Jump to a key chapter
Stem Cell Genomics Definition
Stem cell genomics is a fascinating and fast-evolving field that combines the study of stem cells with genomics, the science of understanding the complete set of DNA within an organism. It allows researchers to delve into the intricate genetic blueprints that command the behavior and development of stem cells. This examination has vast potential in medical applications, from regenerative medicine to understanding genetic diseases.
Stem cells are unique cells with the remarkable ability to develop into different cell types in the body during early life and growth. They serve as a repair system, dividing without limit to replenish other cells.
Genomics refers to the comprehensive study and analysis of genomes, which are the complete set of DNA, including all of its genes. Genomics aims to understand the structure, function, evolution, and mapping of genomes.
In stem cell genomics, scientists focus on the genetic makeup of stem cells, which encompasses their DNA sequences, gene expression patterns, and any genetic mutations. Through this study, you can learn how stem cells maintain their ability to become any cell type and understand factors that influence their differentiation.
Consider a case where a person's skin cells, which originate from stem cells, are genetically analyzed. By studying the genomics of these skin cells, scientists can identify specific genes responsible for skin regeneration and possibly even repair damaged tissue or treat skin-related disorders by manipulating these genes.
Understanding stem cell genomics can aid in creating personalized medicine strategies. It enables researchers to tailor medical treatments according to an individual’s genetic profile, potentially increasing the effectiveness and safety of treatments.
Scientists employ advanced technologies such as single-cell sequencing and CRISPR gene editing in stem cell genomics.
- Single-cell sequencing allows precise analysis of individual cells, helping researchers to understand the heterogeneity of stem cell populations in unprecedented detail.
- CRISPR facilitates targeted changes in the genome, enabling the study of gene functions and the development of therapies to correct genetic disorders.
Applications of Stem Cell Genomics in Regenerative Medicine
The integration of stem cell genomics into regenerative medicine is revolutionizing ways to heal and restore damaged tissues and organs. By harnessing the power of stem cells, medical researchers are developing novel therapies to repair or replace dysfunctional cells in a range of conditions.
Tissue Repair and Regeneration
Stem cell genomics provides insights into how stem cells can be directed to regrow damaged tissues. For instance, cells from the bone marrow, known as mesenchymal stem cells (MSCs), can potentially be guided to restore damaged cartilage in osteoarthritis patients. Understanding the genomics behind these processes enables the creation of targeted treatments that improve repair efficiency and reduce the risk of complications.
An example of this is in the treatment of heart disease. Stem cells are being genetically engineered to become heart muscle cells, with clinical trials showing promise in repairing heart muscle after a heart attack.
In a laboratory setting, researchers study the genetic pathways that govern stem cell differentiation. By manipulating these pathways, they can influence the cells' transformation into specific tissue types. This could lead to breakthroughs in treating diseases such as Parkinson's or type 1 diabetes, where certain cell types are damaged or deficient.
Development of Personalized Therapies
Through the insights gained from stem cell genomics, it's possible to craft personalized therapies that consider an individual's unique genetic makeup. By analyzing a patient's stem cells, researchers can identify potential risks and tailor interventions that are more effective and with fewer side effects. This personalized approach optimizes treatment suitability and improves outcomes.
Personalized therapies are a growing trend in medicine, providing a more tailored approach that fits precisely with a patient's genetic profile.
Drug Discovery and Development
Stem cell genomics is also an influential tool in drug discovery. Scientists can use patient-derived stem cells to create in vitro disease models that reflect the patient's disease phenotype. This enables the screening of drug effectiveness and safety in a personalized context. Moreover, genomics technology can pinpoint genetic targets for new medications, accelerating the development process.
In cancer medicine, patient-specific cancer stem cells are being used to test anti-cancer drugs, allowing for patient-specific treatment options based on the genetic profile of their cancer.
The pharmaceutical industry is increasingly investing in stem cell genomics to understand the complex interactions between drugs and human cells. With the aid of comprehensive genetic analyses, researchers can identify biomarkers that predict drug responses, paving the way for more effective therapeutic interventions. Furthermore, this genetic information can highlight candidates for drug repurposing, thus reducing the time and cost associated with bringing new drugs to market.
Stem Cell Genome Editing and Techniques
Stem cell genome editing revolutionizes the study and application of stem cells by allowing precise modifications of the genetic material. Techniques such as CRISPR Cas9 have emerged as powerful tools for altering genes within stem cells, providing new insights into developmental biology and potential therapy avenues.
CRISPR Cas9 Genome Editing in Embryonic Stem Cells
Embryonic stem cells (ESCs) are pluripotent cells derived from the early-stage embryo, possessing the ability to differentiate into any cell type. The introduction of CRISPR Cas9 to ESCs allows researchers to create targeted mutations, facilitating the study of gene function and disease modeling. By editing the genome of ESCs, scientists can investigate developmental processes and pathological mechanisms in a controlled lab environment.CRISPR Cas9 functions by creating double-strand breaks at specific genomic locations, guided by a short RNA sequence. Once a break occurs, cells naturally repair the damage through non-homologous end joining or homologous recombination, potentially introducing mutations or inserting new genetic material.
For example, gene function in mouse models is studied by employing CRISPR Cas9 to knock out genes in mouse embryonic stem cells. By observing the consequences of gene disruption, you can infer the role of these genes in development and disease.
CRISPR systems are derived from bacterial immune defense mechanisms, originally designed to protect against viral infections.
One fascinating application is the creation of organoids, which are miniaturized and simplified versions of organs. By editing the genes within ESCs, researchers can study how specific genetic alterations influence organ development. This can lead to remarkable insights into human organ physiology and potential treatment options for organ-specific diseases.
CRISPR Cas9 Genome Editing in Human Hematopoietic Stem Cells
Human hematopoietic stem cells (HSCs) give rise to all other blood cells and represent a crucial component in the development of blood-related therapies. Utilizing CRISPR Cas9 on HSCs opens up opportunities to correct genetic disorders such as sickle cell anemia and thalassemia directly at the hematopoietic progenitor level. By precisely altering defective genes in HSCs, you can pave the way for curing these hemoglobinopathies.CRISPR Cas9 allows for the targeted repair of mutations. For instance, in the case of sickle cell disease, a single point mutation in the beta-globin gene can be corrected in HSCs, thus potentially curing the disease.
Successful repairs have been demonstrated where CRISPR Cas9 corrected the point mutation responsible for sickle cell anemia in HSCs ex vivo, offering proof-of-concept for potential curative therapies.
Ensuring the safety of genome editing in HSCs requires comprehensive evaluation to avoid off-target effects. Researchers meticulously screen potential cut sites before choosing gene targets. After editing, rigorous assessments are conducted to confirm the exclusivity of the edit. The therapeutic promise of HSC genome editing combines the precision of CRISPR technology with the innate ability of stem cells to regenerate and repair blood cells. This could offer transformative advantages over traditional therapies that manage symptoms rather than address the underlying genetic cause.
Genomics of Stem Cell Differentiation
Understanding the genomics of stem cell differentiation is pivotal to unlocking their potential in various medical fields. Differentiation is the process by which a stem cell becomes a more specialized cell type. Through studying their genomics, you can decipher the molecular mechanisms that direct this transformation, which is essential for both fundamental biology and therapeutic applications.
Molecular Mechanisms of Differentiation
During differentiation, specific genes are activated or silenced, leading to the development of distinct cell types. Key molecular mechanisms responsible for this include:
- Gene expression regulation: Involves transcription factors that bind to DNA and influence the transcription of genes.
- Epigenetic modifications: Chemical changes to DNA or histones that affect chromatin structure and gene accessibility without altering the DNA sequence.
- Signaling pathways: Cascades of cellular signals that promote or inhibit differentiation, such as the Wnt and Notch pathways.
Differentiation is the process through which a less specialized cell becomes a more specialized cell type. This involves a series of genetic and epigenetic changes in the cell.
To illustrate, consider neural differentiation: stem cells differentiate into neurons through the downregulation of pluripotency genes and the activation of neuron-specific transcription factors. By understanding these genomic changes, scientists can refine protocols for creating neurons in vitro for research and therapy.
Gene expression changes during differentiation are not random but follow a highly orchestrated pattern that ensures the correct cell type forms.
Epigenetic Regulation in Stem Cell Differentiation
Epigenetic regulation plays a critical role in stem cell differentiation. This includes modifications such as DNA methylation and histone acetylation, which can activate or repress gene expression without altering the genetic code itself. These modifications are reversible, allowing for dynamic changes during differentiation.
Epigenetic Modification | Effect |
DNA Methylation | Gene silencing by adding methyl groups to DNA |
Histone Acetylation | Gene activation by adding acetyl groups to histones |
Histone Methylation | Can either activate or repress genes depending on the context |
Researchers have discovered that during differentiation, specific chromatin regions undergo methylation changes. For example, pluripotency genes are often marked by DNA methylation during differentiation, effectively silencing them. This ensures that once a stem cell commits to a certain lineage, it maintains its new identity and does not revert to a pluripotent state. Furthermore, these epigenetic changes are heritable, meaning that they can be passed down through cell generations, stabilizing the differentiated state. By manipulating these epigenetic marks, scientists can improve control over stem cell differentiation processes, which is crucial for developing cell-based therapies.
stem cell genomics - Key takeaways
- Stem Cell Genomics Definition: The study of the complete set of DNA within stem cells, focusing on their genetic blueprints, gene expression patterns, and mutations.
- Applications in Regenerative Medicine: Utilization of stem cell genomics to develop therapies for repairing damaged tissues and creating personalized medical treatments.
- Stem Cell Genome Editing: CRISPR Cas9 enables precise modification of stem cell genes, used for understanding gene functions and therapeutic developments.
- CRISPR Cas9 in Embryonic Stem Cells: Targeting and editing genes in embryonic stem cells to study developmental processes and disease mechanisms.
- CRISPR Cas9 in Human Hematopoietic Stem Cells: Corrects genetic disorders by altering defective genes in blood-forming stem cells, aiming for curative treatments.
- Genomics of Stem Cell Differentiation: Studies the molecular mechanisms of how stem cells become specialized cell types, crucial for therapeutic applications.
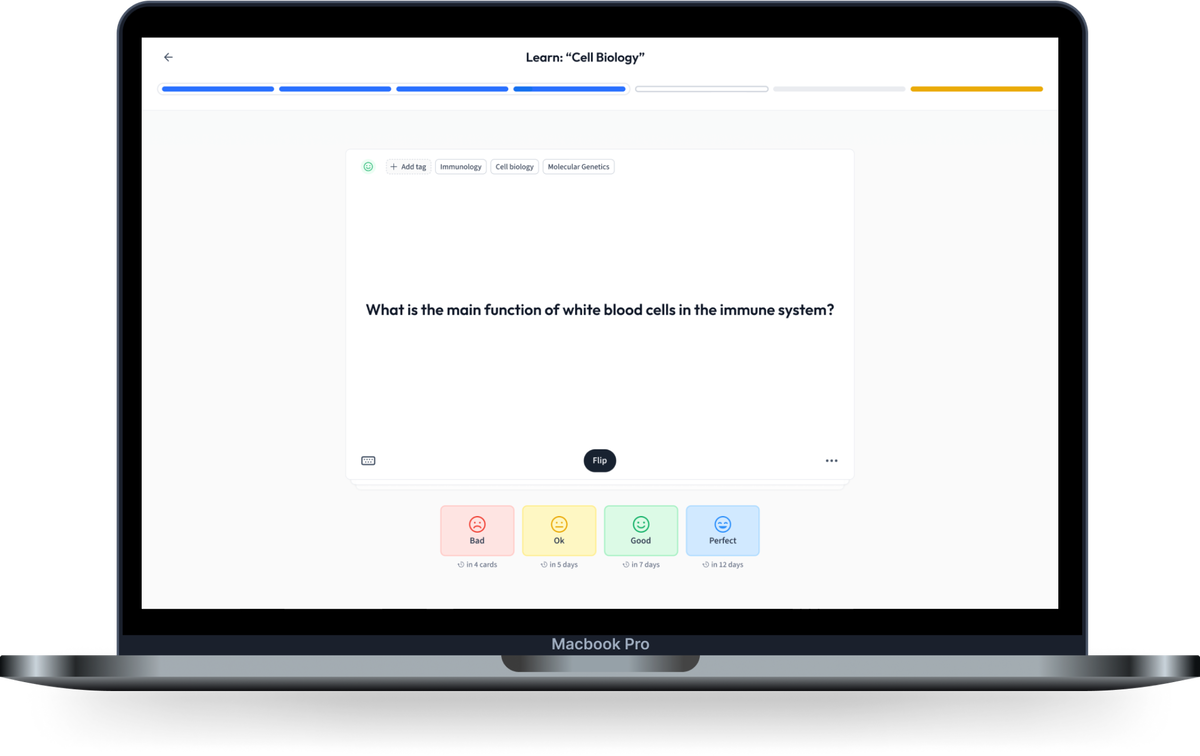
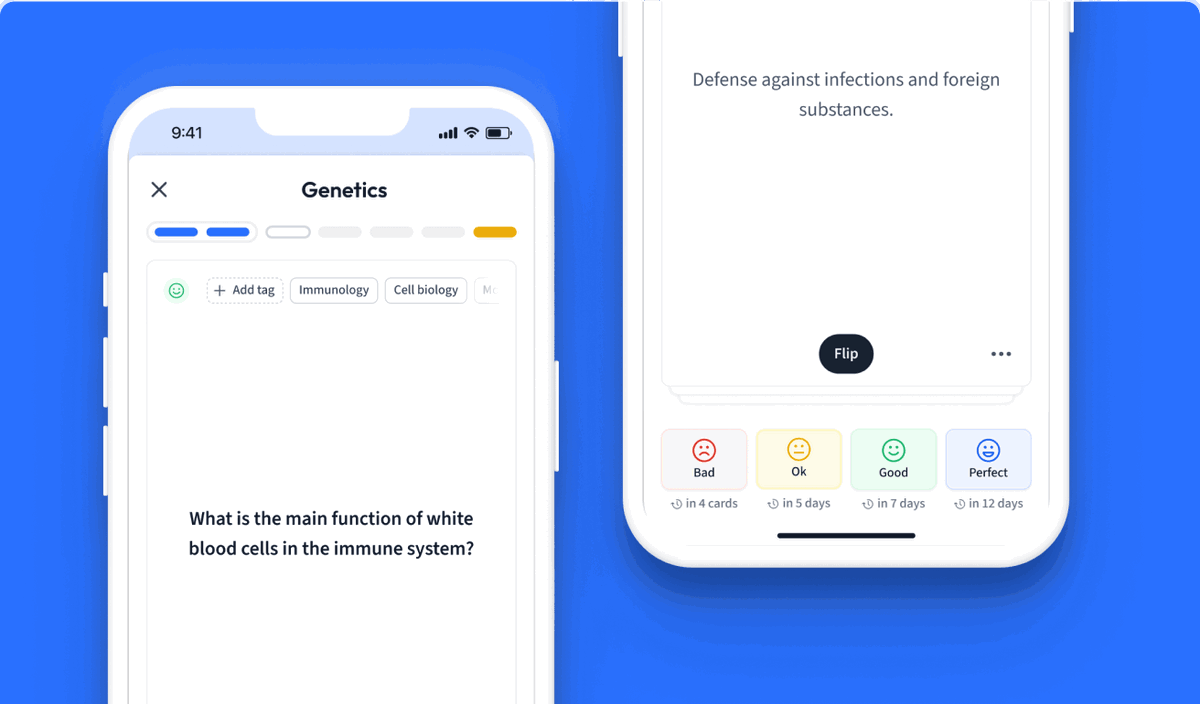
Learn with 12 stem cell genomics flashcards in the free StudySmarter app
Already have an account? Log in
Frequently Asked Questions about stem cell genomics
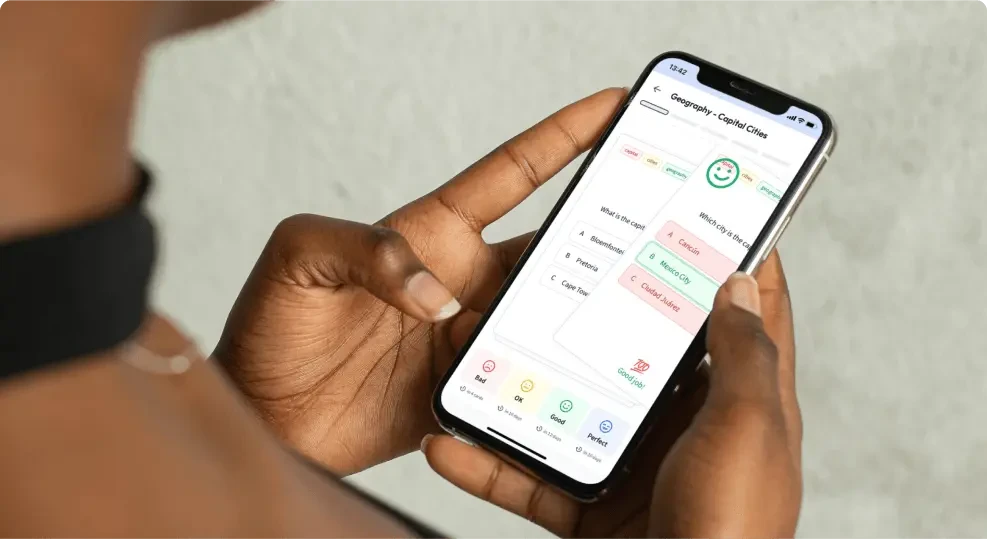
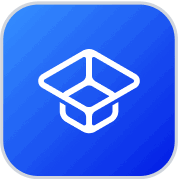
About StudySmarter
StudySmarter is a globally recognized educational technology company, offering a holistic learning platform designed for students of all ages and educational levels. Our platform provides learning support for a wide range of subjects, including STEM, Social Sciences, and Languages and also helps students to successfully master various tests and exams worldwide, such as GCSE, A Level, SAT, ACT, Abitur, and more. We offer an extensive library of learning materials, including interactive flashcards, comprehensive textbook solutions, and detailed explanations. The cutting-edge technology and tools we provide help students create their own learning materials. StudySmarter’s content is not only expert-verified but also regularly updated to ensure accuracy and relevance.
Learn more