Jump to a key chapter
Microfluidics in Nanotechnology: An Overview
Microfluidics has emerged as a vital technology in the domain of nanotechnology, offering new possibilities and applications. By manipulating fluids at the microscale, microfluidics provides precision and control that are crucial for various scientific and industrial processes. Below, you'll delve into microfluidic devices in nanotechnology, understanding their applications and fundamental concepts.
Understanding Microfluidic Devices in Nanotechnology Applications
Microfluidic devices play a significant role in nanotechnology, notably in the development of lab-on-a-chip systems. These devices provide compact, efficient solutions for chemical and biological analyses, often requiring minimal sample sizes and reagents. Here's a glimpse into the applications where these devices make a profound impact:
- Biomedical Analysis: Microfluidic technology is leveraged for point-of-care diagnostic tools, enabling quick and accurate tests for various ailments.
- Pharmaceutical Development: Accelerating drug development processes by providing high-throughput screening methods and personalized medicine diagnostics.
- Environmental Monitoring: Detecting pollutants in air and water with precision, aiding in environmental conservation efforts.
- Food Safety: Ensuring the quality and safety of food products by identifying contaminants swiftly.
Microfluidic Device: A small-scale device that controls, manipulates, and analyzes minimal amounts of fluids using channels with dimensions in the micrometer scale.
An example of a microfluidic device is a lab-on-a-chip that performs polymerase chain reactions (PCR) for DNA amplification, requiring only a few microliters of reagents and samples.
The science behind microfluidic devices involves complex principles such as laminar flow, diffusion, and capillary force. In these systems, the Reynolds number, which is calculated as \[Re = \frac{\rho vL}{\mu}\]where \(\rho\) is the fluid density, \(v\) is the velocity, \(L\) is the characteristic length, and \(\mu\) is the dynamic viscosity, is exceptionally low, often less than 1. This implies that fluid flow is laminar rather than turbulent, a condition essential for precise control and manipulation. The predictability afforded by laminar flow enables microfluidics in its diverse applications, particularly in environments where precision is paramount. Another crucial aspect is diffusion, described by Fick's Laws, which governs mass transport in microchannels, essential for processes such as mixing and reaction in microfluidic devices.
Microfluidic Devices in Nanotechnology Fundamental Concepts
A sound understanding of the fundamental concepts of microfluidics is vital for leveraging its full potential in nanotechnology. Below are some core concepts and principles behind microfluidic devices:
- Laminar Flow: Due to small dimensions, fluids often flow smoothly parallel to boundaries, which enhances control over fluid streams.
- Surface Tension: Microchannels have a high surface-to-volume ratio, so surface effects dominate over inertial forces, crucial for manipulating tiny fluid quantities.
- Capillary Action: This phenomenon allows movement of liquid through a narrow space without external forces, utilized in microfluidic pumps.
- Electroosmotic Flow (EOF): Generated by an electric field acting on charge in the diffuse layer of fluid, EOF is a key principle for controlling fluid movement in microchannels.
The science of microfluidics not only revolutionizes industries but also inspires designs in natural systems, mimicking biological microenvironments to create innovative solutions.
Microfluidics in Nanomedicine Applications
Microfluidics is transforming practices in nanomedicine by offering precise control over small volumes of fluids, which is essential in diagnostics and therapeutic solutions. Its applications in nanomedicine are particularly noteworthy as they help in enhancing treatment accuracy and reliability.
How Microfluidics Revolutionizes Nanomedicine
The influence of microfluidics in nanomedicine cannot be overstated. It plays a crucial role in various aspects of medical research and healthcare. Here's how it brings revolution to the domain:
- Enables tailored drug delivery by creating nanoparticles that can precisely target diseased cells, minimizing side effects.
- Enhances the efficiency of diagnostic devices, such as lab-on-a-chip systems, offering rapid and accurate disease detection at the point of care.
- Facilitates the development of biomimetic environments that replicate human physiological conditions, allowing for more relevant in vitro testing and cellular analysis.
A pivotal example of microfluidics in action is the use of a microfluidic chip for screening potential antiviral drugs against a novel virus rapidly. This method allows the simultaneous testing of multiple compounds, significantly accelerating the discovery of effective treatments.
Microfluidics contributes significantly to the creation of sophisticated drug delivery systems. For instance, microfluidic techniques can engineer drug-loaded nanoparticles with high specificity. The drug release can be modeled with equations accounting for nanoparticle degradation:\[M_t/M_{\text{total}}=k \times t^n\]where \(M_t\) is the drug released over time \(t\), \(M_{\text{total}}\) is the total drug, \(k\) represents the kinetic rate constant, and \(n\) is the release exponent. Such precise control ensures better treatment outcomes by aligning the drug's release profile with the therapeutic window. Furthermore, by utilizing customized polymers, microfluidic devices are tailored to adjust the degradation rate, ensuring optimal drug distribution.
Microfluidics Principles in Nano Medicine Explained
To grasp the role of microfluidics in nanomedicine fully, understanding its fundamental principles is crucial.
- Laminar Flow: In microfluidic devices, the flow of fluids is typically laminar, characterized by parallel layers with no disruption between them, which is essential for precisely targeting disease sites without diffusion-based errors.
- Fluid Dynamics: Fluid behavior in microchannels is heavily dictated by factors defined by Reynolds Number, calculated as \[Re = \frac{\rho v L}{u}\], where \(\rho\) is fluid density, \(v\) is velocity, \(L\) is characteristic length, and \(u\) is the kinematic viscosity, influencing flow regimes within devices.
- Surface Tension: Phenomena such as capillary action are crucial in transporting fluids in microchannels, pushing the boundaries of what's achievable with liquid manipulation at such small scales.
Temperature control in microfluidic devices allows manipulation of reaction rates and can simulate physiological conditions, proving valuable in drug interaction studies.
Microfluidics Technique in Medical Diagnostics
Microfluidics stands at the forefront of innovation in medical diagnostics, transforming how you approach and interpret test results. The precision it offers is vital for developing efficient diagnostic tools that are swift, reliable, and cost-effective.
Role of Microfluidic Devices in Accurate Diagnoses
Microfluidic devices are key players in enhancing the precision and efficiency of diagnostic processes. Their ability to handle small fluid volumes makes them ideal for tasks across various medical applications.
- Early Detection: These devices enable the early detection of diseases such as cancer and infectious pathogens, at stages where treatment can be more effective.
- Point-of-Care Testing: With microfluidics, you can achieve real-time testing, significantly reducing the wait time for diagnostic results.
- Cost Efficiency: By using minimal reagents and samples, microfluidic diagnostics offers a financially sustainable approach, especially for large-scale testing.
- Automation and Integration: These devices integrate multiple lab functions on a single chip, streamlining processes and reducing human error.
A typical example is microfluidic biosensors used to measure glucose levels in diabetic patients. These devices provide rapid readings by analyzing a small blood sample, allowing daily monitoring and management of blood sugar levels.
In regions with limited healthcare infrastructure, microfluidic devices serve as vital tools for carrying out essential diagnostics, improving healthcare accessibility.
Innovations in Diagnostics Through Microfluidics
Continuous advancements in microfluidics are revolutionizing diagnostic solutions, extending capabilities beyond traditional methods. Innovations are reflected in:
- Multiplexed Assays: Performing simultaneous tests increases the breadth and depth of diagnostic information obtained from a single sample.
- Integrated Systems: Combining sample processing, analysis, and data reporting into one device reduces complexity and promotes usability in various settings.
- Wearable Diagnostics: Development of microfluidic wearables aids in continuous health monitoring, offering insights on a person's health status in real-time.
- Cell Sorting and Analysis: Enhanced microfluidic methods enable precise analysis and sorting of individual cells, vital for research and personalized medicine.
Dive deeper into the realm of innovative diagnostics with the application of microfluidics in personalized medicine. The platform allows for a personalized diagnostic approach by utilizing breakthroughs like CRISPR-based detection systems housed within a microfluidic chip. The technology leverages CRISPR/Cas systems for specific nucleic acid sequence detection, adjusting on-demand to accommodate various genetic signatures. In turn, this advances the precision of diagnoses, tailoring healthcare strategies to match individual patient profiles. Microfluidic platforms facilitate this process by providing a controlled environment for enzymatic reactions, necessitating significantly less sample volume while simultaneously increasing the assay's sensitivity and specificity. This deep integration of microfluidics and advanced genetic tools signifies a leap forward in not just detecting but also understanding and treating illnesses at a molecular level.
Future of Microfluidics in Nanotechnology
Microfluidics is paving the way for exciting advances in nanotechnology, offering new dimensions for innovations and applications that extend beyond current capabilities. As you explore this field, you'll see how microfluidics is set to revolutionize various aspects of technology, enhancing precision, efficiency, and control in numerous scientific endeavors.
Emerging Trends in Microfluidics Applications
Emerging trends in microfluidics are shaping the future of this technology, providing dynamic solutions to complex challenges. Here are some of the key trends that you'll find in the landscape today:
- Integration with Artificial Intelligence (AI): Combining AI with microfluidics is set to automate and optimize processes, enabling more accurate predictive modeling and decision-making in real-time.
- Wearable Microfluidic Devices: From health monitoring to environmental sensors, wearable microfluidics offers personalized insights, adapting to the body's changing conditions or external environments.
- Environmental Sustainability: Innovations in eco-friendly materials for microfluidic devices are reducing the ecological footprint, promoting sustainable practices in manufacturing and usage.
- Point-of-Need Diagnostics: Expanding the capabilities of microfluidic devices beyond laboratories to provide diagnostic solutions at remote locations or under-resourced areas, bringing healthcare closer to the end-users.
For instance, microfluidic technology is being utilized to develop advanced blood glucose monitoring systems that are non-invasive and provide continuous tracking of glucose levels in individuals with diabetes. Such devices represent a fusion of microfluidic technology with wearable applications, enhancing user comfort and data accuracy.
Delving deeper, the integration of microfluidics with quantum dot technology opens a new frontier for sensing and imaging applications. Quantum dots, possessing unique optical properties, are coupled with microfluidic chips to create powerful sensors capable of detecting and analyzing pollutants at extremely low concentrations. The sensitivity of these devices is defined by the excitation wavelength \(u\) of the quantum dots, which can be tuned specifically for different analytes. The reliance on microfluidics provides a controlled environment for the interaction of samples with quantum dots, improving the specificity and reliability of the detection. This integration holds promise for innovative solutions in environmental monitoring, healthcare diagnostics, and material sciences.
Pioneering Research in Microfluidics and Nanomedicine
Pioneering research in the intersection of microfluidics and nanomedicine is fostering breakthroughs that promise to reshape medical diagnostics, treatment, and research. Below are some cutting-edge areas where this research is particularly impactful:
- Organ-on-Chip Models: Simulating human organ functions on microfluidic chips provides a novel platform for drug testing and disease modeling, offering more accurate predictions of how diseases progress and how drugs perform in human-like environments.
- Microfluidic Biofabrication: Advanced biofabrication techniques using microfluidics allow for the creation of complex tissue constructs with cellular precision, enabling progress in tissue engineering and regenerative medicine.
- Nanoparticle Synthesis: Controlled synthesis and functionalization of nanoparticles is possible through microfluidic reactors, leading to new possibilities in targeted drug delivery and diagnostics.
- Circulating Tumor Cell Analysis: Microfluidic devices enable the isolation and analysis of circulating tumor cells from blood samples, aiding in early cancer detection and monitoring the effectiveness of treatments.
Incorporating multi-material 3D printing in microfluidics is advancing the creation of more complex and functional devices, pushing the boundaries of what's achievable in design and functionality.
microfluidics in nanotechnology - Key takeaways
- Microfluidics in Nanotechnology: Microfluidics is crucial for precision and control in manipulating fluids at the microscale in various scientific and industrial processes.
- Microfluidic Devices: These devices are essential in creating lab-on-a-chip systems for efficient chemical and biological analyses, requiring minimal samples and reagents.
- Microfluidics Principles: Key principles include laminar flow, surface tension, capillary action, and electroosmotic flow, vital for controlling fluids in microchannels.
- Microfluidics in Nanomedicine: Offers precise fluid control, crucial for diagnostic and therapeutic solutions, enhancing treatment accuracy and reliability.
- Medical Diagnostics Applications: Microfluidic devices enable early disease detection, point-of-care testing, and cost-efficient diagnostics using minimal samples.
- Future Trends: Emerging trends include AI integration, wearable devices, eco-friendly materials, and point-of-need diagnostics, shaping the future of microfluidics.
Learn faster with the 12 flashcards about microfluidics in nanotechnology
Sign up for free to gain access to all our flashcards.
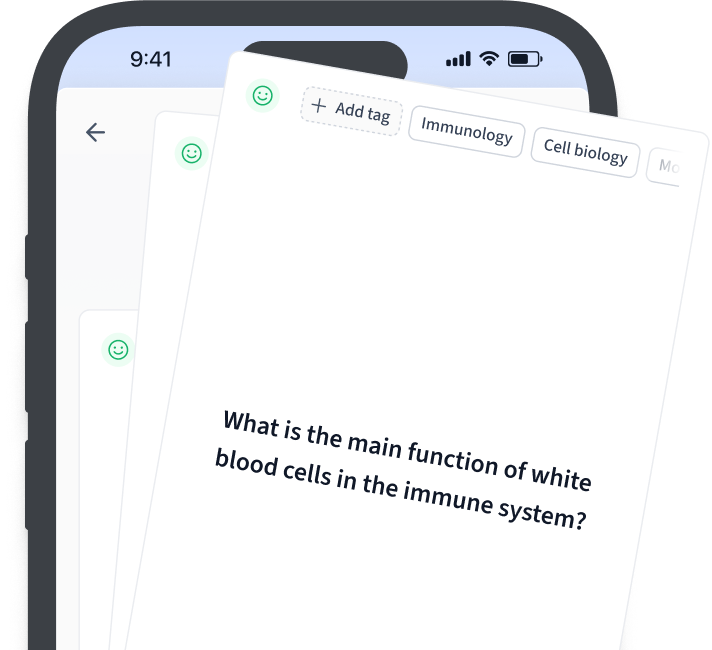
Frequently Asked Questions about microfluidics in nanotechnology
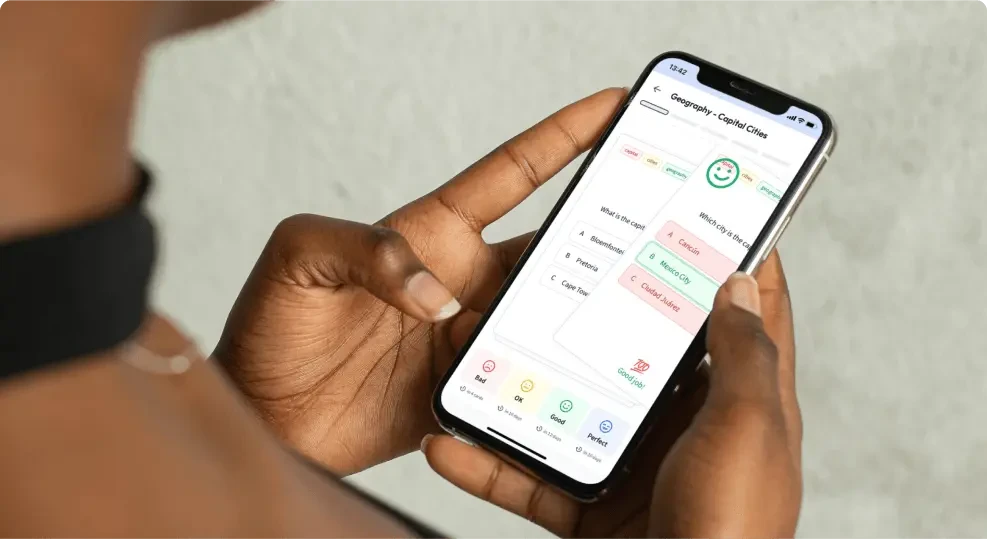
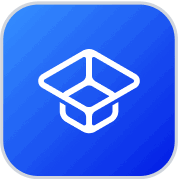
About StudySmarter
StudySmarter is a globally recognized educational technology company, offering a holistic learning platform designed for students of all ages and educational levels. Our platform provides learning support for a wide range of subjects, including STEM, Social Sciences, and Languages and also helps students to successfully master various tests and exams worldwide, such as GCSE, A Level, SAT, ACT, Abitur, and more. We offer an extensive library of learning materials, including interactive flashcards, comprehensive textbook solutions, and detailed explanations. The cutting-edge technology and tools we provide help students create their own learning materials. StudySmarter’s content is not only expert-verified but also regularly updated to ensure accuracy and relevance.
Learn more