Jump to a key chapter
Introduction to Nanoscale Fluid Dynamics
Nanoscale fluid dynamics is a captivating area of study that explores how fluids behave at the nanometer scale. This field of study is crucial for understanding various biological and technological processes at the microscopic level.
The Basics of Nanoscale Fluid Dynamics
Nanoscale fluid dynamics deals with the movement and interaction of fluid particles that are extremely small, often at the molecular or atomic level. At this scale, quantum effects and thermal fluctuations can significantly impact fluid behavior. Unlike macroscopic fluids, these interactions are not always predictable due to the large influence of surface forces compared to volumetric forces.
Nanoscale Fluid Dynamics refers to the study and application of fluid dynamics at a scale where nanoscale forces, behaviors, and effects are significant. It often involves interacting with individual molecules and particles within the fluid medium.
Consider the flow of water through a carbon nanotube. At this nanoscale, the water molecules can exhibit unique behaviors due to the tight spatial confinement and strong interaction with the tube's walls. This might not be observed in bulk fluid flow conditions.
Fluid dynamics at nanoscale often focus less on viscosity and more on surface tension and electrostatic interactions.
Mathematical Models in Nanoscale Fluid Dynamics
At the nanoscale, fluid dynamics require sophisticated mathematical models to account for the unique behaviors and forces at play. This includes considering interactions that might not be present in regular fluid dynamics. Some essential mathematical aspects include:
Nanoscale fluid dynamics often makes use of continuum mechanics, but traditional Navier-Stokes equations may need modification. Quantum effects can be considered using Schrödinger equation adaptations for fluid particles, while molecular dynamics simulations provide insights on particle interactions. Dimensional analysis becomes crucial, often outcomes are scaled using terms like the Reynolds number, but at a much smaller scope:
- The Reynolds number is typically much less than 1, indicating laminar flow where viscous forces dominate.
- The Knudsen number can also become significant, which compares the mean free path of particles to a characteristic length scale of the system.
In a typical flow analysis using the Navier–Stokes equation: \[\frac{d}{dt} \int_V \rho \mathbf{v} \, dV + \int_{\partial V} \rho \mathbf{v} \mathbf{v} \cdot \mathbf{n} \, dS = -\int_{\partial V} p \mathbf{n} \, dS + \int_{\partial V} \mathbf{T} \cdot \mathbf{n} \, dS + \int_V \rho \mathbf{f} \, dV\] At the nanoscale, additional quantum terms or corrections may be applied.
Applications of Nanoscale Fluid Dynamics
Nanoscale fluid dynamics plays a pivotal role in several cutting-edge applications. Understanding these applications can offer insight into the significance of this field. Some prevalent areas include:
The study of fluid dynamics at the nanoscale is essential for developing efficient drug delivery systems, as it helps in optimizing the distribution of nanoparticles in the human body for targeted treatment.
- Biomedical Engineering: In designing drug delivery systems, understanding how fluids behave at the nanoscale allows for precise targeting and efficient delivery of pharmaceuticals at the cellular level.
- Nanotechnology: Engineers use principles of nanoscale fluid dynamics to design microfluidic devices, which are used in various fields like biotechnology for tasks such as DNA sequencing.
- Environmental Science: This knowledge is applied to understand transport phenomena in porous media, crucial for developing filtration systems and sensors for detecting toxins at low concentrations.
Field | Application |
Biomedical | Targeted drug delivery |
Nanotechnology | Microfluidic device manufacturing |
Environmental Science | Water filtration |
Importance of Fluid Dynamics in Medicine
Fluid dynamics plays a vital role in medical science, particularly in understanding and treating cardiovascular diseases. Analyzing how blood flows through arteries and veins helps develop strategies for diagnosing and managing various heart conditions.
Cardiovascular Applications
The study of fluid dynamics is crucial in modeling blood flow within the cardiovascular system. This involves examining the mechanical properties of blood and the forces exerted by it on the vessel walls, which can indicate areas of potential blockage or weakness. The heart's pumping efficiency and how blood circulates can be mathematically represented by equations and principles derived from fluid dynamics.
Consider the use of the Bernoulli's equation in cardiology, which simplifies the analysis of blood flow: \[ P_1 + \frac{1}{2} \rho v_1^2 + \rho gh_1 = P_2 + \frac{1}{2} \rho v_2^2 + \rho gh_2 \] Here,
- P stands for pressure,
- ρ for density,
- v for velocity, and
- h for height.
Respiratory System and Fluid Dynamics
Applying fluid dynamics to the respiratory system assists in understanding airflow through the bronchi and alveoli. It allows the examination of gas exchanges and the effect of various diseases on breathing efficiency.
Reynolds Number is an important dimensionless quantity in fluid mechanics used to predict flow patterns: \[ Re = \frac{\rho v D}{\mu} \] Where
- ρ is the fluid density,
- v is the flow velocity,
- D is the characteristic length, and
- μ is the dynamic viscosity.
Advanced medical technology such as Computational Fluid Dynamics (CFD) is employed to simulate and analyze fluid behavior within the human body. For example, CFD models can simulate how air flows around the nasal cavity and assists in designing superior treatment strategies for respiratory conditions such as asthma or chronic obstructive pulmonary disease (COPD). These models take into account complex geometries of the airways and the variation in lung tissues which conventional studies might not be able to predict. With improved computational power, CFD is paving the way for personalized medicine, adapting treatments based on individual-specific characteristics derived from these simulations.
Techniques in Nanoscale Fluid Dynamics
Nanoscale fluid dynamics is a crucial field for understanding the behavior and properties of fluids at extremely small scales. This has been essential for advancements in various technologies and biological processes.
Understanding Liquid Mechanics at Nanoscale
The study of liquid mechanics at the nanoscale involves analyzing how intermolecular forces influence fluid behavior. At this scale, you must consider phenomena such as van der Waals forces and hydrogen bonding. These forces are significant compared to the bulk properties that dominate macroscopic fluid dynamics.
Van der Waals Forces: These are weak attractive forces between molecules that contribute significantly to nanoscale fluid behavior, especially when the distance between particles is minimal.
An example of liquid mechanics at the nanoscale is the movement of ionic liquids through nanometer-sized pores. Due to significant surface forces and reduced dimensions, these liquids can exhibit unique behaviors, such as increased viscosity or altered thermal conductance.
Consider the phenomenon of capillary action at the nanoscale. In larger systems, it explains how liquid can climb against gravity within narrow spaces. At the nanoscale, this is even more pronounced due to the relative increase of surface forces. The equation governing capillary rise, illustrated by: \[ h = \frac{2 \gamma \cos \theta}{\rho g r} \]
- \( h \) is the height to which the liquid rises.
- \( \gamma \) is the surface tension.
- \( \theta \) is the contact angle.
- \( \rho \) is the density.
- \( g \) is the gravitational constant.
- \( r \) is the radius of the tube.
Current Methods in Nanofluidics
Nanofluidics involves the study and use of fluid flow in tiny, nanometer-sized channels. In this field, scientists develop methods to manipulate small quantities of fluids to explore interactions at the atomic level. These methods are essential in applications such as lab-on-a-chip devices, DNA sequencing, and drug delivery systems.
The development of lab-on-a-chip devices fundamentally relies on understanding fluid dynamics at the nanoscale to enable miniaturized chemical and biological analyses.
Some highlighted techniques in nanofluidics include:
- Electrokinetic Manipulation: Utilizing electric fields to control the movement of charged particles within a fluid.
- Microvalves and Micropumps: Innovations that control fluid flow precisely at the micro and nano level.
- Molecular Dynamics Simulations: These computational models predict how particles in a fluid interact on a molecular level, providing insight into both theoretical and practical applications.
An example of computational nanofluidics is using molecular dynamics simulations to predict fluid flow in carbon nanotubes. These simulations can reveal important details like flow velocity and pressure distributions that are otherwise difficult to measure experimentally.
A leading area of research in nanofluidics involves exploring new materials with unique properties. One such development is the use of graphene in creating more efficient nanochannels.
Material | Usage | Benefits |
Graphene | Nanochannels | High conductivity and strength |
Silicon | Lab-on-chip structures | Ease of fabrication |
Polymers | Flexible microdevices | Cost-effective and versatile |
Application of Nanoscale Fluid Dynamics in Medicine
Nanoscale fluid dynamics has become an indispensable tool in medicine, offering insights into intricate biological systems and enabling cutting-edge treatment methods. By understanding how fluids behave at the nanoscale, medical professionals can improve diagnostic and therapeutic techniques.
Medical Advancements Through Nanofluidics
Nanofluidics plays a crucial role in the evolution of medicine, particularly in the development of drug delivery systems. By leveraging nano-sized channels, researchers can precisely direct drugs to target areas within the body, enhancing treatment efficacy and minimizing side effects.Nanoscale fluid dynamics also facilitates the design of biosensors capable of detecting diseases at early stages. These sensors can isolate and analyze biomolecules with high sensitivity, paving the way for improved diagnostic procedures.
Consider the use of nanofluidics in cancer treatment, where nanoparticles loaded with anticancer drugs are directed through the bloodstream to the tumor site. Due to their small size, these particles can penetrate challenging tissue environments that larger particles cannot, promoting effective drug delivery.
In the realm of pathogens detection, nanofluidic chips have shown great promise. These chips, operating on nanofluidic principles, can separate nucleic acids based on size and charge, aiding in the identification of viral DNA or RNA. The manipulation of fluids at the nanoscale enhances the isolation process, allowing for swift and accurate pathogen detection during epidemics or pandemics.
The precision of nanofluidics allows researchers to develop personalized medicine solutions, adapting treatment protocols to individual patients' needs.
Case Studies: Nanoscale Fluid Dynamics in Medical Practice
To truly grasp the impact of nanoscale fluid dynamics in medicine, reviewing specific case studies is enlightening. These studies demonstrate the versatility and potential of nanofluidic applications in solving complex medical issues.
Nanofluidics: The study and application of fluid flow in systems of nanometer dimensions, often involving the interaction and manipulation of individual molecules and particles.
A groundbreaking case study involves the use of nanofluidic channels to sort and sequence DNA strands. By navigating nanoscale capillaries, DNA fragments are separated based on their size and charge, leading to faster and more accurate genomic studies.
Implementing nanofluidics in medical devices can reduce the size and cost of lab equipment, making advanced diagnostics accessible in remote or developing areas.
In organ transplant surgeries, nanoscale fluid dynamics has significantly enhanced organ preservation techniques. By understanding how cryoprotective agents propagate through tissues at a microscopic level, researchers have developed methods to reduce ice crystal formation during freezing. This results in improved tissue integrity upon thawing, boosting the success rates of organ transplants.The introduction of nanofluidic techniques in artificial organ development is creating breakthroughs, where bioengineered tissues require precise nutrient and oxygen delivery systems, only feasible through the exact control afforded by nanoscale fluid dynamics.
nanoscale fluid dynamics - Key takeaways
- Nanoscale fluid dynamics is the study of fluid behaviors at the nanometer scale, with important quantum effects and thermal fluctuations due to surface forces.
- Incorporating techniques like continuum mechanics and molecular dynamics simulations modify traditional fluid dynamics equations to suit nanoscale fluid dynamics.
- Applications in medicine include optimized drug delivery systems, efficient distribution of nanoparticles, and biosensors for early disease detection.
- Nanoscale fluid dynamics contributes significantly to designing microfluidic devices and lab-on-a-chip technology in nanotechnology sectors.
- Key phenomena at this level include van der Waals forces, capillary action, and electrokinetic manipulation used in nanofluidics.
- Techniques in nanoscale fluid dynamics involve electrokinetic manipulation, molecular dynamics simulations, and use of advanced materials like graphene for innovative solutions.
Learn faster with the 12 flashcards about nanoscale fluid dynamics
Sign up for free to gain access to all our flashcards.
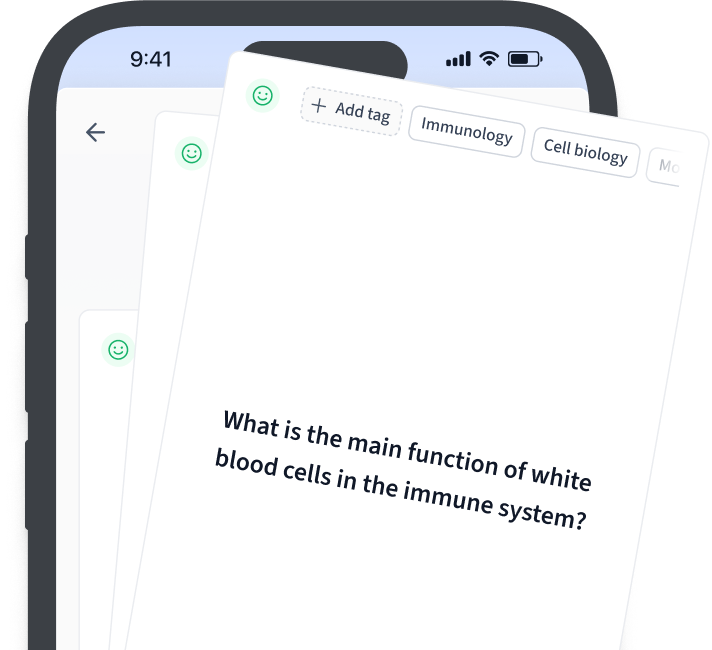
Frequently Asked Questions about nanoscale fluid dynamics
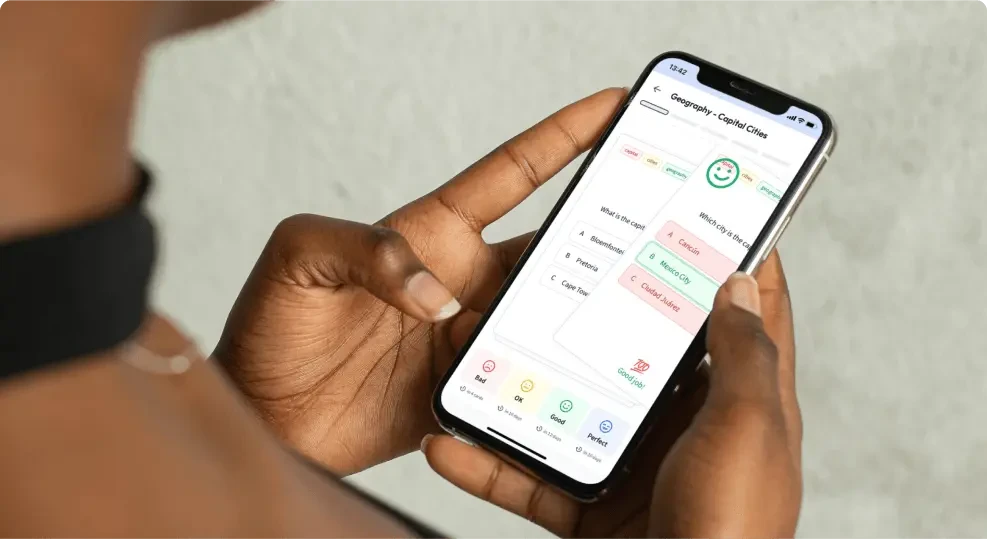
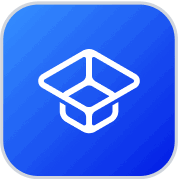
About StudySmarter
StudySmarter is a globally recognized educational technology company, offering a holistic learning platform designed for students of all ages and educational levels. Our platform provides learning support for a wide range of subjects, including STEM, Social Sciences, and Languages and also helps students to successfully master various tests and exams worldwide, such as GCSE, A Level, SAT, ACT, Abitur, and more. We offer an extensive library of learning materials, including interactive flashcards, comprehensive textbook solutions, and detailed explanations. The cutting-edge technology and tools we provide help students create their own learning materials. StudySmarter’s content is not only expert-verified but also regularly updated to ensure accuracy and relevance.
Learn more