Jump to a key chapter
Structural Biochemistry Definition
Structural biochemistry is a branch of biology that focuses on the molecular structures of biological macromolecules, particularly proteins and nucleic acids, and how changes in their structures affect their functions within the cell. It's a fascinating field that combines aspects of chemistry, biology, and physics to understand molecular functions in a detailed manner. Diving into structural biochemistry lays the groundwork for comprehending the complex architecture of life on a molecular level.
Importance of Structural Biochemistry
The understanding of structural biochemistry is vital as it provides insights into how biomolecules like DNA, RNA, and proteins interact and function. These insights are crucial for a variety of reasons:
- Drug Design: Knowledge of protein structures can guide the design of drugs that fit like keys into molecular locks.
- Genetic Engineering: Understanding DNA structures allows for precise genetic modifications.
- Diagnosis of Diseases: Structural changes in proteins can indicate certain diseases.
- Evolution and Phylogenetics: Structures help trace evolutionary relationships among species.
One striking example of structural biochemistry at work is the discovery of the double-helix structure of DNA by James Watson and Francis Crick. This discovery was pivotal in advancing genetic research and understanding inheritance patterns.
Proteins are often referred to as the workhorses of the cell due to their vast array of functions, each dependent on their unique structures.
Tools and Techniques in Structural Biochemistry
Structural biochemists use a range of sophisticated tools and techniques to examine and manipulate biological molecules. These tools include:
- X-ray crystallography: Used to determine the three-dimensional structure of large biomolecules.
- Nuclear Magnetic Resonance (NMR) Spectroscopy: Allows researchers to study protein structures in solution.
- Cryo-electron Microscopy: Provides high-resolution images of biomolecular structures.
X-ray crystallography has been instrumental in solving the structures of many important biomolecules. It involves diffracting X-rays through a crystallized sample of the protein or nucleic acid and analyzing the pattern to deduce its structure. However, not all molecules can be easily crystallized, posing a challenge. Cryo-electron microscopy overcomes some limitations of crystallography by allowing scientists to observe molecules in a near-native state without the need for crystallization. This advancement has been recognized with a Nobel Prize in Chemistry.
Techniques in Structural Biochemistry
In structural biochemistry, various advanced techniques are used to unravel the mysteries of biomolecular structures. These techniques enable scientists to observe, model, and manipulate biological macromolecules, facilitating the understanding of their diverse functions and interactions.
X-ray Crystallography
X-ray crystallography is a pioneering method for determining the atomic and molecular structure of crystals, including proteins and nucleic acids. By measuring the angles and intensities of X-rays diffracted through a crystal, researchers can produce a three-dimensional image of electron densities, which helps in mapping out the precise structure of a molecule.Here is a basic equation involved in X-ray crystallography:
- Bragg's Law: \[n\lambda = 2d\sin\theta\] where \(n\) is an integer, \(\lambda\) is the wavelength of the incident X-ray, \(d\) is the spacing between the crystal planes, and \(\theta\) is the angle of incidence.
A significant example of X-ray crystallography's impact is the determination of the structure of hemoglobin, which elucidated how it carries oxygen in the bloodstream.
Nuclear Magnetic Resonance (NMR) Spectroscopy
NMR spectroscopy is utilized to study the structure and dynamics of proteins and nucleic acids in solution. NMR provides information about the physical and chemical properties of atoms or the molecules in which they are contained, making it ideal for viewing biomolecules in their natural state.
One of the limitations of X-ray crystallography is that it requires the biomolecule to be in a crystallized form, which is not always feasible. On the other hand, NMR spectroscopy does not require crystallization. It uses the magnetic properties of atomic nuclei and their interaction with magnetic fields to get high-resolution data, allowing researchers to analyze molecules in more physiologically relevant environments. The challenge with NMR lies in its difficulty to analyze very large biomolecules and the need for high concentrations of samples.
Cryo-electron Microscopy (Cryo-EM)
Cryo-electron microscopy (Cryo-EM) is a state-of-the-art technique that has revolutionized the field of structural biochemistry. By flash-freezing aqueous samples of biomolecules, scientists can use electron beams to capture detailed images of biomolecular complexes without requiring crystallization.
Cryo-EM is especially powerful for studying large complexes such as ribosomes.
Using Cryo-EM, researchers were able to visualize the Zika virus at an unprecedented resolution, which was crucial for the development of vaccines and therapeutic strategies.
Comparative Chart of Techniques
For clarity and quick comparison, here's a chart comparing the various techniques:
Technique | Requires Crystallization? | Sample State | Best for |
X-ray Crystallography | Yes | Crystalline | Small to Medium-sized proteins |
NMR Spectroscopy | No | Solution | Small proteins and nucleic acids |
Cryo-EM | No | Frozen Hydrated | Large complexes |
Structural Biochemistry in Medical Research
Structural biochemistry plays a significant role in medical research by offering profound insights into the molecular structures of biological compounds. Understanding how these molecules function and interact provides a foundation for many medical breakthroughs.
Role in Drug Discovery
In drug discovery, structural biochemistry is essential for understanding the specific interactions between drug molecules and their biological targets. This understanding leads to the development of medications that can effectively combat diseases. By analyzing molecular structures, researchers can design drugs that fit precisely into the active sites of their target proteins.At the molecular level, the interaction between a drug and its target is often described through equations such as the binding affinity equation:
- \[ K_d = \frac{{[D][T]}}{{[DT]}} \]
- \(K_d\) is the dissociation constant,
- \( [D] \) represents the concentration of the drug,
- \( [T] \) is the concentration of the target,
- \( [DT] \) is the concentration of the drug-target complex.
A lower \(K_d\) value indicates a higher affinity of the drug for its target, which is often desirable in therapeutic settings.
Exploring Genetic Disorders
By studying the structures of DNA and associated proteins, structural biochemists can identify mutations that lead to genetic disorders. These abnormalities in genetic material can cause conditions such as sickle cell anemia or cystic fibrosis. By mapping out these molecular structures, researchers can pinpoint exact locations of defects and understand their biochemical consequences, paving the way for potential therapies.
Consider the case of cystic fibrosis, a genetic disorder caused by mutations in the CFTR gene. Structural biochemistry has enabled researchers to determine the precise configuration of the CFTR protein, shedding light on how specific mutations disrupt its function. This understanding has led to the development of targeted drugs that can correct these defects, providing significant relief to patients.
Enzyme Function and Inhibition
Enzymes are proteins that catalyze biochemical reactions, and understanding their structure is key to manipulating their activity. Structural biochemistry helps explain how enzymes recognize their substrates and what structural features are critical for their function. This knowledge can be used to design inhibitors that prevent enzyme action, which is crucial in treating diseases where enzyme activity is detrimental, such as in cancer or bacterial infections.
A classic example of enzyme inhibition is the drug penicillin, which inhibits the enzyme transpeptidase in bacteria, preventing them from forming cell walls and thereby killing them.
Structural Biology in Biochemistry
Structural biology in biochemistry delves into the architecture of biomolecules, revealing how their form enables their function. This field is crucial for understanding the complex interactions that sustain life.
Biochemistry Protein Structure and Function
Proteins are fundamental macromolecules with diverse roles in biological processes. They function as enzymes, structural components, signaling molecules, and transporters. Understanding protein structure is vital since it dictates how they interact with other molecules.The primary structure of proteins consists of a sequence of amino acids, held together by peptide bonds. This linear chain folds into secondary structures like alpha-helices and beta-sheets, stabilized by hydrogen bonds. Tertiary structure refers to the overall 3D shape of a single polypeptide, while quaternary structure involves multiple polypeptides assembling into a functional protein complex.
Protein Structure Hierarchy:
- Primary: Amino acid sequence
- Secondary: Alpha-helices and beta-sheets
- Tertiary: 3D conformation of a single polypeptide
- Quaternary: Assembly of multiple polypeptides
For instance, hemoglobin has a quaternary structure comprising four polypeptide chains. Its ability to transport oxygen is directly linked to its structural configuration.
Minor alterations in amino acid sequences can significantly impact protein function, which is why genetic mutations sometimes result in diseases.
Biochemistry Protein Structure Techniques
Various techniques are used to study protein structures, each offering unique insights:
- X-ray Crystallography: Provides high-resolution details of crystalline proteins.
- NMR Spectroscopy: Useful for examining proteins in solution.
- Cryo-electron Microscopy: Captures images of large protein complexes in their native state.
- Spectrophotometry: Measures absorption of light to study protein-ligand interactions.
X-ray crystallography has been a cornerstone in structural biology, leading to breakthroughs in understanding biomolecular structures. It works by analyzing the pattern of X-ray beams diffracted through crystals of biomolecules. The famous equation used here is Bragg's Law: \[ n\lambda = 2d\sin\theta \] where \(n\) is an integer, \(\lambda\) is the wavelength of X-rays, \(d\) is the distance between crystal planes, and \(\theta\) is the angle of incidence. These details collectively enable the creation of a 3D model that showcases the atomic positions within the crystal.
Cryo-EM has gained prominence for visualizing large biomolecular complexes that cannot be crystallized easily, like viruses and ribosomes.
structural biochemistry - Key takeaways
- Structural Biochemistry Definition: A branch of biology focusing on molecular structures of biological macromolecules, such as proteins and nucleic acids, assessing how structural changes affect their functions.
- Structural Biochemistry in Medical Research: Provides crucial insights into interactions and functions of biomolecules, aiding drug design, genetic engineering, disease diagnosis, and understanding evolutionary relationships.
- Biochemistry Protein Structure and Function: Proteins' diverse biological roles depend on their structure, which includes primary (amino acid sequence), secondary (alpha-helices and beta-sheets), tertiary (3D conformation), and quaternary (assembly of polypeptides) structures.
- Biochemistry Protein Structure Techniques: Techniques include X-ray crystallography, NMR spectroscopy, Cryo-electron Microscopy, and spectrophotometry, each providing unique insights into protein structures.
- Techniques in Structural Biochemistry: Tools such as X-ray crystallography, NMR spectroscopy, and Cryo-electron Microscopy are used to determine molecular structures of biomolecules, crucial for visualizing molecular interactions.
- Structural Biology in Biochemistry: It examines the architecture of biomolecules, essential for understanding biological processes through molecular interactions.
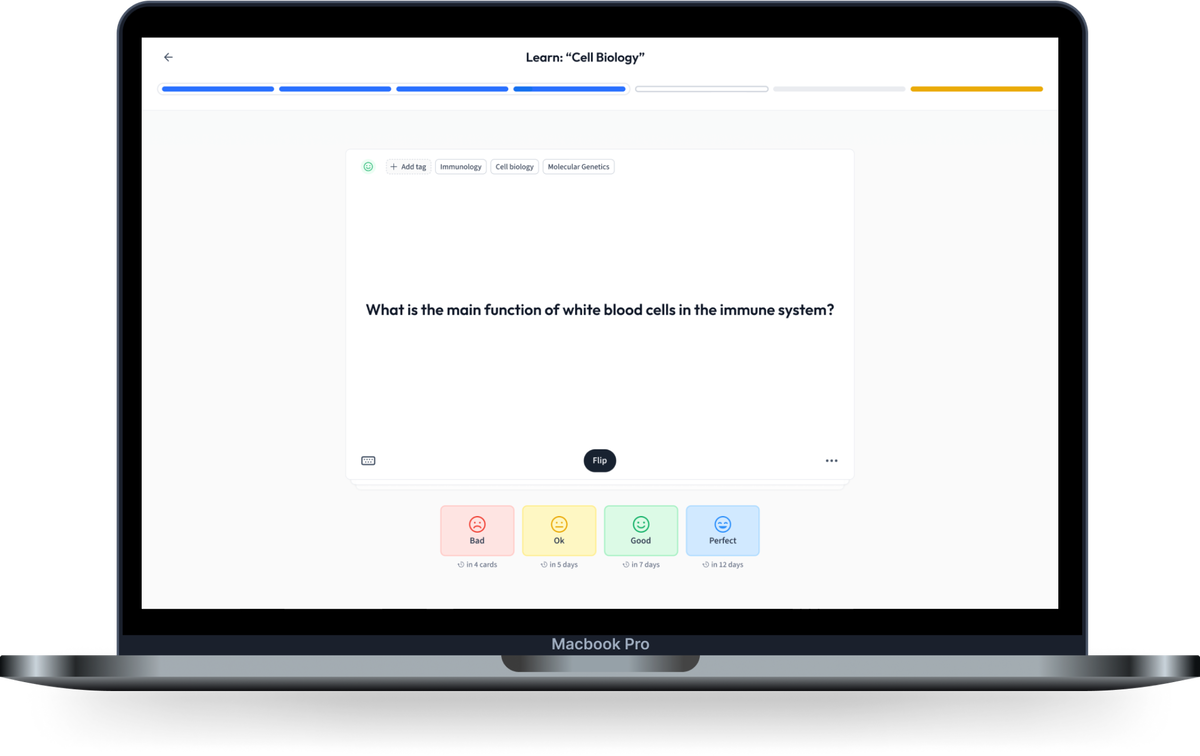
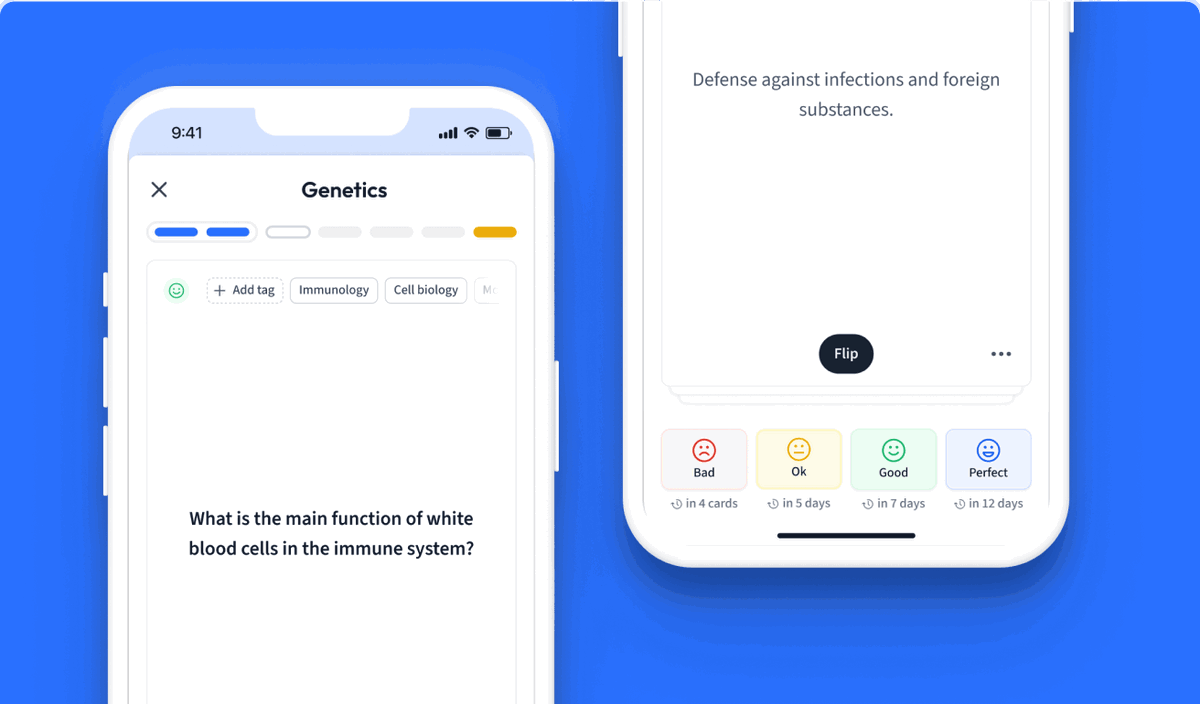
Learn with 12 structural biochemistry flashcards in the free StudySmarter app
Already have an account? Log in
Frequently Asked Questions about structural biochemistry
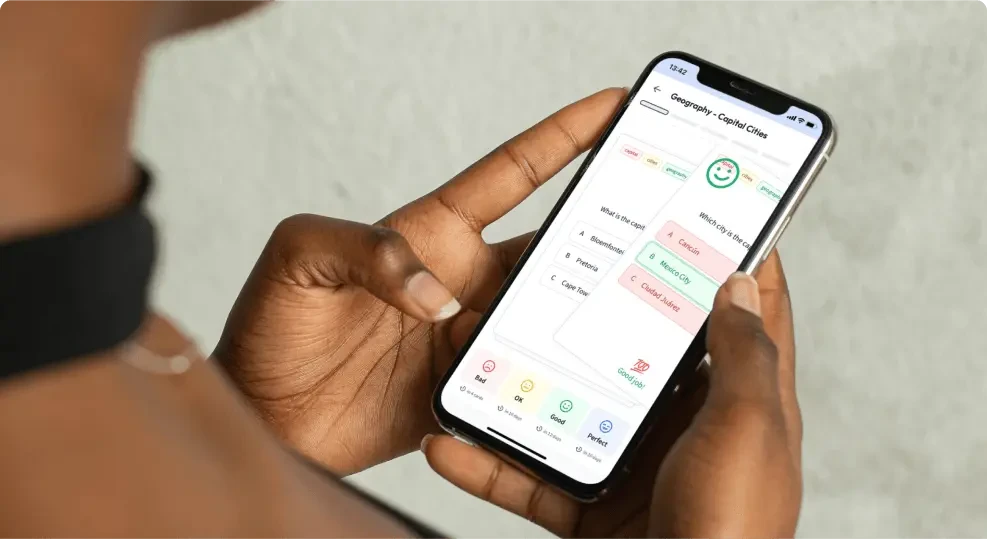
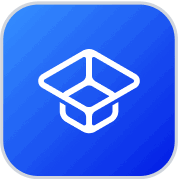
About StudySmarter
StudySmarter is a globally recognized educational technology company, offering a holistic learning platform designed for students of all ages and educational levels. Our platform provides learning support for a wide range of subjects, including STEM, Social Sciences, and Languages and also helps students to successfully master various tests and exams worldwide, such as GCSE, A Level, SAT, ACT, Abitur, and more. We offer an extensive library of learning materials, including interactive flashcards, comprehensive textbook solutions, and detailed explanations. The cutting-edge technology and tools we provide help students create their own learning materials. StudySmarter’s content is not only expert-verified but also regularly updated to ensure accuracy and relevance.
Learn more