Jump to a key chapter
Definition of Antimicrobial Nanoparticles
Antimicrobial nanoparticles are tiny particles that are synthesized to combat microorganisms like bacteria, viruses, and fungi. These particles are engineered at the nanoscale, usually ranging from 1 to 100 nanometers in diameter. Their small size and large surface area provide unique properties that make them effective in targeting and eliminating harmful microbes.
Basic Concept of Antimicrobial Nanoparticles
Antimicrobial nanoparticles work by interacting with microbial membranes, disrupting their functions, or penetrating cells to interfere with their internal processes. This unique mechanism helps in avoiding the traditional pathways that microorganisms often develop resistance to. Here are some key concepts:
- Size and shape: These factors affect the nanoparticles’ antimicrobial properties. The smaller and more uniformly shaped they are, the more effective they tend to be.
- Surface area: Greater surface area increases the contact interface with microbes, enhancing their antimicrobial effectiveness.
- Material composition: Different materials, such as silver, copper, and zinc oxide, are chosen for their specific antimicrobial properties.
Antimicrobial refers to agents that kill microorganisms or stop their growth. In the context of nanoparticles, these are tiny particles engineered with materials that exhibit these properties.
Nanoparticles exhibit different properties compared to bulk materials and their smaller counterparts, due to quantum mechanics effects at the nanoscale.
An example of antimicrobial nanoparticles in everyday use is silver nanoparticles, commonly incorporated in wound dressings to prevent infection.
The understanding of antimicrobial nanoparticles leverages advancements in nanotechnology and microbiology. Their effectiveness is developed through various methods such as chemical reduction of metals like gold and silver in controlled processes to form nanoparticles. Each type of particle, depending on its composition and functionalization, exhibits unique interactions with microbes. For example, silver nanoparticles release ions that disrupt membrane functions and generate reactive oxygen species, leading to bacterial cell death. Furthermore, within respiratory systems, certain nanoparticles can be tailored to target virus stages, potentially inhibiting viral entry or replication in the host cells.
Types of Antimicrobial Nanoparticles
There are several types of antimicrobial nanoparticles, each distinguished by their composition and method of action. Some common types include:
- Silver nanoparticles (AgNPs): Known for their broad-spectrum antimicrobial properties, silver nanoparticles are effective against both gram-positive and gram-negative bacteria.
- Copper nanoparticles (CuNPs): These exhibit excellent antiviral and antibacterial activity, often used in coatings and medical instruments.
- Gold nanoparticles (AuNPs): Used mainly for their compatibility and effectiveness in drug delivery, they can be functionalized with antimicrobial peptides.
- Zinc oxide nanoparticles (ZnO-NPs): Known for their UV protective and antibacterial properties, used in cosmetics and surface coatings.
- Chitosan nanoparticles: Derived from natural polymers, these offer biodegradable solutions with antimicrobial properties.
Silver nanoparticles are widely used in consumer products like washing machines and refrigerators, claiming to eliminate or reduce pathogens present on surfaces.
Synthesis of Antimicrobial Nanoparticles
The synthesis of antimicrobial nanoparticles involves various methods that are strategically chosen to enhance their efficiency and efficacy against pathogens. The synthesis methods determine the size, shape, and stability, which are crucial for their antimicrobial activity.
Chemical Methods for Synthesis
Chemical methods for synthesizing antimicrobial nanoparticles primarily involve reactions in controlled environments to regulate the size and distribution of the nanoparticles. These techniques often include chemical reduction, sol-gel processes, and hydrothermal methods. Here's a closer look at each technique:
- Chemical reduction: This involves reducing metal salts to their nanoparticles using reducing agents such as sodium borohydride or citric acid. For example, silver nitrate can be reduced to silver nanoparticles by sodium borohydride, expressed in equations as \[\text{AgNO}_3 + \text{NaBH}_4 \rightarrow \text{Ag} + \text{Other products}\].
- Sol-gel process: This wet-chemical technique involves the transition of a solution system from a liquid 'sol' into a solid 'gel' phase, often used to produce metal oxide nanoparticles.
- Hydrothermal synthesis: This process utilizes high pressure and temperature to enhance the crystallinity and uniformity of nanoparticles, often resulting in unique properties.
An example of chemical synthesis is the production of copper nanoparticles using hydrazine in an alkaline medium, which results in nanoparticles with a high surface area and strong antibacterial properties.
Chemical reduction methods offer high control over nanoparticle size and shape, which are critical in defining their interactions with microorganisms. The choice of reducing agent can significantly impact the nanoparticle characteristics, enhancing their specific action against bacteria or viruses. Mathematical modeling in chemical reduction can be used to predict the growth kinetics of nanoparticles and their size distribution. Commonly, the first-order kinetics equation \( N(t) = N_0 \times e^{-kt} \) is utilized for determining the concentration of metal nanoparticles formed over time, where \( N_0 \) is the initial concentration and \( k \) is the rate constant.
Biological Synthesis Techniques
Biological synthesis techniques use natural, environmentally friendly processes involving plants, algae, fungi, or bacteria to produce antimicrobial nanoparticles. This approach utilizes the biological materials' inherent reducing and stabilizing properties to create nanoparticles. Key points include:
- Microbial synthesis: Bacteria like Escherichia coli and Pseudomonas species can reduce metal ions to form nanoparticles.
- Plant-mediated synthesis: Plant extracts containing natural reducers like flavonoids and proteins facilitate the conversion of metal salts into nanoparticles.
- Algal synthesis: Algae offer a high biomass and efficient reactions to produce nanoparticles at larger scales.
For instance, tea leaf extracts can reduce silver ions to silver nanoparticles, resulting in green-synthesized nanoparticles employed in biomedical applications.
Biological synthesis methods are also known as green synthesis methods, emphasizing their eco-friendly process.
Physical Approaches in Synthesis
Physical methods employ physical forces to create antimicrobial nanoparticles and commonly involve techniques such as milling, laser ablation, and evaporation-condensation. These methods are advantageous for producing highly stable nanoparticles:
- Ball milling: A mechanical process where metal powders are ground to produce nanoparticles. It's a straightforward method but can be energy-intensive.
- Laser ablation: Involves using a laser to remove material from a bulk target to generate nanoparticles directly. It offers contamination-free production but requires precise control.
- Evaporation-condensation: A top-down method where materials are evaporated and then rapidly cooled to form nanoparticles. It's often used for high-purity nanoparticles.
Physical synthesis methods, especially laser ablation, can produce nanoparticles with unique optical properties and structures. By tuning the laser parameters, various sizes and shapes can be achieved, influencing their interaction with microbes. The dynamics of laser ablation can be modeled with evaporation rates and thermal effects, where the ablation depth, \( d \), can be expressed through the equation \( d = \frac{F}{\rho \times C_p} \), where \( F \) is the laser fluence, \( \rho \) the material density, and \( C_p \) the specific heat capacity.
Mechanism of Action of Antimicrobial Nanoparticles
Antimicrobial nanoparticles exhibit unique mechanisms to kill or inhibit the growth of microorganisms. These particles utilize their physical and chemical properties to interact with pathogens, offering an innovative approach in combating infectious diseases.
Interaction with Microbial Cells
Antimicrobial nanoparticles interact with microbial cells through direct contact with the cell membrane, penetrating their defense and interfering with crucial biological processes. - Cell membrane disruption: Many nanoparticles attach to microbial membranes, causing structural damage and leading to cell leakage and eventual death. - Penetration and internal interference: Some nanoparticles can penetrate the cell membrane, disrupting metabolic pathways and genetic material (DNA/RNA). - Production of reactive oxygen species (ROS): Certain nanoparticles induce the formation of ROS, leading to oxidative stress and cell damage.This interaction results in the effective inactivation of pathogens, proving their efficiency in treating infections.
Silver nanoparticles, when interfaced with bacteria, can cause cell wall damage leading to bacterial death by osmotic imbalance.
The effectiveness of nanoparticles on microbial cells is also influenced by environmental conditions like pH and temperature.
Unlike conventional antibiotics, nanoparticles target multiple sites in microbial cells simultaneously. For instance, in bacteria, nanoparticles might block respiratory enzymes, influence ribosome assembly, and disrupt membrane potential. These multifaceted attacks reduce the likelihood of microbe resistance development, offering a robust defense against drug-resistant strains. The research emphasizes monitoring real-time interactions through advanced microscopy techniques to map nanoscale actions better.
Role of Nanoparticle Size and Shape
Size and shape significantly influence antimicrobial properties of nanoparticles. Their unique geometric arrangements and surface areas play a critical role:
- Size: Smaller particles have a higher surface-area-to-volume ratio, leading to more surface contact with microbes and increased antimicrobial activity. This allows them to easily penetrate cell membranes.
- Shape: Nanoparticles with different shapes, such as spherical, rod-like, or triangular, exhibit varying efficacies. Certain shapes can interact more effectively with bacterial membranes.
The optimization of these parameters is key to developing highly potent nanoparticle-based antimicrobials.Factor Effect on Antimicrobial Activity Size Smaller size increases activity Shape Specific shapes enhance interactions Experiments have shown that rod-shaped silver nanoparticles tend to exhibit superior antimicrobial activity compared to spherical ones.
Release of Active Ions
The release of active ions from antimicrobial nanoparticles is a vital aspect of their mechanism. This release facilitates microbial action via several pathways:- Ion release: Nanoparticles, particularly metal-based like silver or copper, release ions that interact with microbial structures, leading to cell inactivation.- Inactivation of enzymes: Released ions can bind to enzyme active sites, preventing essential biochemical reactions within the microbes.- Membrane permeability alteration: These ions can alter microbial membrane permeability, causing imbalance and cell death.The controlled release of ions over time provides a sustained antimicrobial effect, making these nanoparticles effective in long-term applications.
Ion release from nanoparticles can be meticulously controlled through surface modifications. Functionalizing nanoparticles with polymers, for instance, can modulate the ion release rate based on environmental triggers such as pH levels or temperature changes.This tunability allows for applications in targeted therapies where precise ion dosage is crucial for maximum efficacy without causing harm to human cells. The smart design of such nanoparticles is at the frontier of personalized medicine.
Applications of Antimicrobial Nanoparticles in Medicine
Antimicrobial nanoparticles offer promising applications in the medical field, serving as effective agents against infections. Their unique properties make them suitable for various uses, from drug delivery systems to innovative coatings and wound healing solutions.
Use in Drug Delivery Systems
In drug delivery systems, antimicrobial nanoparticles play a crucial role in enhancing therapeutic outcomes. Their ability to target specific sites and control drug release offers significant advantages:
- Nanoparticles can penetrate biological barriers, facilitating drug delivery to hard-to-reach areas.
- Functionalized nanoparticles provide targeted delivery, reducing side effects and increasing drug efficacy.
- Controlled release mechanisms ensure sustained therapeutic levels over time.
An example involves encapsulating antibiotics within liposomal nanoparticles, which allows for direct delivery to infected tissues, minimizing systemic exposure.
Nanoparticles are often coated with polyethylene glycol (PEG) to improve their stability and circulation time in the body.
Advanced research in nanoparticle-enabled drug delivery systems is focusing on incorporating stimuli-responsive mechanisms such as pH-sensitive or temperature-sensitive materials. This allows nanoparticles to release their payload only under certain physiological conditions, optimizing drug effectiveness. For cancers, this means particles can remain inactive until reaching the acidic tumor environment, ensuring drugs concentrate where needed most and improving patient outcomes.
Antimicrobial Coatings and Surfaces
The integration of antimicrobial nanoparticles into coatings and surfaces presents a revolutionary approach to combating microbial contamination, particularly in healthcare settings:
- Surface coatings: Nanoparticle-infused surfaces prevent biofilm formation, a common source of hospital-acquired infections.
- Self-sanitizing materials: Tables, door handles, and other frequently touched surfaces can remain infection-free.
- Medical equipment: Instruments coated with nanoparticles help ensure sterility and reduce post-operative infections.
Titanium implants, when coated with silver nanoparticles, have shown a decreased risk of post-surgical infections, highlighting their efficacy as antimicrobial surfaces.
Antimicrobial coatings are especially useful in ventilators and other reusable medical equipment.
The effectiveness of antimicrobial coatings is amplified through the use of multifunctional nanoparticles. These include incorporating other elements, such as zinc, to provide additional benefits like UV protection and abrasion resistance, further extending the utility and lifespan of the coating. Such composite materials are under study for broader use in public transport and food processing industries, promising safer environments across various sectors.
Role in Wound Healing
Antimicrobial nanoparticles offer significant benefits in wound healing by enhancing healing processes while preventing infection:
- Infection prevention: Nanoparticles prevent colonization by pathogens, promoting aseptic conditions in the wound area.
- Enhanced tissue regeneration: They support cell proliferation and migration, essential for effective wound healing.
- Anti-inflammatory properties: Reduction in inflammation speeds up recovery and minimizes scarring.
Nano-silver dressings are frequently used in burns treatment, offering both antibacterial functions and accelerating the healing process.
Nanoparticles in wound healing reduce the need for frequent dressing changes, minimizing discomfort and potential for further injury.
Recent advances focus on combining nanoparticles with hydrogels for improved wound care. Hydrogels provide a moist environment conducive to skin regeneration, and when infused with nanoparticles, they further this by providing continuous microbial protection. The hydrogel-nanoparticle hybrid not only transports growth factors or other bioactive compounds but also offers a dynamic response to environmental changes, adjusting its properties to better support the wound healing timeline.
Antimicrobial Activity of Silver Nanoparticles
Silver nanoparticles are highly regarded for their strong antimicrobial properties, making them effective agents against a wide range of pathogens including bacteria and fungi. Their application in various fields, especially medicine, highlights their significant role in infection control.
Mechanism of Silver Nanoparticles
The antimicrobial mechanisms of silver nanoparticles involve multiple pathways, making them highly effective. These mechanisms include:
- Interaction with microbial membranes: Silver nanoparticles attach to the cell membranes of microorganisms, causing structural damage that leads to increased permeability and eventual cell death.
- Silver ion release: The nanoparticles release silver ions that penetrate cells and disrupt key cellular processes, such as respiratory enzyme functions.
- Reactive oxygen species (ROS) production: Inside microbial cells, silver nanoparticles stimulate the production of ROS, which cause oxidative stress and damage crucial biomolecules like DNA and proteins.
Studies have demonstrated that silver nanoparticles can be effective against Staphylococcus aureus, a common cause of skin infections, by altering the bacteria's membrane integrity.
The unique properties of silver nanoparticles, such as their ability to generate ROS and release ions, are often referred to as a 'nano-sword' type action. This multi-faceted attack ensures that microbes cannot easily develop resistance, unlike traditional antibiotics that typically have a single target. Research into combining silver nanoparticles with conventional antibiotics has shown enhanced synergistic effects, potentially reducing the doses needed for effective treatment and lowering the chances of side effects.
Effectiveness Against Bacteria and Fungi
Silver nanoparticles exhibit excellent effectiveness against a broad spectrum of bacteria and fungi. This includes
Nanoparticles are particularly effective against multi-drug resistant strains, providing a viable solution to combat antibiotic resistance challenges. Their ability to disrupt cell functions and induce oxidative stress is a significant factor in their broad-spectrum activity.Type Examples Gram-positive bacteria Staphylococcus aureus, Streptococcus pneumoniae Gram-negative bacteria Escherichia coli, Pseudomonas aeruginosa Fungi Candida albicans, Aspergillus niger Silver nanoparticles have been used in topical creams to treat fungal infections like athlete's foot, demonstrating their effectiveness in real-world applications.
Silver nanoparticles are also being incorporated into textiles and medical devices to provide antimicrobial properties, enhancing their application scope.
The broad-spectrum activity of silver nanoparticles is greatly influenced by their interaction with the genetic material of microbes. Studies suggest that silver ions can bind to bacterial DNA, preventing replication and transcription processes critical for bacterial growth and survival. This action, combined with the physical damage to the cell, ensures a high degree of microbe eradication. Efforts are underway to harness these properties to develop coatings and solutions for environments prone to microbial contamination, such as hospitals and food processing industries.
Challenges and Safety Considerations
Despite their benefits, the use of silver nanoparticles poses several challenges and safety considerations.
- Toxicity to human cells: While effective against pathogens, high concentrations of silver nanoparticles may pose risks to human cells, leading to potential cytotoxicity and genotoxicity.
- Environmental impact: The environmental release of silver nanoparticles raises concerns about their impact on ecosystems, particularly on aquatic life.
- Resistance development: Although less likely, prolonged exposure to silver nanoparticles might contribute to the development of resistant microbial strains.
Research is being conducted to improve the biocompatibility of silver nanoparticles by adjusting their size and coating them with stabilizing agents to reduce toxicity.
Biodegradable polymer coatings are being developed to encapsulate silver nanoparticles, minimizing their release into the environment while maintaining antimicrobial efficacy.
Emerging strategies to address safety concerns regarding silver nanoparticles include using protein or peptide coatings to enhance biocompatibility without compromising antimicrobial effectiveness. These coatings can serve as 'nano-glues' that facilitate safe adherence to desired surfaces while controlling ion release rates to manageable levels. Such innovations in surface chemistry aim to balance the antibacterial benefits with minimal adverse effects on human health and the environment, ensuring sustainable antimicrobial solutions.
antimicrobial nanoparticles - Key takeaways
- Definition of Antimicrobial Nanoparticles: Tiny particles engineered at the nanoscale to combat microorganisms such as bacteria, viruses, and fungi, exhibiting unique properties due to their small size and large surface area.
- Mechanism of Action: Antimicrobial nanoparticles disrupt microbial membranes or penetrate cells, often avoiding traditional pathways, thus reducing resistance development. They release ions that disrupt microbial functions or produce reactive oxygen species (ROS).
- Synthesis Methods: Chemical reduction, sol-gel processes, hydrothermal methods, and biological synthesis techniques involving plants or microorganisms to form antimicrobial nanoparticles.
- Applications in Medicine: Used in drug delivery systems, antimicrobial coatings for surfaces, and wound healing solutions by preventing infection, promoting tissue regeneration, and controlling release mechanisms.
- Antimicrobial Activity of Silver Nanoparticles: Silver nanoparticles are known for broad-spectrum antimicrobial effectiveness against bacteria and fungi, working through membrane disruption, ion release, and ROS production.
- Challenges and Safety Considerations: Risks include potential toxicity to human cells, environmental impact, and possible resistance development, highlighting the need for careful design and encapsulation strategies.
Learn faster with the 10 flashcards about antimicrobial nanoparticles
Sign up for free to gain access to all our flashcards.
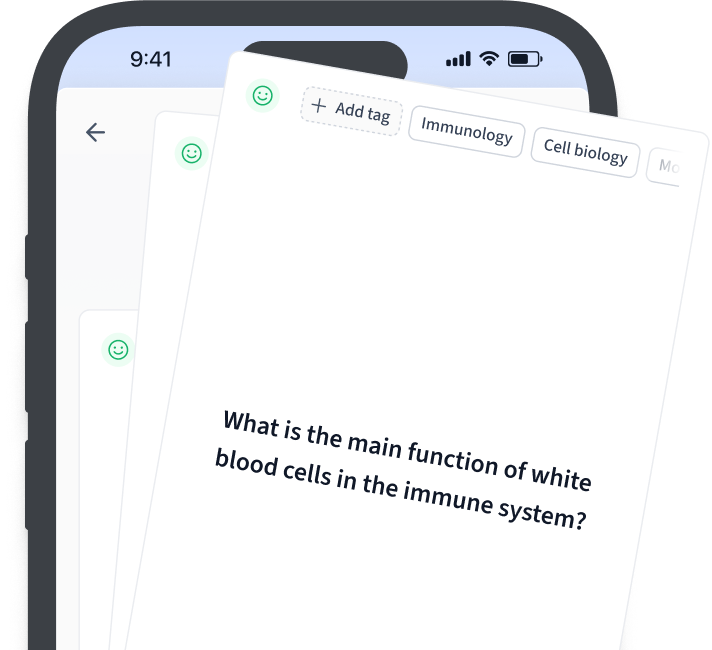
Frequently Asked Questions about antimicrobial nanoparticles
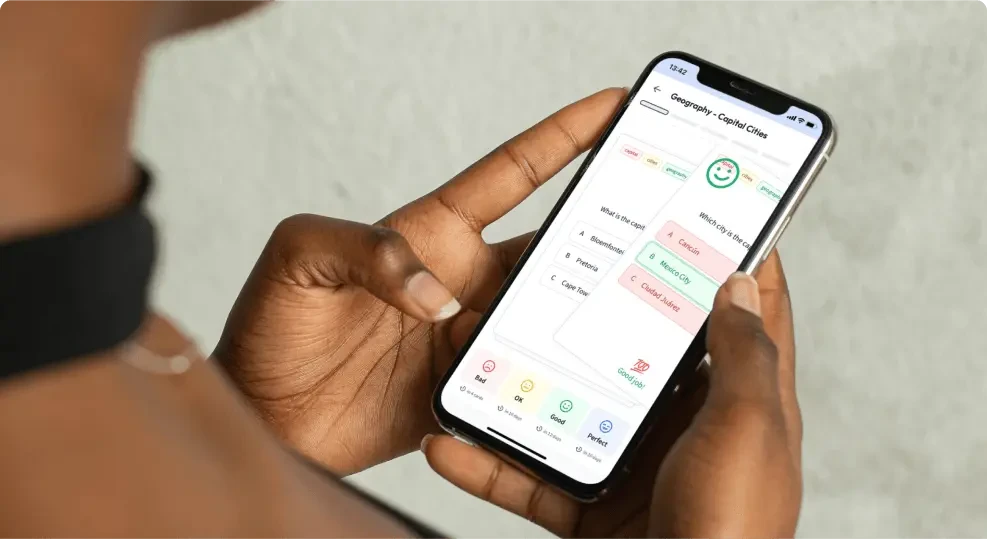
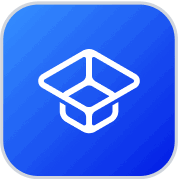
About StudySmarter
StudySmarter is a globally recognized educational technology company, offering a holistic learning platform designed for students of all ages and educational levels. Our platform provides learning support for a wide range of subjects, including STEM, Social Sciences, and Languages and also helps students to successfully master various tests and exams worldwide, such as GCSE, A Level, SAT, ACT, Abitur, and more. We offer an extensive library of learning materials, including interactive flashcards, comprehensive textbook solutions, and detailed explanations. The cutting-edge technology and tools we provide help students create their own learning materials. StudySmarter’s content is not only expert-verified but also regularly updated to ensure accuracy and relevance.
Learn more