Jump to a key chapter
Definition of Nanofabrication Methods
Nanofabrication methods encompass a range of techniques used to build devices on the nanoscale. This scale operates at dimensions around one billionth of a meter, often manipulating matter at the atomic or molecular levels. These methods are crucial for applications in electronics, medicine, and materials science, enabling the creation of structures and components that exhibit novel properties not seen in bulk materials.
Nanofabrication Methods: Techniques used to construct and manipulate materials on the nanoscale, enabling the development of complex devices and systems with unique properties.
Example of a Nanofabrication Method: One common nanofabrication method is photolithography, which is used extensively in the semiconductor industry to create microcircuits on silicon wafers. Photolithography involves:
- Coating a substrate with a photosensitive material called photoresist.
- Exposing the photoresist to light through a mask that defines the desired pattern.
- Developing the photoresist to leave behind the pattern on the substrate.
- Etching away the substrate revealed by the developed photoresist to create the nanostructure.
Tip: Think of nanofabrication like building with LEGO blocks, but on a scale almost invisible to the naked eye.
Nanofabrication integrates several scientific disciplines, including physics, chemistry, and material science. At such small scales, quantum mechanical effects become significant, influencing the properties of materials and offering enhanced functionalities. For example, the quantum confinement effect, where electronic properties of materials are altered due to their restricted size, is a crucial aspect of nanofabrication. This effect can be seen with quantum dots, semiconductor particles that have quantum mechanical properties that differ from larger materials. Mathematically, these effects can be explored with the Schrödinger equation for a particle in a potential box, expressed as: \[ E_n = \frac{{n^2 h^2}}{{8mL^2}} \]Where:
- E_n is the energy of the nth level,
- h is Planck's constant,
- m is the particle's mass,
- L is the length of the confinement box,
- n is a positive integer denoting the energy level.
Techniques in Medical Nanofabrication
Medical nanofabrication techniques are pivotal in advancing biomedical applications at the nanoscale. These methods are employed to create nanoscale structures that can interact with biological systems, offering possibilities for targeted drug delivery, tissue engineering, and diagnostic tools.
Lithography in Medical Nanofabrication
Lithography is a widely used technique in nanofabrication. In medical applications, it is utilized to fabricate microneedles and nanoparticles that can deliver drugs or serve as imaging agents.The lithography process involves several steps:
- Photoresist application: A light-sensitive material is applied to the substrate.
- Exposure: The photoresist is exposed to light through a patterned mask.
- Development: The exposed photoresist is developed, revealing the pattern.
- Etching: The exposed areas are etched away, creating nanostructures on the substrate.
Example: Microneedle ArraysMicroneedle arrays fabricated via lithography are used to deliver drugs painlessly through the skin. These tiny needles penetrate the outer layer of the skin without reaching nerve endings, minimizing pain. Additionally, microneedles can be structured to allow for controlled drug release over time.
Self-Assembly in Medical Nanofabrication
Self-assembly is a process where molecular components organize themselves into ordered structures without external direction. In medical applications, self-assembly is used to create nanoparticles for drug delivery and construction of scaffolds for tissue engineering.Key features of self-assembly include:
- Spontaneity: Components naturally organize themselves.
- Precision: Structures form with high precision due to molecular interactions.
- Adaptability: Structures can adapt to environmental changes, ideal for biological applications.
The mathematics of self-assembly can be described using potential energy landscapes, where the system seeks the lowest energy state. These concepts are modeled using equations that predict the stability and configuration of structures: Consider a simple system where potential energy is minimized as molecules assemble: \[ U(r) = -A \frac{{e^{-kr}}}{r} \] Where:
- U(r) is the potential energy as a function of distance r
- A is a constant representing the interaction strength
- k is another constant related to the term's decay
Did you know? Self-assembly processes are inspired by biological systems, such as DNA formation, where structures naturally form through base pairing and hydrogen bonding.
Soft Lithography in Medical Nanofabrication
Soft lithography is a versatile technique used for fabricating flexible and biocompatible surfaces. This method involves creating a mold with a patterned elastomer, often polydimethylsiloxane (PDMS), which is then used to shape materials at the nanoscale.Soft lithography is particularly advantageous for medical applications due to its:
- Flexibility: Capable of forming non-planar substrates.
- Biocompatibility: Suitable for use in biological environments.
- Cost-effectiveness: Offers a low-cost alternative to traditional lithography techniques.
Applications of Nanofabrication in Medicine
Nanofabrication plays a transformative role in medicine by enabling the development of innovative medical solutions. These applications focus on creating advanced drug delivery systems, diagnostic tools, and devices for regenerative medicine.
Drug Delivery Systems
Nanofabrication methods are instrumental in designing drug delivery systems that enhance the efficiency and specificity of treatments. These systems can deliver drugs directly to targeted cells, minimizing side effects and improving therapeutic outcomes.
Example: Targeted NanocarriersNanocarriers such as liposomes, dendrimers, and polymeric nanoparticles are engineered to transport and release medications at specific sites in the body. Targeting is achieved by functionalizing the nanoparticle surface with ligands that recognize and bind to specific cell markers.Such systems improve drug solubility and stability, offering enhanced control over release rates.
These smart drug delivery systems primarily utilize:
- Liposomes: Spherical vesicles with an aqueous core, often used to encapsulate hydrophilic drugs.
- Dendrimers: Branched macromolecules with voids for drug loading, and customizable surface groups for targeting.
- Polymeric Nanoparticles: Particles made from biocompatible polymers that degrade to release drug payloads.
Diagnostic Tools
Nanofabrication techniques are advancing diagnostic tools to achieve higher sensitivity and specificity. Nanosensors and lab-on-a-chip technologies are key innovations in early detection and monitoring of diseases.
Example: Lab-on-a-ChipLab-on-a-chip devices integrate multiple laboratory functions on a single chip, capable of handling extremely small fluid volumes. These devices are used for rapid analysis without the need for a full laboratory setup.By combining microfluidics with nanoscale sensors, these chips can analyze blood samples for biomarkers indicative of diseases such as diabetes and cancer.
Tip: Lab-on-a-chip technology not only speeds up diagnostics but also provides point-of-care testing, enabling immediate decision-making by healthcare professionals.
Regenerative Medicine
In the field of regenerative medicine, nanofabrication allows for the design of biomimetic scaffolds that support cell growth and tissue repair. These scaffolds are engineered to mimic natural extracellular matrices, guiding the regeneration of complex tissues.
The development of scaffolds utilizes techniques such as electrospinning, which produces fibrous structures promoting cell adhesion and proliferation.Electrospinning involves:
- Preparation of a polymer solution.
- Applying a high voltage to create a charge on the fluid surface.
- Formation of a jet that elongates and thins into nanofibers as it travels to a collector.
Advances in Nanofabrication Technology in Medicine
The rapid advances in nanofabrication technology have opened new frontiers in medicine, providing tools to engineer components with unprecedented precision. These technologies impact areas such as regenerative medicine, diagnostics, and targeted therapy. Critical advancements include development of biocompatible nanomaterials, integrated nanosensor devices, and personalized medicine solutions.
Fact: Nanofabrication technologies not only enhance existing therapies but also enable the discovery of entirely new treatment paradigms.
Recent progress in nanofabrication technologies involves:
- Biocompatible Nanomaterials: New materials are being created that integrate seamlessly into biological systems, minimizing immune responses while delivering therapeutic benefits.
- Nanosensors: Capable of detecting minute molecular changes, these sensors offer very early detection and monitoring of diseases, paving the way for personalized intervention strategies.
- Stem Cell Engineering: Using nanostructured surfaces to influence cell differentiation pathways, leading to advancements in tissue engineering.
Example: Nanosensors in Smart Drug DeliverySmart drug delivery systems now use nanosensors to provide feedback on the release and effectiveness of drugs, adjusting doses in real-time to maintain optimal therapeutic levels. Consider the feedback loop principle based in sensor data processing:The system can be modeled using a differential equation representing the drug delivery rate adjusted by sensor feedback:\[ \frac{dQ}{dt} = k_s (T_{desired} - T_{current}) \]Where:
- dQ/dt represents the change in drug amount.
- k_s is the sensitivity constant.
- T_{desired} is the desired therapeutic level.
- T_{current} is the current drug level.
nanofabrication methods - Key takeaways
- Definition of Nanofabrication Methods: Techniques used to construct and manipulate materials on the nanoscale, enabling development of complex devices with unique properties.
- Nanoscale and Photolithography: Nanofabrication operates at the atomic or molecular level (nanoscale), using methods like photolithography for semiconductor manufacturing.
- Medical Applications: Used for creating drug delivery systems, diagnostic tools, and tissue engineering applications, offering precision and targeted capabilities.
- Techniques in Medical Nanofabrication: Includes lithography for microneedles and nanoparticles, self-assembly for drug delivery, and soft lithography for flexible surfaces.
- Advances in Technology: Enhancements in biocompatible nanomaterials, nanosensors for detection and monitoring, and stem cell engineering improve regenerative medicine.
- Emerging Applications: Include smart drug delivery systems using nanosensors for real-time dosage adjustment and lab-on-a-chip devices for disease diagnostics.
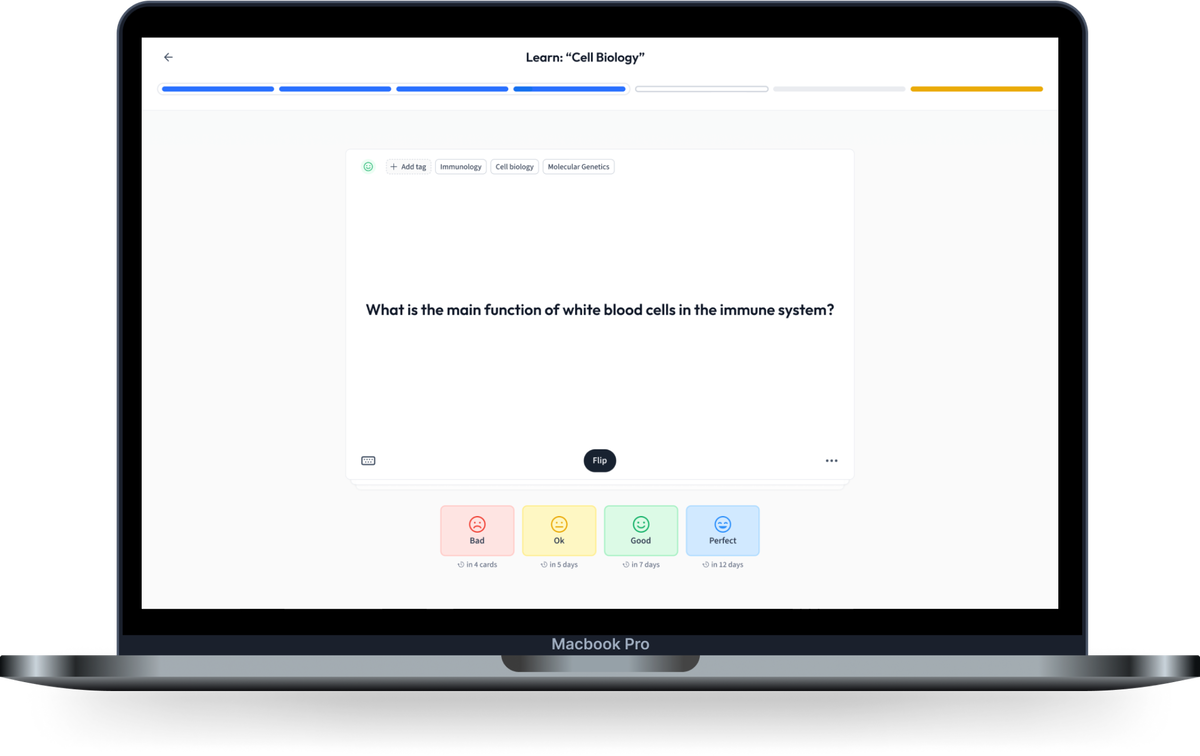
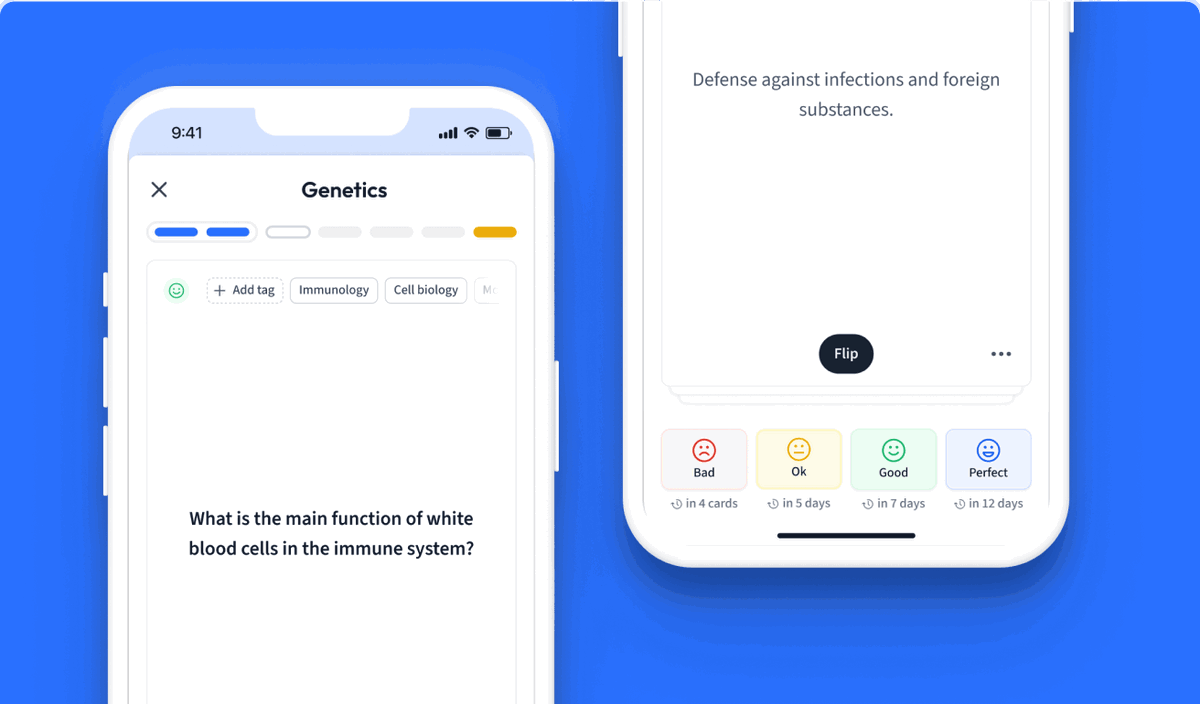
Learn with 12 nanofabrication methods flashcards in the free StudySmarter app
Already have an account? Log in
Frequently Asked Questions about nanofabrication methods
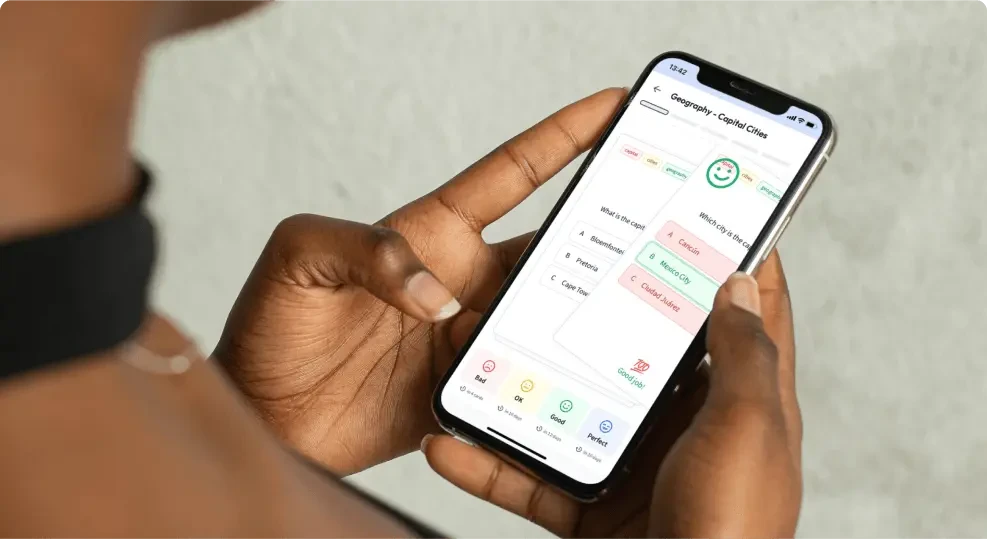
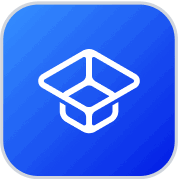
About StudySmarter
StudySmarter is a globally recognized educational technology company, offering a holistic learning platform designed for students of all ages and educational levels. Our platform provides learning support for a wide range of subjects, including STEM, Social Sciences, and Languages and also helps students to successfully master various tests and exams worldwide, such as GCSE, A Level, SAT, ACT, Abitur, and more. We offer an extensive library of learning materials, including interactive flashcards, comprehensive textbook solutions, and detailed explanations. The cutting-edge technology and tools we provide help students create their own learning materials. StudySmarter’s content is not only expert-verified but also regularly updated to ensure accuracy and relevance.
Learn more