Jump to a key chapter
Definition of Nanotopography in Medicine
Nanotopography refers to the specific surface features at the nanoscale, typically ranging from 1 to 100 nanometers, that influence biological and mechanical processes. In the medical field, understanding and manipulating these tiny surface details can lead to significant advancements in treatment and research strategies.
Understanding Nanotopography
In order to fully grasp the concept of nanotopography, it is essential to break down its elements:
- Scale: Operates at the nanometer level, permitting manipulation of materials and surfaces at atomic precision.
- Surface Features: Involves patterns, heights, and gradients, all of which can directly affect cell behavior.
- Impact: Critical in influencing protein adsorption, cell proliferation, and tissue integration.
Nanotopography: Surface characteristics at the nanoscale that affect biological interaction and behavior, important in medical application due to its precision and scalability.
Consider the example of an orthopedic implant: By engineering the surface nanotopography, developers can improve the integration of the implant with surrounding bone, reducing rejection rates and increasing the longevity of the implant.
One of the fascinating applications of nanotopography is in neuroscience. Here, researchers design nanoscale patterns to study how neurons grow and form networks. This understanding can potentially lead to breakthroughs in neurological disorder treatments, such as Alzheimer's and Parkinson's disease. The complex relationship between cell behavior and surface patterns provides insight into how to create specific responses. For example, certain nanotopographic features can lead to faster neuron growth or guide the direction of neural connections. Researchers continue to unravel the precise mechanisms through which these patterns exert their effects, which opens a promising frontier for regenerative medicine.
Importance of Nanotopography in Medical Research
The role of nanotopography in medical research cannot be overstated. Its precise control over surface interaction forms a cornerstone of innovation in various domains, including:
- Drug Delivery: Enhanced drug delivery mechanisms that target specific cells and tissues by using nano-surface modifications to improve efficiency and reduce side effects.
- Cell Culture Studies: Provides a more lifelike environment for cells growing in vitro, which is essential for reliable drug testing and regenerative medicine research.
- Implant Technology: Leads to improved biocompatibility of implants, minimizing complications such as infection or tissue rejection.
Did you know? The nanoscale is so small that it's hard to imagine—a nanometer is one billionth of a meter, thousands of times thinner than a human hair!
Nanotopography Influence on Cell Behavior
Nanotopography can have profound effects on how cells behave, interact, and develop. The interaction between cells and their environment is critical for numerous biological processes, including tissue regeneration, immune responses, and disease progression. Understanding these interactions can lead to innovations in biotechnology and medicine.
Interaction of Cells with Nanotopography
The interaction between cells and nanotopographic surfaces is complex yet crucial for several medical applications. These interactions are dictated by the following factors:
- Surface Texture: Different nanoscale patterns can affect the way cells orient, spread, and adhere.
- Material Composition: The chemical nature of the surface can enhance or deter cellular interactions.
- Mechanical Properties: Stiffness and elasticity at the nanoscale influence cell signaling and differentiation.
For instance, in wound healing applications, surfaces engineered with specific nanotopography can promote faster skin cell migration and organization. This can speed up the healing process by providing a more favorable environment for cell attachment and proliferation, acting as a scaffold for new tissue formation.
Cells 'feel' their environment at the nanoscale, and this 'feeling' can dictate their behavior and fate!
Examining nanotopographic influences sheds light on how to manipulate cellular behavior for therapeutic purposes. For example, in cancer research, understanding cell interaction with nanotopography can aid in developing surfaces that either encourage normal cell behavior or prevent cancer cell growth. By shaping the microenvironment precisely, researchers can potentially control malignant transformations. Nano-engineered surfaces are also being investigated for their ability to guide stem cell differentiation, offering hope for regenerating damaged tissues. The applications in this area are vast, reflecting the fascinating complexity of cellular interaction with microscopic environmental cues.
Effects of Nanotopography on Cell Adhesion and Growth
The effects of nanotopography on cell adhesion and growth are critical for developing biomaterials and implants. By manipulating nanoscale features, scientists can:
- Enhance Cell Adhesion: Specific patterns and surface chemistries can promote stronger and more stable cell attachment.
- Control Cell Growth: Surfaces can be engineered to either encourage or inhibit cell proliferation.
- Direct Cell Differentiation: Certain designs can signal cells to specialize into particular types.
Nanotopography Effect | Application |
Enhanced Adhesion | Improving implant integration |
Controlled Growth | Drug testing models |
Directed Differentiation | Stem cell therapies |
Adhesion: The process by which cells attach to a surface, which is critical for various biological and medical applications.
Nanotopography Techniques in Medical Research
Nanotopography has become a game-changer in medical research, offering the ability to manipulate surface features at an unprecedented scale. Different techniques have emerged to create surfaces with precise nanoscale features, paving the way for innovations in biomedical applications.
Common Nanotopography Techniques
Several techniques are frequently employed to create nanotopographic features on surfaces. Each method offers unique advantages and is suitable for different applications:
- Electron Beam Lithography (EBL): Utilizes focused electron beams to create patterns at very high resolutions, ideal for complex nanostructures.
- Atomic Force Microscopy (AFM): Employs a sharp tip to mold surface features, useful for both imaging and patterning.
- Self-Assembly: Based on the natural tendency of molecules to form ordered structures, it is often used to produce large-area nanostructures.
A deeper insight into electron beam lithography (EBL) reveals its capability to manipulate materials at almost atomic precision. This technique focuses on controlling the beam to adjust parameters like dosage and dwell time, affecting the final nanotopography. A notable application includes producing nanopatterned surfaces for studying neuronal adhesion, where EBL is instrumental in mimicking the cellular environment. The mathematical precision available through EBL is akin to the formula \left(a^2 + b^2 = c^2\right) known from geometry—a fundamental concept that governs the creation of nanoscale patterns by guiding beam paths.
Innovative Tools in Nanotopography
Research into innovative tools for nanotopography continues to evolve, offering fresh perspectives on fabricating nanostructured surfaces. Advances include:
- Nanoimprint Lithography (NIL): A cost-effective process that involves pressing a mold into a resist layer to transfer nanoscale patterns.
- Focused Ion Beam (FIB) Milling: Uses a beam of ions for precise material removal, allowing modification and analysis of surfaces at high resolutions.
- Multiphoton Lithography: Explores photopolymerizable resins to build three-dimensional nanostructures, valuable for creating complex biomedical devices.
Using nanoimprint lithography (NIL), researchers can fabricate biocompatible surfaces for cardiac cell growth. By imprinting specific nanotopographic patterns, they can improve the alignment and electrical coupling of cardiac cells, which is critical for developing functional cardiac tissues.
The real advantage of focused ion beam milling is its ability to not only create but also analyze and modify nanostructures in situ, providing comprehensive control over nanoscale features.
Effects of Nanotopography in Medicine
The influence of nanotopography on medicine is vast, introducing innovative methods to improve treatment and biocompatibility. Understanding these effects unlocks a new realm of possibilities in therapeutic applications and medical device design. By manipulating surfaces at the nanoscale, researchers can optimize interactions at the cellular level, which impacts everything from drug delivery systems to the integration of medical implants.
Case Studies on Nanotopography Influence
Numerous case studies highlight the profound impact of nanotopography on medical advancements. These studies demonstrate the practicality and benefits of fine-tuning surface structures for various applications.In an orthopedic implant study, researchers adjusted the implant's surface nanotopography to enhance bone cell adhesion. The results showed a significant reduction in implant rejection rates.Another case explored the use of nanopatterned surfaces in wound healing. By employing specifically designed nanotopographies, the surfaces promoted quicker skin cell migration and reduced healing times by up to 30%.In the field of drug delivery, a study demonstrated how nano-engineered carriers improved the targeting and release of chemotherapy agents, minimizing side effects and maximizing efficacy.
Consider the development of contact lenses with nanotopographic features. These lenses are designed to deliver drugs to the eye over a sustained period, providing a more efficient treatment for conditions such as glaucoma. Through precise surface manipulation, these lenses can control the release rate of medication, enhancing patient compliance and treatment outcomes.
In these case studies, the degree of influence nanotopography has on cellular interactions often correlates directly with the nanoscale precision achieved during fabrication.
Future Implications of Nanotopography in Medicine
Looking forward, nanotopography is poised to revolutionize medicine even further by advancing technologies and opening new research avenues. The ability to precisely control surface properties at the nanoscale will likely lead to the development of more sophisticated medical devices and personalized medicine solutions.
- Regenerative Medicine: Leveraging nanotopography to guide stem cell differentiation and promote tissue regeneration.
- Precision Medicine: Customizing nano-patterned surfaces to suit individual patients’ needs, improving treatment specificity and outcomes.
- Biodegradable Implants: Using nanotopography to control the rate of degradation, matching the healing rates of tissues to enhance recovery.
A promising area for future development is the intersection of nanotechnology and AI. By combining the precision of nanotopography with the predictive power of artificial intelligence, it's possible to create adaptive medical devices that respond to changes in a patient's condition in real-time. This integration could lead to the next generation of 'smart' implants that adjust their properties for optimal healing and support, marking a significant leap forward in personalized healthcare delivery. Such devices may use feedback loops that monitor healing progress and automatically modulate certain characteristics to enhance recovery.
nanotopography influence - Key takeaways
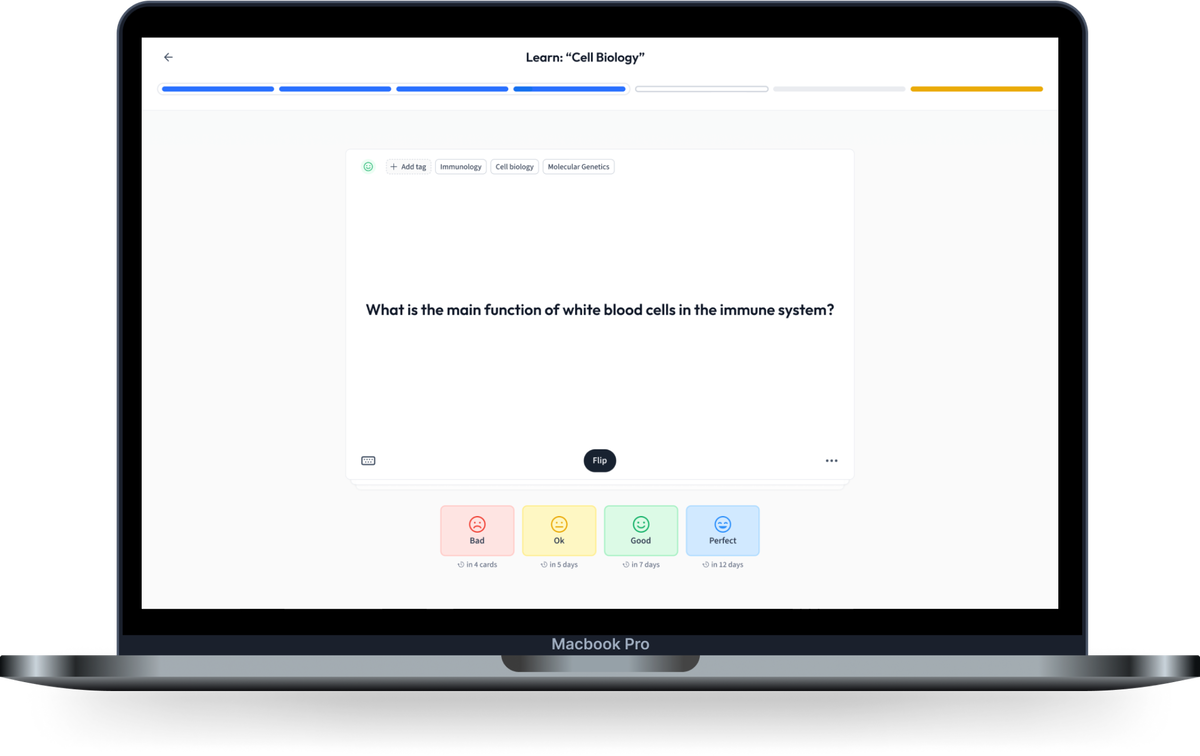
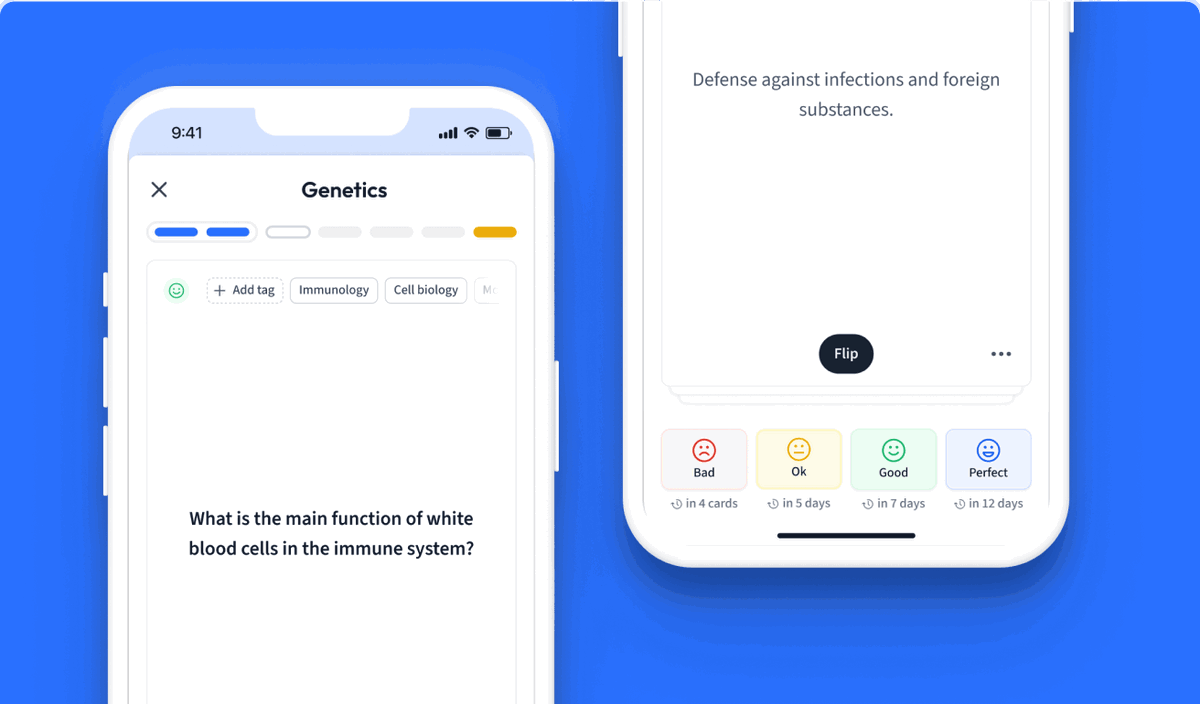
Learn with 12 nanotopography influence flashcards in the free StudySmarter app
Already have an account? Log in
Frequently Asked Questions about nanotopography influence
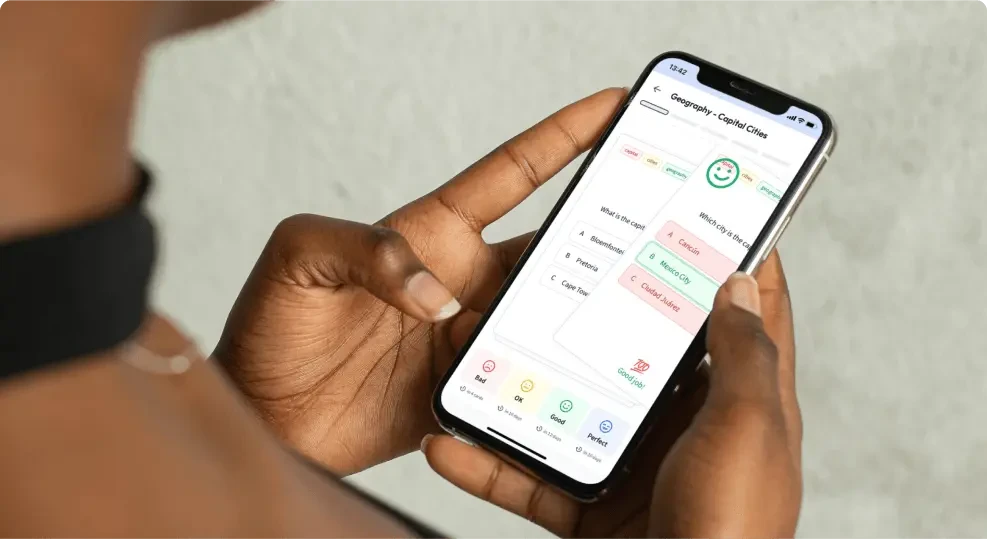
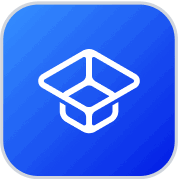
About StudySmarter
StudySmarter is a globally recognized educational technology company, offering a holistic learning platform designed for students of all ages and educational levels. Our platform provides learning support for a wide range of subjects, including STEM, Social Sciences, and Languages and also helps students to successfully master various tests and exams worldwide, such as GCSE, A Level, SAT, ACT, Abitur, and more. We offer an extensive library of learning materials, including interactive flashcards, comprehensive textbook solutions, and detailed explanations. The cutting-edge technology and tools we provide help students create their own learning materials. StudySmarter’s content is not only expert-verified but also regularly updated to ensure accuracy and relevance.
Learn more