition of Tissue Architecture: It refers to the spatial arrangement and physical organization of cells and extracellular components in tissues, crucial for maintaining functionality and health.
- Techniques to Study Tissue Architecture: Microscopy, MRI, and CT are key imaging techniques; histology and spatial transcriptomics offer detailed insights into tissue organization.
- Exploring Tissue Architecture Using Spatial Transcriptomics: Spatial transcriptomics combines molecular profiling with spatial data to map gene expression within tissues, enhancing understanding of tissue development and disease progression.
- Unsupervised Discovery of Tissue Architecture in Multiplexed Imaging: Uses techniques like multiplexed imaging to explore complex tissue structures and integrating machine learning for deeper insights.
- Importance of Tissue Architecture in Disease Diagnosis: Abnormal tissue architecture is a key indicator in diagnosing diseases such as cancer, aiding in personalized treatment strategies.
- Examples of Tissue Architecture in Human Organs: Heart, liver, and brain have unique tissue architectures, each adapted for distinct functions like pumping blood, metabolism, and information processing.
Learn faster with the 12 flashcards about tissue architecture
Sign up for free to gain access to all our flashcards.
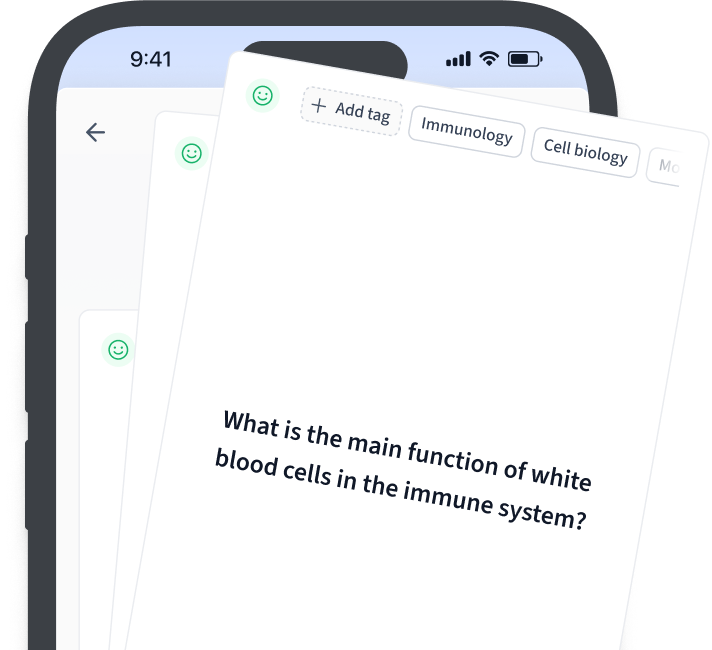
Frequently Asked Questions about tissue architecture
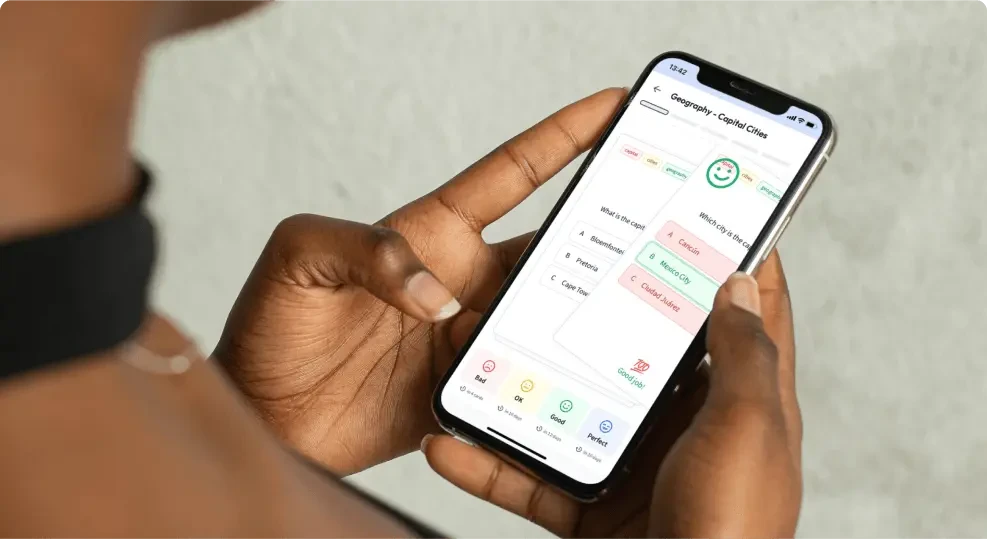
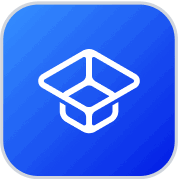
About StudySmarter
StudySmarter is a globally recognized educational technology company, offering a holistic learning platform designed for students of all ages and educational levels. Our platform provides learning support for a wide range of subjects, including STEM, Social Sciences, and Languages and also helps students to successfully master various tests and exams worldwide, such as GCSE, A Level, SAT, ACT, Abitur, and more. We offer an extensive library of learning materials, including interactive flashcards, comprehensive textbook solutions, and detailed explanations. The cutting-edge technology and tools we provide help students create their own learning materials. StudySmarter’s content is not only expert-verified but also regularly updated to ensure accuracy and relevance.
Learn more