Jump to a key chapter
Membrane Transport - Definition
Membrane transport is a fundamental concept in biology and medicine, crucial for maintaining cellular homeostasis and enabling various physiological functions.
What is Membrane Transport?
Membrane transport refers to the movement of substances across the cell membrane. This process is essential for the survival and function of cells, as it regulates the import and export of necessary nutrients, ions, and waste products.
- Passive Transport: Movement without the expenditure of energy, primarily influenced by concentration gradients.
- Active Transport: Movement requiring energy, often against a concentration gradient.
- Facilitated Transport: Assisted movement of substances via specific proteins.
Cell Membrane: A biological membrane separating the interior of cells from the outside environment, controlling the movement of substances in and out of the cell.
Types of Membrane Transport
There are several types of membrane transport mechanisms, each serving distinct purposes within the cellular environment.
- Simple Diffusion: The passive movement of molecules from a region of higher concentration to one of lower concentration.
- Osmosis: The diffusion of water molecules across a selectively permeable membrane.
- Primary Active Transport: An energy-consuming process that moves molecules across a membrane against their concentration gradient, typically using ATP.
- Secondary Active Transport: Uses energy from electrochemical gradients, not directly from ATP.
Consider a red blood cell in a hypertonic solution: water moves out of the cell by osmosis, causing the cell to shrink. This demonstrates the impact of membrane transport on cell structure and function.
Membrane transport can also be influenced by temperature and pressure, impacting the rate and direction of movement.
Initially, the study of membrane transport focused on simple systems. However, advances in technology have revealed the complexity of transport proteins and channels, revolutionizing our understanding. Current research delves into the molecular biology of transport mechanisms, examining protein structures and their roles in disease states.
Types of Membrane Transport
Understanding the different types of membrane transport is crucial for grasping how substances move in and out of cells. This knowledge can help you comprehend various biological processes and the role of cell membranes in maintaining homeostasis.
Passive Membrane Transport Processes Include
Passive transport is a method by which molecules transit across the cell membrane without the use of cellular energy. This process is driven by the concentration gradient, moving substances from areas of higher concentration to regions of lower concentration, thus maintaining equilibrium.
- Simple Diffusion: Allows small or uncharged molecules, like oxygen and carbon dioxide, to pass directly through the lipid bilayer.
- Facilitated Diffusion: Involves the use of transport proteins to help substances that cannot freely diffuse across the membrane.
- Osmosis: The diffusion of water through a selectively permeable membrane.
Here is a summary of passive transport processes:
Process | Description |
Simple Diffusion | Directly through the lipid bilayer |
Facilitated Diffusion | Uses transport proteins |
Osmosis | Water movement through membrane |
A classic example of passive transport is the movement of oxygen from the lungs to the blood. Oxygen levels are higher in the alveoli (lung sacs) compared to the blood, thus oxygen naturally diffuses across the cell membranes of the lung capillaries into the bloodstream.
Temperature can affect diffusion rates; higher temperatures generally increase the rate of diffusion by energizing the molecules involved.
Which Membrane Transport Process Consumes ATP and Uses a Carrier?
Active transport differs from passive transport as it requires energy in the form of ATP to move molecules against their concentration gradient. This process ensures that essential molecules and ions are available within the cell regardless of their external concentrations.
There are two main types of active transport:
- Primary Active Transport: Directly relies on ATP to move molecules, such as ions, across a membrane. An example is the sodium-potassium pump, which maintains cellular electrochemical gradients.
- Secondary Active Transport: Also known as cotransport, it uses the energy derived from electrochemical gradients created by primary active transport. Substances move together (symport) or in opposite directions (antiport), leveraging the flow of one molecule to facilitate the movement of another.
Active transport mechanisms are fundamentally important in cellular functions such as nerve impulse transmission and nutrient absorption. For instance, the sodium-potassium pump is involved in the conduction of electrical signals along nerve cells and the regulation of cell volume, which can be critical in preventing cell lysis or apoptosis. This pump expels three sodium ions out of the cell and imports two potassium ions, vital for maintaining the resting potential essential for nerve function.
Membrane Transport Protein
Membrane transport proteins play a pivotal role in controlling what enters and exits the cell. These proteins are essential for maintaining cellular function and homeostasis.
What Are Membrane Transport Proteins?
Membrane transport proteins are integral membrane proteins facilitating the movement of ions, small molecules, or proteins across a biological membrane. These proteins are vital for maintaining the internal environment of the cell, ensuring optimal conditions for cellular processes.
- Channel Proteins: Form pores allowing specific molecules or ions to pass through by diffusion.
- Carrier Proteins: Bind to molecules and undergo a conformational change to transport them across membranes.
- Pumps: Use energy (usually ATP) to transport substances against their concentration gradient.
Below is a table summarizing types of membrane transport proteins:
Type | Function |
Channel Protein | Allows passage of ions/molecules through a pore |
Carrier Protein | Changes shape to move molecules across the membrane |
Pump | Uses energy to transport substances against a gradient |
Integral Membrane Proteins: Proteins that are permanently attached to the biological membrane and often necessary for cell communication and transport.
Functions of Membrane Transport Proteins
Membrane transport proteins have various functions that are essential for cellular activities and overall organismal health. These functions include:
- Enabling nutrient uptake and waste removal.
- Maintaining ion balance and cell volume.
- Facilitating communication between cells through signaling molecules.
- Regulating electrical activity in neurons and muscle cells.
A well-known example of a membrane transport protein is the sodium-potassium pump. This pump maintains the electrochemical gradient across the cell membrane, essential for nerve impulse transmission and muscle contraction.
Some transport proteins are highly specific, allowing only certain ions or molecules to pass through, which helps maintain selective permeability of the cell membrane.
Transport proteins can be affected by mutations, leading to various diseases. For instance, malfunction of the cystic fibrosis transmembrane conductance regulator (CFTR) protein results in cystic fibrosis, a condition that affects the respiratory and digestive systems. Understanding the structure-function relationship of membrane transport proteins is therefore critical in developing targeted treatments and therapies for such genetic disorders.
Transport Across Cell Membrane
The movement of various substances across cell membranes is a fundamental aspect of cellular function. Understanding this process is crucial for grasping how cells interact with their environment, absorb nutrients, and expel waste.
Cell Membrane Structure and Transport
The cell membrane, a bilayer of lipids with embedded proteins, controls the passage of substances in and out of the cell. This selectively permeable membrane ensures that essential molecules are transported efficiently while keeping harmful substances out.
Substances move across the cell membrane through:
- Lipid Bilayer Transport: Allows hydrophobic or small polar molecules to diffuse across.
- Transporter Proteins: Provide a passageway for ions and larger polar molecules.
Type of Transport | Characteristics |
Passive Transport | Occurs down a concentration gradient, no energy required. |
Active Transport | Occurs against a concentration gradient, requires energy. |
Selectively Permeable Membrane: A membrane that allows certain molecules to pass through while blocking others.
Mathematical Representation of Membrane Transport
Mathematical models can help describe and predict how substances move across cell membranes. These models often involve equations describing diffusion and active transport mechanisms.
An example of a diffusion process can be described using Fick's law:
Fick's Law: \[ J = -D \frac{dc}{dx} \] where J is the diffusion flux, D is the diffusion coefficient, and \(\frac{dc}{dx}\) is the concentration gradient.
In terms of active transport, the rate of transport can be modeled in relation to energy consumption:
Basic Energy Equation: \[ \text{Energy} = \text{Force} \times \text{Displacement} \]
An application of these equations can be seen in glucose transport into cells. When glucose is transported into a cell by facilitated diffusion, it follows a concentration gradient. However, in conditions where it moves against the concentration gradient, active transport mechanisms requiring ATP are involved.
The efficiency of substance transport can vary due to factors such as temperature and pH, which can affect the fluidity of the membrane and the activity of transport proteins.
Understanding membrane transport mechanisms provides insights into various physiological processes and diseases. Malfunctions in these transport systems can lead to conditions such as diabetes, where glucose transport is impaired, or cystic fibrosis, which involves defective chloride channels.
Recent advancements in technology have allowed scientists to delve deeper into the protein structures involved in membrane transport, uncovering how mutations cause certain transport proteins to malfunction. This knowledge paves the way for developing targeted therapies that can correct or compensate for these defects.
membrane transport - Key takeaways
- Definition of Membrane Transport: The movement of substances across the cell membrane, crucial for cell survival and function.
- Types of Membrane Transport: Includes passive (simple diffusion, osmosis) and active transport (primary and secondary).
- Membrane Transport Proteins: Integral proteins that assist in the transport of ions and molecules across the cell membrane.
- Transport Across Cell Membrane: The process allows interaction with the environment, absorption of nutrients, and waste expulsion.
- Passive Membrane Transport Processes Include: Simple diffusion, facilitated diffusion, and osmosis, all occurring without energy expenditure.
- Which Membrane Transport Process Consumes ATP and Uses a Carrier: Active transport moves molecules against a concentration gradient using ATP and carriers, like the sodium-potassium pump.
Learn faster with the 12 flashcards about membrane transport
Sign up for free to gain access to all our flashcards.
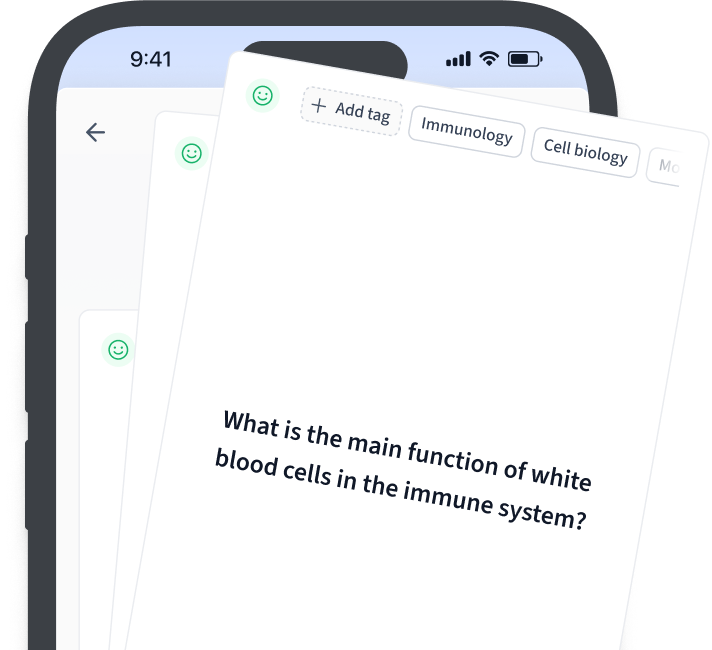
Frequently Asked Questions about membrane transport
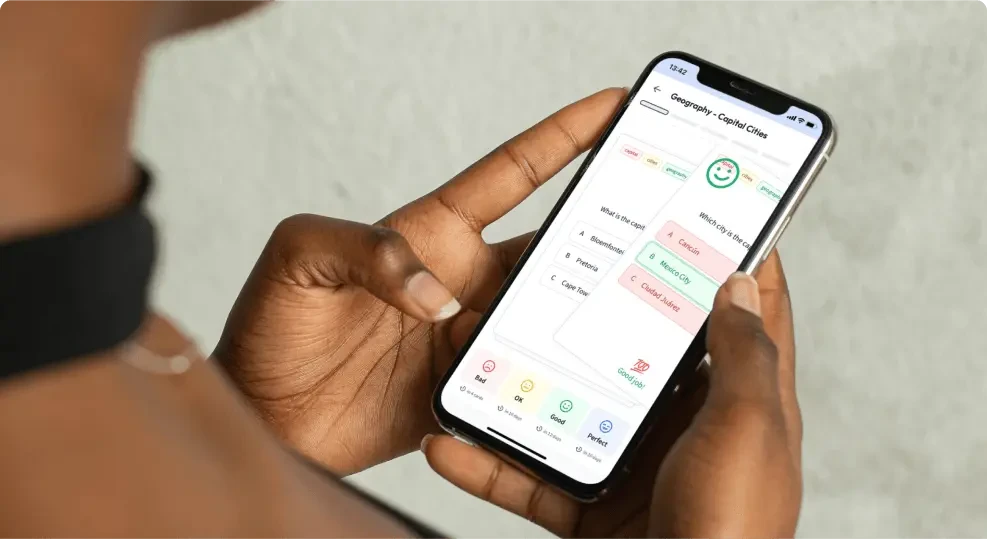
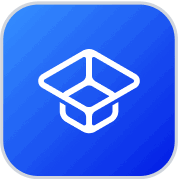
About StudySmarter
StudySmarter is a globally recognized educational technology company, offering a holistic learning platform designed for students of all ages and educational levels. Our platform provides learning support for a wide range of subjects, including STEM, Social Sciences, and Languages and also helps students to successfully master various tests and exams worldwide, such as GCSE, A Level, SAT, ACT, Abitur, and more. We offer an extensive library of learning materials, including interactive flashcards, comprehensive textbook solutions, and detailed explanations. The cutting-edge technology and tools we provide help students create their own learning materials. StudySmarter’s content is not only expert-verified but also regularly updated to ensure accuracy and relevance.
Learn more