Jump to a key chapter
Introduction to Biophysical Simulations in Medicine
Biophysical simulations are revolutionizing medicine by providing detailed models of biological processes. These simulate the behaviors of complex biological systems, offering insights into their functions and interactions. By utilizing these simulations, you can predict outcomes, understand disease mechanisms, and develop treatments with greater precision.
How Biophysical Simulations Work
Biophysical simulations involve mathematical and computational modeling to mimic biological processes. These models often include differential equations, which describe changing systems. For example, the equation for chemical reaction dynamics can be represented as: \[\frac{dC}{dt} = kC\]where \( C \) is the concentration of the reactant and \( k \) is the reaction rate constant.These simulations are constructed by inputting biological data into computational algorithms. Some key elements include:
- Parameters: Inputs like reaction rates, diffusion constants, and environmental variables.
- Equations: Mathematical formulae describing interactions.
Consider simulating the interaction between a drug and a receptor. The model might use a differential equation to describe the binding:\[D + R \underset{k_{-1}}{\overset{k_1}{\rightleftharpoons}} DR\]where \( D \) is the drug, \( R \) is the receptor, and \( k_1 \) and \( k_{-1} \) are the forward and reverse reaction rates, respectively.
Applications in Medicine
Biophysical simulations have numerous applications in the medical field. They allow for:
- Drug development: Predicting drug interactions and optimizing compounds.
- Understanding disease progression: Modeling how diseases spread at the cellular level.
- Personalized medicine: Simulating how a specific patient's body will react to a course of treatment.
In drug development, simulations can be used to perform virtual screenings of thousands of compounds before laboratory testing. This approach, called in silico experimentation, can significantly reduce the time and cost of finding effective drugs. Additionally, modeling the kinetics of drug-receptor interactions allows researchers to predict the efficacy of potential pharmaceuticals. Using the Michaelis-Menten equation:\[v = \frac{{V_{max}[S]}}{{K_m + [S]}}\]where \( v \) is the reaction velocity, \( [S] \) is the substrate concentration, \( V_{max} \) is the maximum reaction rate, and \( K_m \) is the Michaelis constant, helps understand enzyme kinetics in a realistic setting.
Biophysical simulations are not only used for research but also for educational purposes. They provide a visual and interactive way to understand complex biological processes.
Biophysical Simulations Definition and Meaning in Medicine
Biophysical simulations encompass the mathematical and computational modeling of biological processes to gain insights into their functions and dynamics. These models play a crucial role in advancing medical research and treatment.
Biophysical Simulations are computational techniques used to model biological systems and processes to understand their behaviors and predict outcomes.
Simulations can range from modeling the folding of a protein to simulating entire biological pathways. By inputting experimental data into computational models, you can understand complex interactions in biological systems.
Molecular Modeling and Simulation in Computational Biochemistry and Biophysics
Molecular modeling is a key aspect of computational biochemistry, involving the use of computer algorithms to model molecular structures and dynamics. The accuracy of these models is crucial for understanding biochemical processes and developing new therapeutic agents.
When simulating a protein-ligand interaction, you can apply molecular dynamics simulations, which calculate the forces between particles to predict movement. An equation often used in these simulations is Newton's second law: \[F = ma\]where \( F \) is the force applied, \( m \) is the mass, and \( a \) is the acceleration.
These simulations are powerful tools in:
- Predicting molecular interactions
- Understanding enzyme mechanisms
- Drug design
- Exploring protein folding
In biophysics, complex simulation methods such as quantum mechanics/molecular mechanics (QM/MM) are used to study chemical reactions within biological systems. This hybrid approach combines the precision of quantum mechanics for the reactive part of a system with the efficiency of classical mechanics for the rest. It allows for detailed simulations of interactions at the cellular level, providing valuable insights into biological processes.
High-performance computing resources are often required for advanced biophysical simulations due to the complex nature and size of the biological systems being studied.
Techniques in Biophysical Simulations
In the realm of biophysical simulations, various techniques are employed to model and analyze biological systems. These methods allow you to delve into the intricate details of biological processes, opening up pathways for innovations in medical research and treatment.
Biophysics Membranes Simulation
Membrane simulations in biophysics are essential for understanding cellular processes such as nutrient transport, signal transduction, and energy conversion. These simulations use different techniques to represent and analyze the dynamics of biological membranes.
Biophysics Membrane Simulation involves computational modeling of cellular membrane structures to study their physical properties and interactions.
These simulations typically involve:
- Lipid Bilayers: Simulating the lipid bilayers to understand their fluidity and permeability.
- Protein Interaction: Examining how proteins interact with membrane lipids.
For example, simulating the interaction of a transmembrane protein with the lipid bilayer can reveal how the protein stabilizes and changes in response to different lipid compositions. This can be examined by combining the equations of motion for all particles in the system.
In-depth studies often utilize coarse-grained models that simplify the membrane structure to enhance simulation efficiency. Whereas atomistic models prioritize detailed interactions, coarse-grained models speed up simulations by reducing the system's complexity but maintaining essential properties. Advanced computational resources are often required to run these detailed and resource-intensive models, justifying the need for high-performance computing in this field.
Modern simulations can model complex behaviors such as membrane fusion and fission, providing insights into processes like viral entry into cells.
Applications of Biophysical Simulations in Medical Research
Biophysical simulations have opened up revolutionary pathways in medical research. These computational models allow a deeper understanding of complex biological systems, facilitating advancements in diagnosing, preventing, and treating diseases. You will find them indispensable for simulating scenarios that would be infeasible or too costly to explore physically.
Drug Discovery and Development
In drug discovery, biophysical simulations play a crucial role in identifying promising drug candidates and optimizing their efficacy. By modeling the interaction between drugs and biological targets at a molecular level, these simulations help you predict pharmacodynamics and pharmacokinetics within the body.
For example, consider a simulation of a drug binding to an enzyme target. The Michaelis-Menten equation, \[v = \frac{{V_{max}[S]}}{{K_m + [S]}}\], can be used to describe the reaction rate \( v \) concerning substrate concentration \([S]\). Here, \(V_{max}\) is the maximum rate, and \(K_m\) is the Michaelis constant. These simulations provide insights into how strongly the drug binds and its potential efficacy.
Personalized Medicine
Biophysical simulations facilitate personalized medicine by allowing the prediction of how individual patients will respond to specific treatments. These models consider unique genetic, environmental, and lifestyle factors for each patient to tailor medical treatment precisely.
Personalized Medicine is an approach where medical treatment is customized to the individual characteristics of each patient.
Personalized simulations can help optimize drug dosage and reduce adverse effects, improving overall treatment outcomes.
Understanding Disease Mechanisms
Simulations are crucial in unraveling the complexities of disease mechanisms. They allow you to study the progression and effects of diseases at a cellular and molecular level, providing critical insights that inform therapeutic strategies. For instance, simulating the spread of cancer cells within a tissue matrix can help develop measures to inhibit metastasis.
A deeper exploration of disease modeling involves multi-scale simulations that integrate data across various biological levels, from molecules to entire tissues. This approach helps in understanding the systemic effects of diseases. Simulations often use models like the Lotka-Volterra equations to study interactions between species, applicable to cancer cells and normal cells. These equations are: \[\frac{{dx}}{{dt}} = x(\alpha - \beta y)\], \[\frac{{dy}}{{dt}} = -y(\gamma - \delta x)\], where \(x\) and \(y\) represent the populations, and \( \alpha, \beta, \gamma, \delta \) are coefficients representing interaction rates.
biophysical simulations - Key takeaways
- Biophysical simulations definition and meaning in medicine: Mathematical and computational modeling of biological processes to gain insights into their functions and dynamics.
- Molecular modeling and simulation computational biochemistry and biophysics: Use of computer algorithms to model molecular structures and dynamics aiding in drug design and understanding biochemical processes.
- Techniques in biophysical simulations: Differential equations, Monte Carlo algorithms (e.g., MCell), molecular dynamics simulations, and coarse-grained models.
- Biophysics membranes simulation: Computational modeling of cellular membrane structures to study their physical properties and interactions.
- Applications of biophysical simulations in medical research: Help in drug development, personalized medicine, understanding disease mechanisms, and predicting molecular interactions.
- Introduction to biophysical simulations in medicine: Simulations provide detailed models of biological processes, enhancing prediction of outcomes, disease mechanisms, and treatment development.
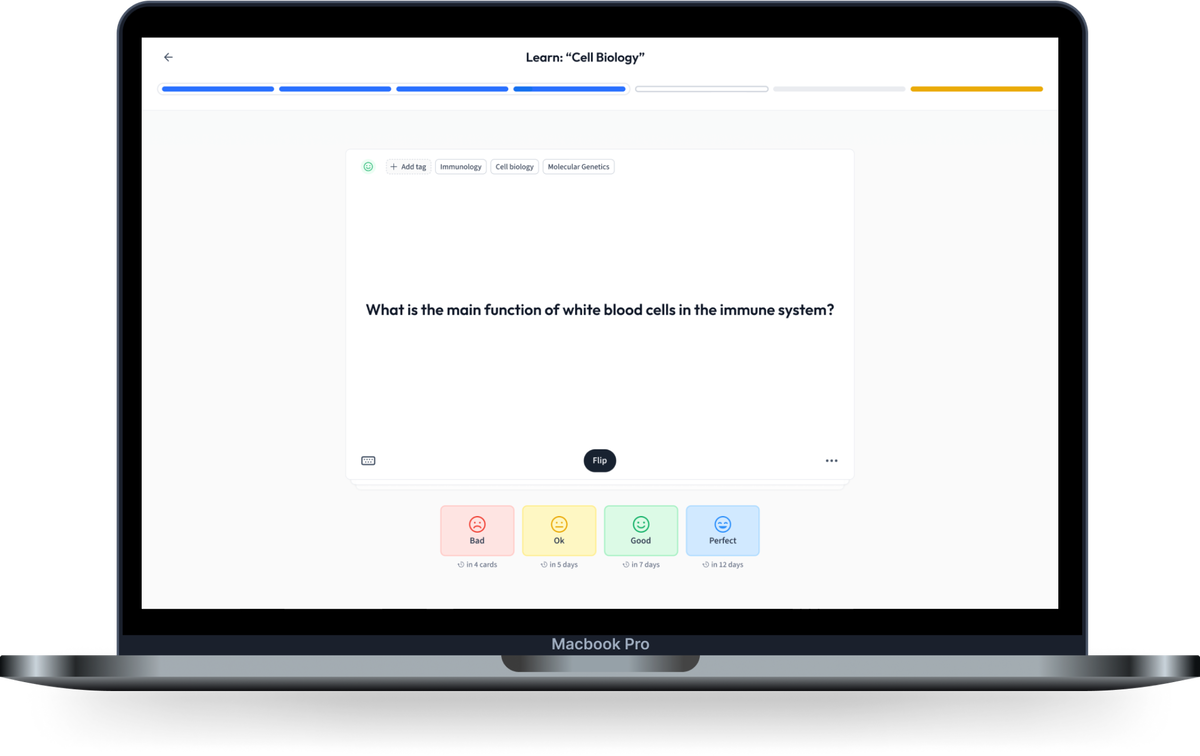
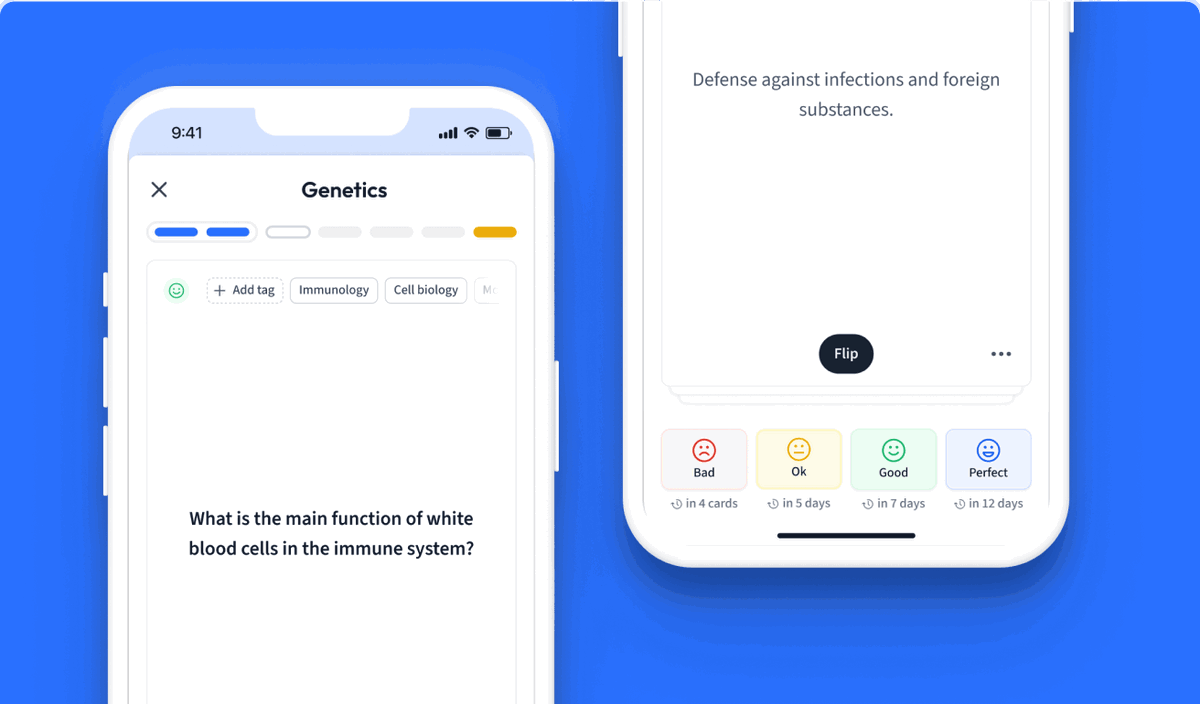
Learn with 12 biophysical simulations flashcards in the free StudySmarter app
Already have an account? Log in
Frequently Asked Questions about biophysical simulations
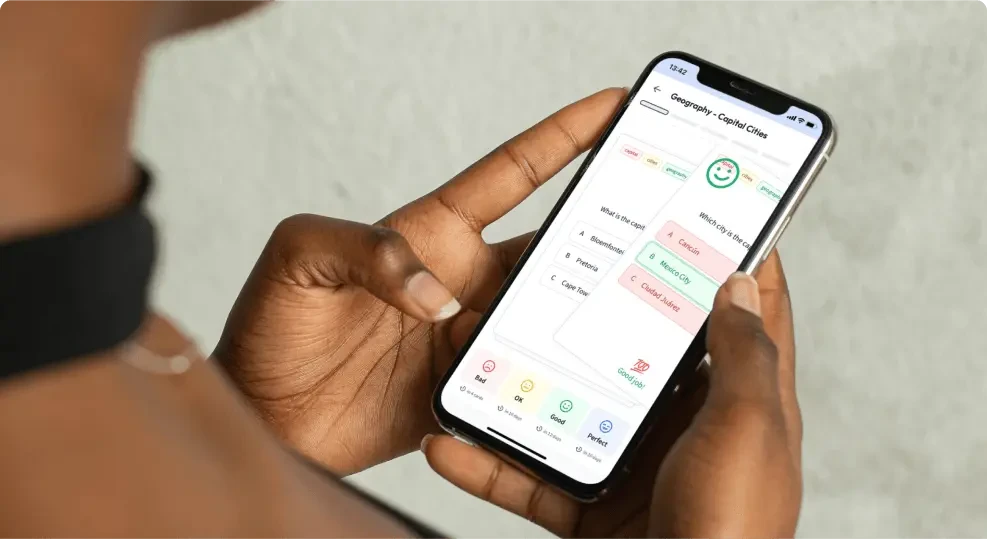
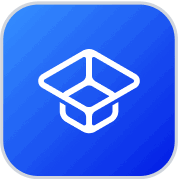
About StudySmarter
StudySmarter is a globally recognized educational technology company, offering a holistic learning platform designed for students of all ages and educational levels. Our platform provides learning support for a wide range of subjects, including STEM, Social Sciences, and Languages and also helps students to successfully master various tests and exams worldwide, such as GCSE, A Level, SAT, ACT, Abitur, and more. We offer an extensive library of learning materials, including interactive flashcards, comprehensive textbook solutions, and detailed explanations. The cutting-edge technology and tools we provide help students create their own learning materials. StudySmarter’s content is not only expert-verified but also regularly updated to ensure accuracy and relevance.
Learn more