Jump to a key chapter
Nanotechnology Tissue Engineering Basics
Nanotechnology tissue engineering is a rapidly growing field that combines biotechnology and nanotechnology to create structures and materials for repairing or replacing damaged tissues in the body. This interdisciplinary approach utilizes materials at the nanoscale to improve the properties and functionality of biomedical structures. Here, you will discover the fundamentals of this innovative field.
What is Nanotechnology in Tissue Engineering?
Nanotechnology involves manipulating matter at an atomic and molecular scale, specifically materials sized between 1 and 100 nanometers. Its application in tissue engineering allows for the design of nano-sized scaffolds that support cell growth and tissue repair. These scaffolds mimic the natural extracellular matrix, promoting cell adhesion, proliferation, and differentiation. In tissue engineering, nanotechnology can enhance the mechanical properties of scaffolds, making them more resilient and adaptable to the body's movements. Nano modifications can also improve the delivery of growth factors and drugs, allowing for more controlled and efficient treatments. The nanoscale offers a unique way to influence biological processes, enabling treatments for a variety of tissues, like skin, bone, and even organs.
Nanotechnology Tissue Engineering: The application of nanotechnology to improve tissue regeneration through advanced materials and techniques at the nanoscale.
Nanotechnology allows scaffolds to be highly porous, facilitating nutrient and waste exchange, which is essential for cell survival.
Applications of Nanotechnology in Tissue Engineering
Nanotechnology's integration into tissue engineering is transforming the scope and effectiveness of biomedical applications:
- Skin Tissue Repair: Nano-based materials are used to create artificial skin capable of water retention and elasticity, crucial for burn victims.
- Bone Regeneration: Nanostructured composites can mimic bone's natural mineralized structure, improving integration and healing in fractures.
- Drug Delivery Systems: Nano-sized particles can deliver drugs directly to the target area, reducing side effects and increasing treatment efficiency.
Consider the process of bone regeneration. By using a scaffold made of nano-hydroxyapatite, which mimics the mineral composition of bone, it is possible to enhance osteointegration. This not only allows for quicker healing but also improves the strength of the repaired bone.
The Science Behind Nanotechnology and Tissue Engineering
Understanding the scientific principles of nanotechnology is fundamental to its application in tissue engineering. The nanoscale phenomena, such as quantum mechanics, surface energy, and chemical reactivity, are crucial in designing effective biomaterials.
- Quantum Mechanics: At the nanoscale, you encounter unique electrical and optical properties, which are essential for developing responsive and adaptive materials.
- Surface Area to Volume Ratio: Due to its high surface area compared to volume, nanomaterials can interact more effectively with cells and proteins.
- Chemical Reactivity: Nano-sized particles have increased chemical reactivity, which can be harnessed to improve cell signaling pathways in tissue regeneration.
Scaffolds made from nano-biomaterials can improve vascularization, promoting faster and more effective tissue integration.
Let's delve deeper into the application of quantum mechanics in nanotechnology tissue engineering. At this scale, materials may exhibit quantum tunneling, where electrons pass through energy barriers, influencing electrical conductivity and enabling the creation of 'smart' biomaterials. These materials can detect and respond to changes, such as a change in pH or temperature, providing adaptive responses for more effective tissue engineering solutions. Additionally, by harnessing the unique properties of nanoparticles, researchers continue to explore ways to enhance imaging and diagnostic techniques, critical to understanding and monitoring tissue regeneration.
Application of Nanotechnology in Tissue Engineering
Nanotechnology is transforming tissue engineering by enabling the manipulation of materials at the nanoscale, offering groundbreaking opportunities for biomedical applications. This technology allows for the creation of advanced scaffolds and drug delivery systems that are critical in tissue regeneration.
Enhancing Tissue Scaffolds with Nanotechnology
Nanotechnology enhances tissue scaffolds, which act as a supportive matrix for cell growth and differentiation. By incorporating nanofibers, nanocomposites, and nanoparticles, scaffold structures mimic the natural extracellular matrix, promoting better cell interaction and growth.
- Nanocomposites improve scaffold's mechanical strength, making them suitable for load-bearing tissues.
- Nanofibers create a porous network facilitating nutrient flow and cellular migration.
- Nanoparticles enhance bioactivity by increasing scaffold surface area and interaction with biological molecules.
The porosity created by nanofibers is key to balancing strength and cell perfusion in tissue scaffolds.
Targeted Drug Delivery in Tissue Engineering
Nanotechnology facilitates targeted drug delivery within tissues, offering controlled and sustained release of therapeutic agents. This is vital in tissue engineering settings where localized delivery can minimize side effects and enhance treatment efficacy. Nanoparticles such as liposomes, micelles, and dendrimers can be loaded with drugs and engineered to release their payload in response to stimuli, such as pH changes or temperature variations.
For targeted cancer treatment, nanoparticles can be designed to release anticancer drugs when encountering the acidic environment of a tumor. This precision delivery system reduces harm to healthy tissues and increases drug concentration in the cancerous area.
Mathematical Modeling in Scaffold Design
The design of nanostructured scaffolds benefits from mathematical modeling, which helps predict and optimize their mechanical and biological properties. Mathematical equations guide the scaling and composition of nanomaterials to achieve desired outcomes. Consider the mechanical properties modelled by the equation: \[E_{\text{scaffold}} = f(E_{\text{nano}}, V_{\text{nano}}, \text{architecture})\] where \(E_{\text{scaffold}}\) is the scaffold's elastic modulus, \(E_{\text{nano}}\) the nanomaterial's modulus, and \(V_{\text{nano}}\) the volume fraction of the nanomaterial. Mathematical optimization aids in tailoring the scaffold properties for specific tissue engineering applications.
Delving deeper into the mathematical approaches, computational models simulate the biological environment and scaffold-tissue interactions. Models like finite element analysis (FEA) assess stress distribution and strain within scaffolds, ensuring they meet mechanical demands of the target tissue. Bioprinting, informed by these simulations, allows for precise scaffold fabrication, integrating nanoscale features that promote cellular function and tissue integration.
Biomaterials and Nanotechnology for Tissue Engineering
Biomaterials play a crucial role in tissue engineering, providing the 3D structure needed for tissue regeneration. When combined with nanotechnology, these materials can be crafted to mimic the body's natural tissues more closely.
Key Properties of Biomaterials
To be effective in tissue engineering, biomaterials must meet several requirements. These include biocompatibility, biodegradability, and suitable mechanical properties.
- Biocompatibility: The material should not provoke immune responses, ensuring it integrates well with human tissue.
- Biodegradability: It should degrade at a rate that matches the growth of new tissue, leaving no harmful residues.
- Mechanical Properties: The scaffold must withstand physical forces without losing structural integrity.
Property | Importance |
Biocompatibility | Prevents immune rejection |
Biodegradability | Ensures safe absorption |
Mechanical Strength | Supports tissue growth |
Advancements with Nanotechnology
Nanotechnology enhances biomaterials by introducing nanoscale features that improve their performance in tissue engineering applications. The increased surface area of nanomaterials supports better cell adhesion and growth. Furthermore, nanoparticles can introduce functional capabilities, such as delivery systems for growth factors, enhancing the scaffolds' regenerative potential. Incorporating nanotechnology into biomaterial scaffolds entails the integration of concepts from nanofabrication and nanomodification, providing tailored solutions for complex tissue engineering challenges.
Nanofabrication: The design and creation of structures with nanoscale dimensions that enhance material properties for targeted applications.
Consider using nanofibrous scaffolds for cartilage regeneration. The nanofibers' high surface area to volume ratio supports chondrocyte attachment, influencing cartilage matrix production by mimicking the nanostructure of natural cartilage.
Diving deeper into the application of nanotechnology, you can explore self-assembly techniques where biomaterials naturally form desired structures at the nanoscale. This approach allows for the creation of materials that self-organize into complex architectures, reducing the need for intricate manufacturing processes. Self-assembling peptides, for example, can form hydrogel scaffolds within the body, providing a supportive environment for cell adhesion and growth. Furthermore, computational models predict atomic-level interactions in these systems, optimizing their design for specific tissue engineering applications.
Mathematical Modeling in Scaffold Design
Mathematical modeling is integral to optimizing scaffold design, guiding the decision-making process for material selection and structural composition. Equations such as the elasticity formula: \[E = \frac{\text{stress}}{\text{strain}}\] are essential for predicting a scaffold's mechanical behavior. Advanced models can simulate environmental interactions, such as nutrient diffusion and cell migration, allowing for fine-tuning of scaffold characteristics.
The combination of machine learning with mathematical models in biomaterial design can accelerate discovery processes, enabling rapid development of new nanoscale biomaterials.
Nanotechnology in Bone Tissue Engineering
Nanotechnology is revolutionizing the field of bone tissue engineering by offering innovative solutions for bone repair and regeneration. The unique properties of nanomaterials facilitate the design of scaffolds that closely mimic the structural and mechanical properties of natural bone.
Techniques in Nanotechnology for Tissue Repair
Several cutting-edge techniques harness nanotechnology for tissue repair, enhancing the restoration of damaged or diseased tissues, particularly bone structures.
- Nanocomposite Scaffolds: These scaffolds incorporate nanoparticles to enhance strength and bioactivity, promoting better cell attachment and proliferation.
- Electrospinning: Produces nanofibrous meshes that mimic the extracellular matrix, supporting cell growth and nutrient exchange.
- Sol-gel Processing: A chemical method to synthesize nanostructured materials with controlled porosity for bone scaffolds.
Electrospinning: A technique that uses an electric field to produce extremely fine fibers from a polymer solution, creating structures that mimic the natural extracellular matrix.
Electrospun nanofibers have a high surface area, which can significantly enhance protein adsorption and cell interaction.
Examples of Nanotechnology in Tissue Engineering
Nanotechnology is applied extensively in various tissue engineering contexts beyond bone regeneration.
- Skin Tissue Engineering: Nanosilver particles are used for their antimicrobial properties, improving healing in skin grafts and wounds.
- Cardiac Repair: Nanoparticles facilitate the growth and repair of cardiac tissue by deliverin0g growth factors and stem cells.
- Nerve Regeneration: Nanofibrous scaffolds provide guidance cues for nerve regrowth, essential in nerve injury recovery.
In cardiac repair, nanoparticles can deliver oxygen to hypoxic cardiac tissues, enhancing cell survival and tissue regeneration. For instance, using perfluorocarbon nanoparticles can enhance oxygen solubility, improving myocardial repair.
Nanotechnology in Tissue Engineering and Regenerative Medicine
The incorporation of nanotechnology in regenerative medicine elevates the possibilities in tissue engineering by enabling advanced treatments that mimic and support natural healing processes.Key applications include:
- Stem Cell Delivery: Nanocarriers enhance the delivery and integration of stem cells in damaged tissues.
- Biocompatible Nanocoatings: Improve the integration of implants by modifying surface characteristics at the nanoscale.
- Nanoneedles: Used for precise, minimally invasive delivery of substances to specific cells and tissues.
In regenerative medicine, the application of nanotechnology involves processes like self-assembling peptides, which form hydrogels that adapt to environmental stimuli. These systems can control the release of growth factors, providing sustained therapeutic effects. Additionally, nanogel-based systems for drug delivery have shown promise in reducing systemic toxicity and improving the therapeutic index of drugs. Mathematical modeling aids these innovations by simulating tissue responses, optimizing scaffold designs for desired adaptability and response to biological cues.
nanotechnology tissue engineering - Key takeaways
- Nanotechnology in tissue engineering combines biotechnology and nanotechnology to create nanoscale structures for tissue repair and replacement.
- Nano-sized scaffolds mimic the extracellular matrix, supporting cell growth, adhesion, and differentiation, and enhance mechanical properties and drug delivery.
- Applications include skin tissue repair, bone regeneration, and targeted drug delivery systems using nanomaterials like nanoparticles, nanofibers, and nanocomposites.
- Techniques such as electrospinning, sol-gel processing, and mathematical modeling optimize scaffold design for tissue repair, particularly in bone reconstruction.
- Examples of nanotechnology in tissue engineering include using nanosilver particles for antimicrobial properties in skin grafts and nanofibrous scaffolds for nerve regeneration.
- In regenerative medicine, nanotechnology aids in stem cell delivery, enhances implant integration, and utilizes nanoneedles for precise substance delivery.
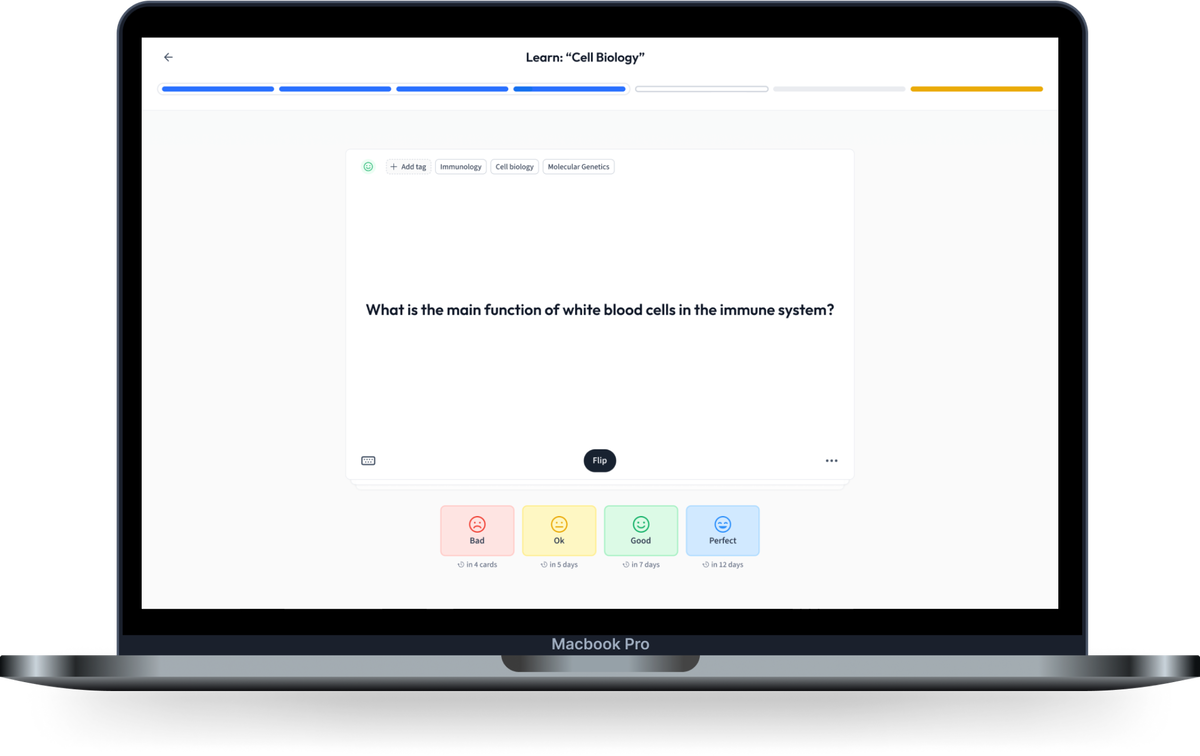
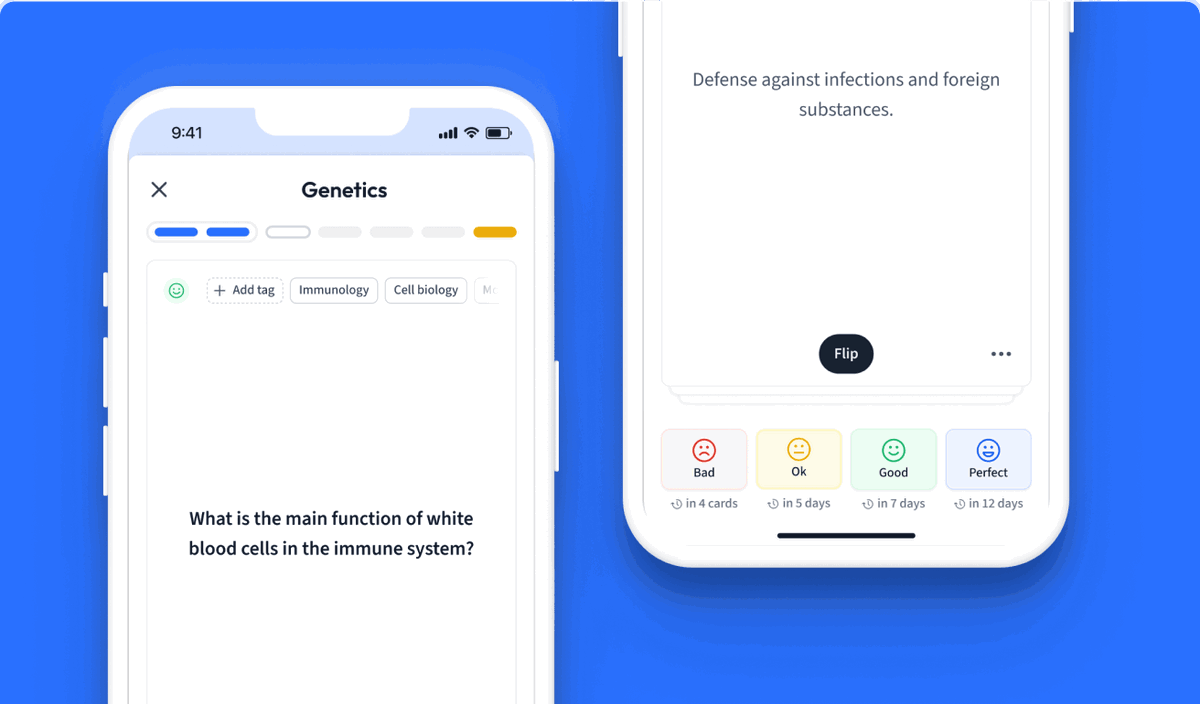
Learn with 12 nanotechnology tissue engineering flashcards in the free StudySmarter app
Already have an account? Log in
Frequently Asked Questions about nanotechnology tissue engineering
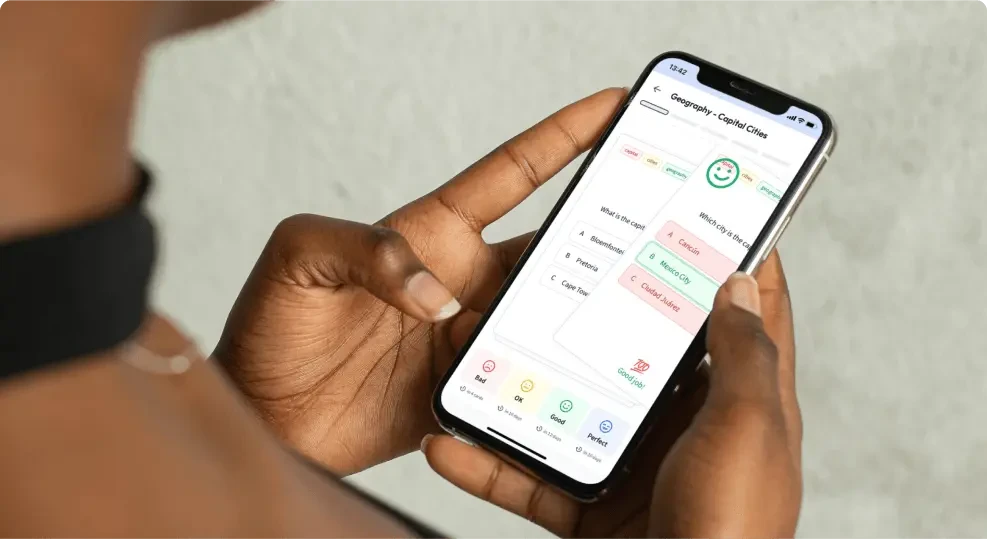
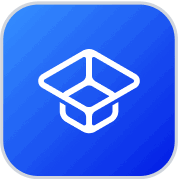
About StudySmarter
StudySmarter is a globally recognized educational technology company, offering a holistic learning platform designed for students of all ages and educational levels. Our platform provides learning support for a wide range of subjects, including STEM, Social Sciences, and Languages and also helps students to successfully master various tests and exams worldwide, such as GCSE, A Level, SAT, ACT, Abitur, and more. We offer an extensive library of learning materials, including interactive flashcards, comprehensive textbook solutions, and detailed explanations. The cutting-edge technology and tools we provide help students create their own learning materials. StudySmarter’s content is not only expert-verified but also regularly updated to ensure accuracy and relevance.
Learn more