Jump to a key chapter
Stem Cell Plasticity Definition
Stem cell plasticity refers to the remarkable ability of stem cells to differentiate into a wide range of cell types, allowing them to contribute to tissue repair and regeneration throughout the body. Understanding this concept is pivotal in the field of regenerative medicine.
Stem cell plasticity is the ability of stem cells, especially multipotent and pluripotent stem cells, to develop into various specialized cell types beyond their expected lineage, enabling potential applications in therapies and research.
Types of Stem Cells
You will come across several types of stem cells when exploring their plasticity:
- Totipotent stem cells: These have the ability to differentiate into any cell type, including extraembryonic or placental cells.
- Pluripotent stem cells: Capable of giving rise to almost any cell type, but not extraembryonic tissues.
- Multipotent stem cells: These can develop into multiple, but limited, cell types related to a specific lineage.
- Unipotent stem cells: Specialize in generating a single cell type, although they retain the capability of self-renewal.
Consider hematopoietic stem cells found in the bone marrow. These are multipotent, meaning they can differentiate into various blood cell types such as red blood cells and white blood cells, demonstrating their essential role in maintaining healthy blood and immune systems.
Applications in Medicine
The concept of stem cell plasticity opens up diverse potential applications in medicine. Here are some key areas:
- Regenerative Medicine: Using stem cells to repair or replace damaged tissues and organs.
- Gene Therapy: Correcting genetic defects by integrating functional genes into stem cells.
- Drug Testing: Producing specific cell types from stem cells to test drug efficacy and toxicity.
- Treatment of Degenerative Diseases: Targeting disorders such as Parkinson's disease and diabetes by replenishing lost cells through differentiation.
Stem cells' potential in creating diverse cell types hinges on their environment or niche. The niche comprises various signals and factors that influence the behavior and fate of stem cells. These signals direct the transformation of uncommitted stem cells into specialized ones, demonstrating the dynamic interaction between stem cells and their surroundings. Research is ongoing to replicate specific niches in laboratories to optimize stem cell therapies and to better understand how these environments control cell fate.
Stem cells have the potential to significantly extend the human lifespan by treating chronic diseases and injuries, showcasing the transformative power of stem cell plasticity.
Mechanisms of Stem Cell Plasticity
Understanding the mechanisms of stem cell plasticity is crucial for harnessing their potential in medical applications. These mechanisms involve complex processes that determine how stem cells differentiate and develop into specialized cells.
Molecular Signaling Pathways
Stem cell behavior is greatly influenced by molecular signaling pathways, which serve as communication channels within cells. They are responsible for transferring signals from the outside environment into the cell's nucleus, prompting changes in gene expression. Key pathways include:
- Notch signaling: Plays a role in maintaining stem cell's undifferentiated state and controlling their fate.
- Wnt signaling: Essential for cell proliferation and differentiation.
- Hedgehog signaling: Regulates stem cell growth and tissue regeneration.
Signaling pathways are systems of communication that govern basic cellular activities and coordinate cell actions to ensure proper function and adaptation.
Epigenetic Modifications
Epigenetic modifications refer to chemical changes in DNA and proteins that do not alter the DNA sequence but influence gene activity. Such modifications can activate or suppress genes required for differentiation:
- DNA methylation: Inhibits gene expression and is vital in cell fate decisions.
- Histone modification: Alters chromatin structure, affecting how DNA is read by the cell.
Recent studies highlight the reversible nature of epigenetic modifications, suggesting that even fully-differentiated cells might regain stem cell-like properties by resetting their epigenetic marks. This potential opens new avenues for tissue regeneration and personalized medicine, demonstrating the extensive influence of epigenetic regulation on stem cell plasticity.
Role of Microenvironment
The microenvironment, or stem cell niche, provides the necessary support for stem cell survival and function. This environment comprises various cues influencing stem cell behavior:
- Cell-to-cell interactions: Direct physical contacts influence stem cell fate decisions.
- Extracellular matrix: Provides structural support and transmits chemical signals.
- Soluble factors: Proteins and ions in the niche can dictate stem cell differentiation paths.
In the case of neural stem cells, their niche contains factors like growth hormones that guide them to develop into different types of neurons or support cells, showcasing the powerful influence of the microenvironment.
Researchers are developing synthetic niches in laboratories to study stem cell behavior in controlled environments, which can help refine therapeutic approaches.
Examples of Stem Cell Plasticity
Exploring examples of stem cell plasticity can provide you with a clear understanding of how stem cells contribute to tissue repair and regeneration by transforming into various specialized cells. Let's delve into some specific cases that illustrate this phenomenon.
Neural Stem Cells
Neural stem cells, found in the brain, showcase remarkable plasticity. These multipotent cells can differentiate into three primary cell types of the nervous system: neurons, astrocytes, and oligodendrocytes.
- **Neurons:** Transmit and process information in the nervous system.
- **Astrocytes:** Support and maintain the blood-brain barrier, repair brain and spinal cord following traumatic injuries.
- **Oligodendrocytes:** Produce myelin sheath to insulate axons for better electric signal transmission.
An example of neural stem cell plasticity is demonstrated when these cells are transplanted into damaged neural tissue, where they differentiate into the required cell types to aid regeneration and repair, potentially offering therapeutic solutions for conditions like spinal cord injuries and neurodegenerative diseases.
Mesenchymal Stem Cells
Mesenchymal stem cells (MSCs), found in the bone marrow, adipose tissue, and umbilical cord tissue, have the flexibility to differentiate into various cell types such as osteoblasts, chondrocytes, and adipocytes.
- **Osteoblasts:** Cells that form new bone.
- **Chondrocytes:** Cells that form cartilage.
- **Adipocytes:** Cells that store fat.
Mesenchymal stem cells have immune-privileged properties, making them suitable candidates for allogenic transplantation, where donor and recipient are not genetically identical.
Hematopoietic Stem Cells
Hematopoietic stem cells (HSCs) are responsible for the formation of blood cells and are valuable in treatments for blood-related disorders. These multipotent cells can differentiate into various types of blood cells, such as:
- **Red blood cells**: Carry oxygen from the lungs to the rest of the body.
- **White blood cells**: Play a critical role in the immune response.
- **Platelets**: Help in blood clotting and wound healing.
When discussing stem cell plasticity, it is critical to mention the emerging area of induced pluripotent stem cells (iPSCs). Scientists can reprogram differentiated cells back to a pluripotent state, allowing them to give rise to any cell type of the body. This technology holds promise for personalized treatments without the ethical issues associated with embryonic stem cells. The ability to generate patient-specific iPSCs could revolutionize transplant medicine, potentially bypassing immune rejection issues and increasing the success rates of regenerative therapies.
Adult Stem Cell Plasticity
Adult stem cell plasticity is a pivotal concept in regenerative medicine and remarkable for its ability to enable adult stem cells to transform into multiple cell types beyond their original niche, contributing to tissue repair and regeneration.
Plasticity in Stem Cells Research
Stem cell research has advanced significantly, shedding light on the underlying mechanisms of adult stem cell plasticity. Researchers are largely focused on understanding how these cells can be induced to change into various cell types, a quality that holds great promise for therapeutic applications.
- Investigating signaling pathways that regulate plasticity.
- Exploring epigenetic changes that enable cellular transformation.
- Developing laboratory techniques to mimic natural niches for controlled differentiation.
An example of research into plasticity is the exploration of hematopoietic stem cells which under the right conditions can transform into non-hematopoietic cells such as hepatocytes, the primary cells in the liver. This transformation suggests potential treatments for liver diseases using bone marrow-derived cells.
In recent years, the ability to generate induced pluripotent stem cells (iPSCs) from adult somatic cells has revolutionized the understanding of stem cell plasticity. iPSCs are created by reprogramming adult cells to a pluripotent state, in which they can differentiate into any cell type, similar to embryonic stem cells. This technology not only bypasses the ethical issues associated with using embryonic cells but also provides a platform for personalized medicine. Large-scale biobanks of iPSCs derived from diverse populations are being created to facilitate research into genetic diseases and develop tailored therapies.
Stem Cell Plasticity Mechanisms and Applications
The mechanisms underlying stem cell plasticity are integral to unlocking their full therapeutic potential. These mechanisms involve complex interactions between genetic, epigenetic, and environmental factors that guide stem cells toward differentiation.
- Gene expression: Regulation of specific genes determines cell fate.
- Epigenetic modifications: Alter chromatin structure, affecting accessibility of genes.
- Interaction with niche: Microenvironmental signals provide cues for differentiation.
Epigenetic modifications refer to reversible changes in gene expression without altering the DNA sequence, playing a crucial role in stem cell differentiation and function.
Adult stem cell therapy is advancing towards clinical applications, with ongoing trials for conditions such as heart disease, diabetes, and neurodegenerative disorders.
Applications of stem cell plasticity extend across various domains:
- Regenerative medicine: Repairing damaged tissues and organs.
- Drug discovery: Generating specific cell types for testing drug efficacy.
- Gene therapy: Correcting genetic defects on a cellular level.
stem cell plasticity - Key takeaways
- Stem cell plasticity definition: The ability of stem cells to differentiate into a wide range of cell types beyond their expected lineage, crucial for tissue repair and regenerative medicine.
- Mechanisms of stem cell plasticity: Involves molecular signaling pathways, epigenetic modifications, and microenvironmental interactions that guide stem cell differentiation.
- Examples of stem cell plasticity: Neural stem cells transform into neurons, astrocytes, and oligodendrocytes; mesenchymal stem cells differentiate into osteoblasts, chondrocytes, and adipocytes; hematopoietic stem cells form various blood cell types.
- Adult stem cell plasticity: The capability of adult stem cells to differentiate into multiple cell types, contributing to tissue repair and regeneration. Key area of research in regenerative medicine.
- Plasticity in stem cells research: Focuses on understanding and manipulating signaling pathways, epigenetic changes, and simulating niches to induce stem cell transformation.
- Stem cell plasticity mechanisms and applications: Includes gene expression, epigenetic modifications, interactions with niches, and applications in regenerative medicine, drug discovery, and gene therapy.
Learn faster with the 12 flashcards about stem cell plasticity
Sign up for free to gain access to all our flashcards.
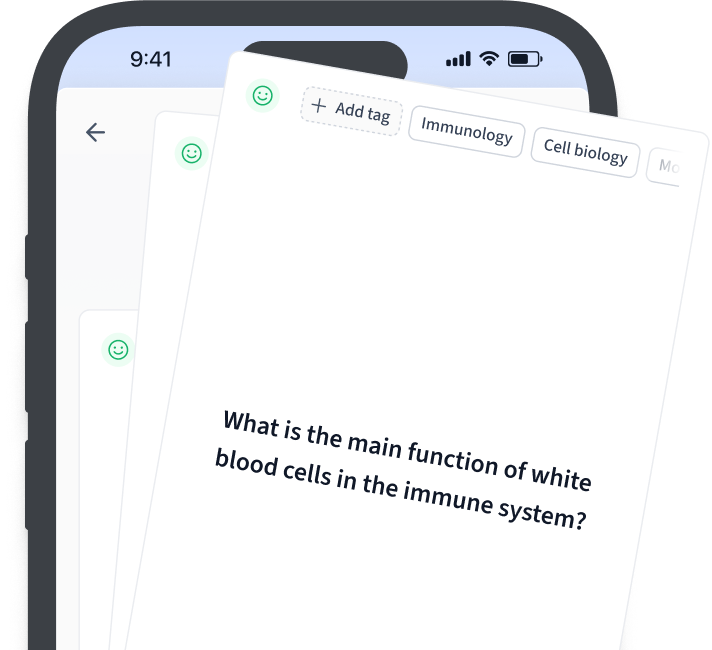
Frequently Asked Questions about stem cell plasticity
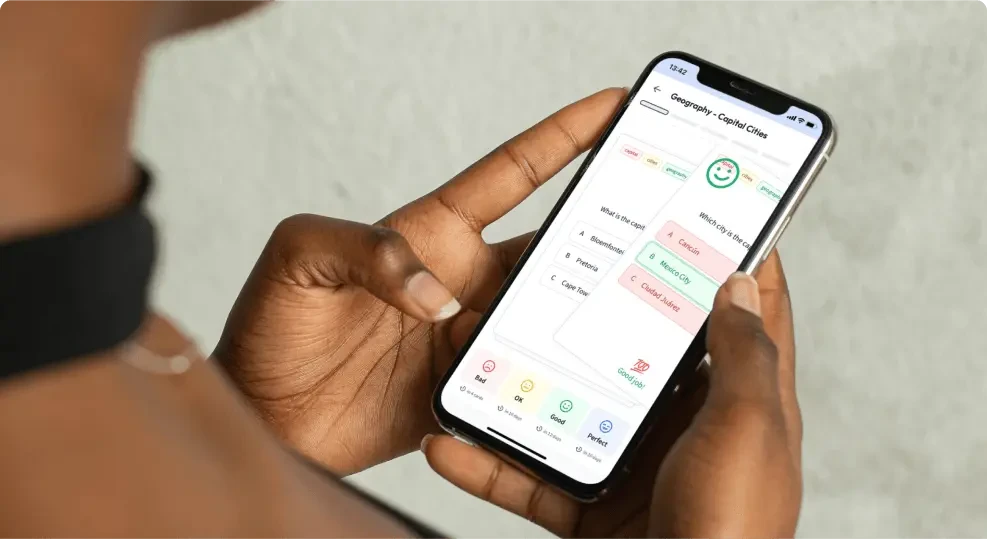
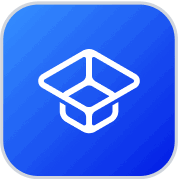
About StudySmarter
StudySmarter is a globally recognized educational technology company, offering a holistic learning platform designed for students of all ages and educational levels. Our platform provides learning support for a wide range of subjects, including STEM, Social Sciences, and Languages and also helps students to successfully master various tests and exams worldwide, such as GCSE, A Level, SAT, ACT, Abitur, and more. We offer an extensive library of learning materials, including interactive flashcards, comprehensive textbook solutions, and detailed explanations. The cutting-edge technology and tools we provide help students create their own learning materials. StudySmarter’s content is not only expert-verified but also regularly updated to ensure accuracy and relevance.
Learn more