Jump to a key chapter
Cellular Enzymology Definition
Cellular Enzymology refers to the study of enzymes within living cells. Enzymes are biological catalysts that speed up chemical reactions in the body without being consumed in the process. Understanding their function and regulation is crucial to comprehending cellular processes.
Key Concepts in Cellular Enzymology
In the study of cellular enzymology, several key concepts need to be understood. These include the structure of enzymes, the mechanisms by which they catalyze reactions, and their role in cellular metabolism. Enzymes are specific in their action, meaning each enzyme acts on specific substrates. This specificity is due to the unique structure of the enzyme's active site.
An active site is the region of an enzyme where substrate molecules bind and undergo a chemical reaction. The specific shape and chemical environment of the active site allow it to bind to the substrate with high specificity.
Enzyme activity can be influenced by several factors, including temperature, pH, and the presence of inhibitors or activators.
- Temperature: Enzymes have an optimal temperature range in which they function most effectively. Too high or too low a temperature can denature the enzyme, causing it to lose its activity.
- pH: Each enzyme has an optimal pH at which it is most active. Deviations from this pH can lead to reduced activity or denaturation.
- Inhibitors: These are molecules that decrease enzyme activity. They can bind to the enzyme's active site or to another site on the enzyme, affecting its function.
- Activators: These are molecules that increase enzyme activity, often by changing the enzyme's shape to enhance substrate binding.
For instance, the enzyme catalase catalyzes the decomposition of hydrogen peroxide into water and oxygen. The reaction can be represented as: \[ 2H_2O_2 \rightarrow 2H_2O + O_2 \] Catalase is crucial for protecting cells from oxidative damage by hydrogen peroxide.
Enzymes lower the activation energy of a reaction, making it easier for the reaction to proceed.
Enzymes often work in complexes or pathways known as metabolic pathways. These pathways are a series of enzyme-driven reactions where the product of one reaction becomes the substrate for the next. An example is the glycolysis pathway, where glucose is broken down into pyruvate, yielding energy in the form of ATP. This pathway involves several enzymes, including hexokinase, phosphofructokinase, and pyruvate kinase. The regulation of these enzymes is critical for controlling metabolic flow. Enzymes can also be regulated through allosteric regulation, where a molecule binds to a site other than the active site, causing a change in enzyme activity. This type of regulation allows cells to respond rapidly to changes in their environment and is a key aspect of cellular enzymology. A deeper understanding of enzyme kinetics can be gained through the Michaelis-Menten equation, which describes the rate of enzymatic reactions by relating reaction rate to substrate concentration. The equation is given by: \( v = \frac{V_{max}[S]}{K_m + [S]} \) where \( v \) is the rate of reaction, \( V_{max} \) is the maximum rate, \( [S] \) is the substrate concentration, and \( K_m \) is the Michaelis constant, representing the substrate concentration at half-maximal velocity. Understanding these concepts is fundamental to the field and provides insight into how life is maintained at the cellular level.
Application of Cellular Enzymology in Medicine
Cellular enzymology has a profound impact on the field of medicine by offering insights into biological processes and serving as a foundation for developing innovative solutions. It plays a vital role in both drug development and diagnostic innovations, leading to advancements that improve healthcare outcomes.
Drug Development Applications
In the realm of drug development, understanding enzymes is crucial for designing effective treatments. Enzymes are often targeted by drugs to either inhibit or enhance their activity. Drug developers take advantage of the specificity of enzymes to create medications with minimal side effects. Here are some applications:
- Many current therapies, especially for diseases like cancer, actively target enzymes involved in cell proliferation.
- Enzyme inhibitors are widely used as drugs in treating various conditions. For example, protease inhibitors are used in managing HIV/AIDS.
A prime example of enzyme-targeted drug development is the use of ACE (Angiotensin-Converting Enzyme) inhibitors. These drugs are used to treat high blood pressure and heart failure by inhibiting the action of ACE, which reduces blood vessel constriction.
The development of enzyme replacement therapies has been revolutionary for genetic disorders where specific enzymes are deficient.
Enzyme engineering is a fascinating field within drug development that involves modifying enzyme structures to improve drug efficacy. This may involve altering the enzyme’s stability or changing its substrate specificity to better suit medical needs. Another intriguing area is the use of allosteric modulators which bind to an enzyme at a site other than the active site, inducing a conformational change that affects enzyme activity. Allosteric modulation can offer more finely-tuned control over enzyme activity compared to traditional inhibitors. For example, HDAC (Histone Deacetylase) inhibitors work as allosteric modulators, impacting gene expression patterns in cancer therapy, showcasing the potential for innovative treatments stemming from enzyme manipulation.
Diagnostic Innovations
In diagnostics, cellular enzymology has led to breakthroughs in identifying diseases more precisely and swiftly. Enzymes serve as biomarkers; their presence, absence, or altered activity can indicate particular disease states. Technological advances have allowed these enzyme-based diagnostics to become a staple in medical testing. Key innovations include:
- Enzyme-linked Immunosorbent Assays (ELISA) use antibodies and an enzyme reaction to detect the presence of proteins and hormones for disease diagnosis.
- Enzyme activity assays are employed to measure the concentration of specific enzymes in bodily fluids, helping diagnose conditions such as liver or pancreatic diseases.
An example of enzymology in diagnostics is the use of the enzyme amylase to diagnose pancreatitis. Elevated levels of amylase in the blood can indicate inflammation of the pancreas.
Advancements in enzymatic diagnostics have greatly benefited from molecular biology techniques, such as polymerase chain reaction (PCR), which amplifies DNA sequences. PCR relies on the enzyme DNA polymerase to replicate DNA, and its applications have been transformative in both medical and research settings. The development of point-of-care testing devices, which often utilize enzyme-based reactions, has brought diagnostics closer to patients, providing rapid results and aiding in immediate clinical decision-making. Furthermore, the rise of biosensors that detect enzymatic activity has opened up new avenues for non-invasive monitoring of diseases such as diabetes, where glucose levels can be tracked continuously thanks to enzymatic glucose sensors.
Cellular Enzymology Techniques
Cellular enzymology techniques are essential tools in understanding enzyme behavior and function within cells. These methods enable researchers to investigate enzyme dynamics, interaction with substrates, and their role in cellular processes. In this section, you'll explore both common laboratory techniques and advanced approaches utilized in the study of cellular enzymology.
Common Laboratory Techniques in Cellular Enzymology
Common laboratory techniques form the backbone of enzymology research. Techniques such as spectrophotometry, chromatography, and electrophoresis are frequently employed due to their efficacy in analyzing enzymatic reactions.
- Spectrophotometry is utilized to measure enzyme activity by detecting changes in absorbance. For example, the conversion of a substrate into a product might cause a measurable color change.
- Chromatography is used to separate and analyze proteins and enzymes, enabling the study of enzyme purity and activity.
- Electrophoresis allows for the separation of proteins based on their size and charge, useful in identifying enzyme isoforms.
A common example of using spectrophotometry in enzymology is the assay of lactate dehydrogenase (LDH) activity. The reaction converts lactate to pyruvate, with NAD+ turning into NADH, detectable at 340 nm: \[ \text{lactate} + \text{NAD}^+ \rightarrow \text{pyruvate} + \text{NADH} + \text{H}^+ \].
Before running any enzymatic assay, always ensure that your enzyme sample is properly purified to obtain accurate data.
While spectrophotometric assays are foundational, they can be complemented by methods such as mass spectrometry for protein identification and characterization. Mass spectrometry can provide detailed information about enzyme modifications and interactions when paired with chromatography. Additionally, techniques like enzyme-linked immunosorbent assays (ELISA) use antibodies to quantify enzyme concentration or activity, offering a sensitive alternative to spectrophotometry in certain contexts. Integration of multiple techniques often yields the most comprehensive data, enabling a thorough understanding of enzyme functionality and regulation.
Advanced Techniques and Their Uses
As technology advances, more specialized and sophisticated techniques have emerged in cellular enzymology. These methods offer enhanced precision and enable the exploration of enzyme activity in complex biological contexts. Advanced techniques include X-ray crystallography and Nuclear Magnetic Resonance (NMR) spectroscopy.
- X-ray Crystallography: This technique allows for the determination of the 3D structure of enzymes at atomic resolution, providing insights into the active site's architecture and the mechanism of catalysis.
- Nuclear Magnetic Resonance (NMR) Spectroscopy: NMR is used to study enzyme dynamics, monitoring how enzymes and substrates interact in solution over time.
Using X-ray crystallography, scientists have elucidated the structure of the HIV protease, revealing the enzyme's active site and aiding in the design of protease inhibitors critical for HIV treatment.
Combining multiple advanced techniques can provide a more comprehensive understanding of enzyme mechanics and interactions.
One notable advancement in enzymology is the use of cryo-electron microscopy (cryo-EM), which allows for the visualization of enzymes and their complexes without the need for crystallization. This technique can capture multiple states of an enzyme's catalytic cycle, offering a dynamic view of enzymatic action. Coupling cryo-EM with computational modeling further extends its capabilities, permitting the simulation of enzyme reactions at a molecular level. Additionally, kinetic isotope effects provide insights into reaction mechanisms, as isotopic labeling can reveal detailed steps in enzymatic catalysis. These advanced techniques are continually evolving, pushing the boundaries of what can be discerned about enzymes in their native environments.
Importance of Cellular Enzymology
Cellular enzymology is a cornerstone in the understanding of life at a molecular level. It focuses on the role of enzymes, which are critical in facilitating metabolic processes, diagnosing diseases, and even in the development of therapeutic strategies. The study of enzymology is essential for deciphering the complex biochemical pathways that sustain life.
Cellular Enzymology and Metabolic Processes
Enzymes serve as catalysts in various metabolic processes, which are crucial for energy production within a cell. This includes pathways such as glycolysis, the citric acid cycle, and oxidative phosphorylation, which altogether form the backbone of cellular metabolism. Without these enzymes, these pathways would proceed too slowly to sustain life.
Metabolic pathways are a series of chemical reactions occurring within a cell that lead to the conversion of substrates into desired end products, which are essential for cellular function and survival.
Enzymes provide specificity and regulation in metabolic pathways. They help in:
- Controlling the rate of metabolic reactions to meet the cell’s needs
- Ensuring that metabolic pathways proceed in the correct order due to their substrate specificity
- Allowing cells to respond rapidly to changes in energy demands
For instance, the enzyme hexokinase is involved in the early stages of glycolysis, converting glucose into glucose-6-phosphate, which then continues through the glycolytic pathway to generate ATP, a key energy currency for the cell.
Each step in a metabolic pathway is typically controlled by a distinct enzyme, which means multiple enzymes work in concert to execute complex reactions within the cell.
A deeper insight into cellular enzymology demonstrates the concept of enzyme regulation through allosteric enzymes and feedback inhibition. Allosteric enzymes can be modulated by molecules binding to sites distinct from their active sites, enabling them to act as sensors for the cell’s metabolic state. Feedback inhibition is a regulatory mechanism where the end product of a metabolic pathway inhibits an upstream process, preventing the overproduction of intermediates and conserving resources. These regulatory mechanisms are pivotal for balancing metabolic demands and ensuring the efficiency of cellular processes.
Cellular Enzymology Role in Disease
Enzymes are integral not just to normal cellular function but also in pathology. Disorders in enzyme function lead to a plethora of diseases, illustrating the vital role of enzymology in medical diagnostics and treatment. Abnormal enzyme function can contribute to disease states in the following ways:
Overactivity | Causes conditions such as hyperthyroidism, where excess enzyme action accelerates metabolism |
Underactivity | Leads to deficiencies as seen in genetic disorders, like phenylketonuria (PKU) |
Enzyme mutations | Result in dysfunctional proteins, affecting pathways like those seen in cystic fibrosis |
A classic case is the genetic condition phenylketonuria (PKU), where a deficiency in the enzyme phenylalanine hydroxylase inhibits the conversion of phenylalanine to tyrosine, causing harmful levels of phenylalanine to accumulate.
Inhibitors used in therapies often mimic natural metabolic controls, blocking enzymes that become pathogenic.
Research into enzyme deficiencies has led to the development of enzyme replacement therapies (ERT), which involve supplementing patients with the functional form of an enzyme. ERT is used in diseases such as Gaucher’s and Fabry disease, where recombinant DNA technology is utilized to produce human enzymes synthetically, providing life-changing treatments for individuals with these genetic disorders. Additionally, enzyme inhibitors have proven crucial in developing drugs for conditions such as hypertension (using ACE inhibitors) and diabetes (utilizing DPP-4 inhibitors), highlighting the translation of enzymology into clinical interventions.
cellular enzymology - Key takeaways
- Cellular Enzymology Definition: The study of enzymes within living cells, focusing on their structure, function, and role in cellular metabolism.
- Importance in Metabolic Processes: Enzymes are critical in facilitating and regulating metabolic pathways, necessary for energy production and maintaining cellular function.
- Applications in Medicine: Cellular enzymology aids in drug development, targeting enzymes for therapies, and enhancing diagnostics through enzyme biomarkers and assays like ELISA.
- Role in Disease: Abnormal enzyme activity can lead to diseases, with applications in diagnostics and enzyme replacement therapies providing treatment options.
- Common Techniques: Techniques such as spectrophotometry, chromatography, and electrophoresis are employed to study enzyme activities and reactions.
- Advanced Techniques: X-ray crystallography and NMR spectroscopy provide detailed insights into enzyme structures and dynamics, crucial for understanding catalytic mechanisms.
Learn faster with the 12 flashcards about cellular enzymology
Sign up for free to gain access to all our flashcards.
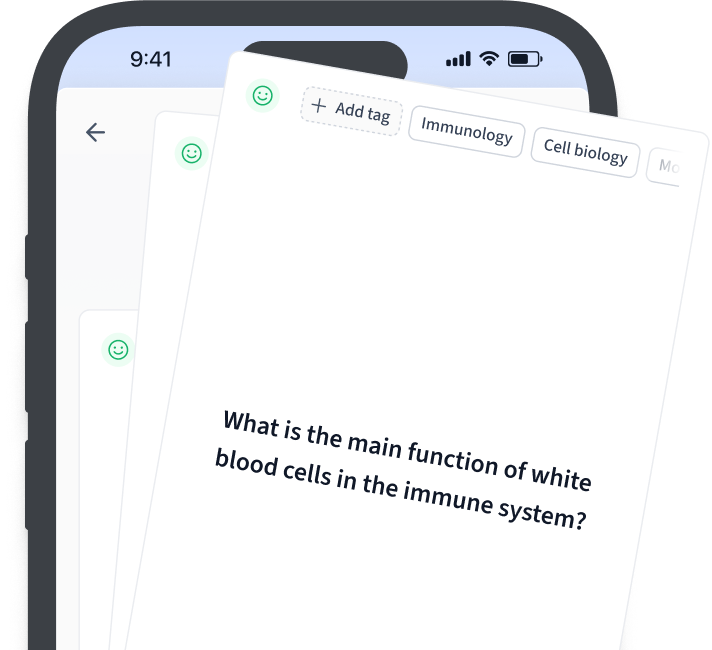
Frequently Asked Questions about cellular enzymology
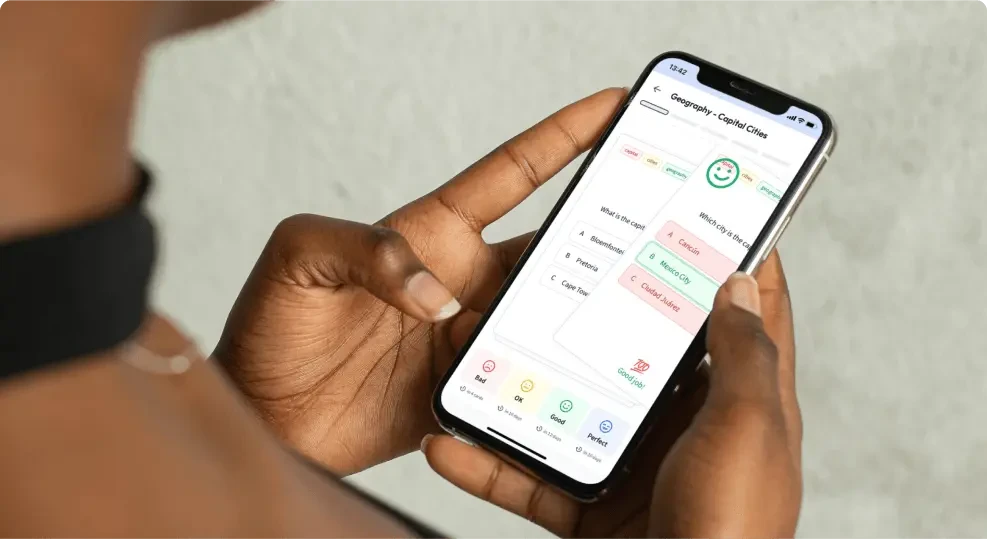
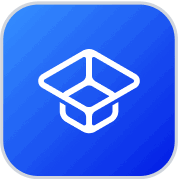
About StudySmarter
StudySmarter is a globally recognized educational technology company, offering a holistic learning platform designed for students of all ages and educational levels. Our platform provides learning support for a wide range of subjects, including STEM, Social Sciences, and Languages and also helps students to successfully master various tests and exams worldwide, such as GCSE, A Level, SAT, ACT, Abitur, and more. We offer an extensive library of learning materials, including interactive flashcards, comprehensive textbook solutions, and detailed explanations. The cutting-edge technology and tools we provide help students create their own learning materials. StudySmarter’s content is not only expert-verified but also regularly updated to ensure accuracy and relevance.
Learn more