Jump to a key chapter
Subcellular Proteomics: A Brief Educational Overview
Subcellular proteomics is an insightful field of study within the broader realm of proteomics. It focuses on the analysis of protein distribution and function within various compartments of a cell. This knowledge is crucial as it helps you understand cellular processes at a finer level. In this section, the focus will shift to the specific techniques used in subcellular proteomics and the concept of spatial proteomics with subcellular resolution.
Techniques in Subcellular Proteomics
Subcellular proteomics encompasses various techniques that allow detailed protein mapping within cells. Different methods offer unique advantages, enabling you to choose the best approach depending on the specific cellular context and research goal.Some popular techniques include:
- Fractionation Techniques: These involve breaking down the cell into subcellular compartments, which are then analyzed to identify proteins specific to each compartment.
- Mass Spectrometry: A key tool for protein analysis, allowing for precise identification and quantification of proteins present in minute quantities.
- Immunofluorescence: This technique uses antibody-based labeling to visualize proteins within cells, providing a spatial context to protein localization.
Did you know? Combining multiple techniques can significantly enhance the accuracy of protein identification within specific cellular compartments.
Imagine a study investigating mitochondrial proteins specifically to understand energy metabolism flaws in a disease state. Using fractionation followed by mass spectrometry, you can precisely identify the list of proteins present only in mitochondrial fractions, providing insights into potential dysfunctions.
Spatial Proteomics with Subcellular Resolution
Spatial proteomics aims to map proteins within the complex architecture of a cell with high precision. It’s particularly important as precise protein localization can determine cellular function and behavior.Key methods to achieve spatial resolution include:
- Fluorescence-Activated Cell Sorting (FACS): Enables the sorting and analysis of proteins in distinct cell populations or cellular organelles.
- Proximity Labeling: Captures proteins in proximity to a known protein of interest, providing insights into local protein environments.
Exploring spatial proteomics further, the use of cutting-edge techniques such as single-cell RNA sequencing combined with proteomics could transform cellular biology. By pairing protein data with gene expression profiles, it's possible to gain a holistic view of cellular states and molecular interactions that govern cell behavior and fate. This integrative approach can rapidly advance areas such as cancer research and personalized medicine, where understanding individual cell differences is crucial for effective treatment development.
Human Proteome Subcellular Localization
Subcellular localization of the human proteome is a comprehensive exploration of where proteins reside within a cell and their respective roles. Understanding the subcellular map of the human proteome is vital for elucidating cellular function and pathology. Let's delve into how a subcellular map is created and its significance in biological research.
Creating a Subcellular Map of the Human Proteome
To create a high-resolution map of the human proteome, researchers employ a series of sophisticated techniques. These methods allow for precise determination of protein locations within various cellular compartments.Key steps involved in mapping include:
- Cell Fractionation: This process separates cellular components, enabling the study of proteins within individual organelles.
- Mass Spectrometry: Proteins from each fraction are analyzed to identify and quantify them, contributing to the comprehensive map.
- Computational Analysis: Bioinformatics tools help interpret data, predict protein localization, and map them to specific cellular locales.
Subcellular Localization: The process of identifying the specific location of proteins within a cellular structure, such as organelles, which is crucial for understanding their biological function.
Consider the protein \textit{cytochrome c}, which plays a role in the electron transport chain. By using subcellular proteomics, researchers can localize it to the mitochondria, confirming its role in energy production and apoptosis processes.
In the context of system biology, mapping the human proteome with cellular specificity uses techniques like \textbf{single-cell proteomics}. This approach enables the study of proteome variability at a single-cell level, enhancing understanding of how protein composition can affect cell-to-cell variability in tissue behavior. Given that human tissues are comprised of diverse cell types, these insights could revolutionize personalized medicine, offering target-based therapies for heterogeneous diseases like cancer.
Fun Fact: The map of the human proteome is often compared to a city's map, where different neighborhoods (organelles) have unique roles and protein 'residents' that contribute to the city's (cell's) overall function.
Chemical Proteomics for Subcellular Proteome Analysis
Chemical proteomics is a powerful approach employed to study the proteome at a subcellular level. It integrates chemistry, biology, and analytical techniques to understand protein function and interactions within cellular contexts. In this section, the focus is on highlighting methods and tools that are pivotal for conducting chemical proteomics.
Methods and Tools in Chemical Proteomics
In the realm of chemical proteomics, various methods and tools are available that enhance the exploration of protein biology in living cells. These techniques are instrumental in providing insights into protein activities, interactions, and modifications.Some of the key approaches include:
- Activity-Based Protein Profiling (ABPP): From this method, researchers can examine enzyme activities within live cells by using small chemical probes tailored to bind active sites.
- Chemical Labeling: This involves the addition of chemical tags to proteins, which can help track and identify them during mass spectrometry analysis.
- Affinity-Based Methods: Using these methods, specific protein interactions are captured and studied through affinity tags or molecules such as antibodies.
Mass Spectrometry: A technique used in chemical proteomics to identify proteins by measuring the mass-to-charge ratio of ions. It enables detailed protein analysis in complex samples.
Consider a scenario where researchers are investigating the post-translational modifications (PTMs) of proteins during cell signaling. By using chemical labels that react specifically with phosphorylated residues, and analyzing them through mass spectrometry, you can determine which proteins undergo phosphorylation and potentially identify new signaling pathways.
Reflecting on the capabilities of chemical proteomics, quantitative chemical proteomics represents a significant advancement. This technique combines the principles of chemical proteomics with quantitative methods to compare protein abundances or modifications under different conditions. Such capabilities are essential for drug discovery, as they help elucidate the mechanisms of drug action or resistance by measuring changes in protein expressions or modifications.
Remember: The success of chemical proteomics often hinges on the ingenuity of the chemical probes and their specificity to target desired cellular proteins successfully.
Importantly, chemical proteomics requires meticulous experimental designs. It often integrates computational tools for data analysis, which facilitates the identification of proteins and their modifications across subcellular compartments. The complex datasets generated demand robust bioinformatics pipelines to ensure accurate protein characterization and localization across the proteome.
Applications of Subcellular Proteomics in Biomedicine
Subcellular proteomics plays a transformative role in biomedicine, with applications that help unravel complex biological processes involved in health and disease. By harnessing the distinct protein signatures of cellular compartments, researchers can gain valuable insights into disease mechanisms, potential biomarkers, and therapeutic targets.
Potential Impacts on Human Health and Disease Studies
The study of proteins at a subcellular level provides crucial information for understanding the pathophysiology of diseases and improving human health. Here’s how subcellular proteomics impacts disease studies:
- Biomarker Discovery: Identifying specific proteins localized in particular cell organelles serves as potential biomarkers for disease diagnosis and progression.
- Pathway Analysis: Understanding how proteins within subcellular compartments interact and contribute to cellular pathways aids in pinpointing disruptions causing diseases.
- Drug Target Identification: By studying the protein dynamics and functions within cells, new drug targets can be discovered, enabling the development of more effective therapies.
- Therapeutic Development: Subcellular proteomics enables the design of therapies that specifically modulate protein activity within defined cellular locales, minimizing off-target effects.
Biomarker: A biological molecule found in blood, other body fluids, or tissues that is a sign of a normal or abnormal process, or of a condition or disease. Biomarkers are used to see how well the body responds to a treatment for a disease or condition.
An example of biomarker discovery through subcellular proteomics is the identification of the protein prostate-specific antigen (PSA), which is found in the prostate gland's secretory vesicles and is commonly used to screen for prostate cancer.
Exploring further, omics integration in biomedicine combines subcellular proteomics with genomics and transcriptomics. This integrative approach allows for a comprehensive understanding of biological networks and how changes in DNA, RNA, and proteins contribute to the onset and progression of diseases. For instance, in cancer research, this means mapping out the entire cascade from gene mutations to protein modifications and their effects on cellular pathways, providing a detailed view that is critical for developing personalized medicine strategies.
Did you know? Utilizing subcellular proteomics can help in identifying early-stage disease biomarkers, offering a potential to intervene before a disease progresses significantly.
subcellular proteomics - Key takeaways
- Subcellular Proteomics Definition: A field focusing on protein distribution and function within cellular compartments for understanding cellular processes.
- Techniques in Subcellular Proteomics: Includes fractionation techniques, mass spectrometry, and immunofluorescence for detailed protein mapping.
- Spatial Proteomics with Subcellular Resolution: Methods like fluorescence-activated cell sorting and proximity labeling map proteins within a cell precisely.
- Human Proteome Subcellular Localization: Involves creating a high-resolution map of human proteome's protein localizations using techniques such as cell fractionation and computational analysis.
- Chemical Proteomics for Subcellular Proteome Analysis: Integrates chemistry and biology to study protein interactions, using methods like activity-based protein profiling and chemical labeling.
- Applications in Biomedicine: Subcellular proteomics aids in biomarker discovery, pathway analysis, drug target identification, and therapeutic development for disease research.
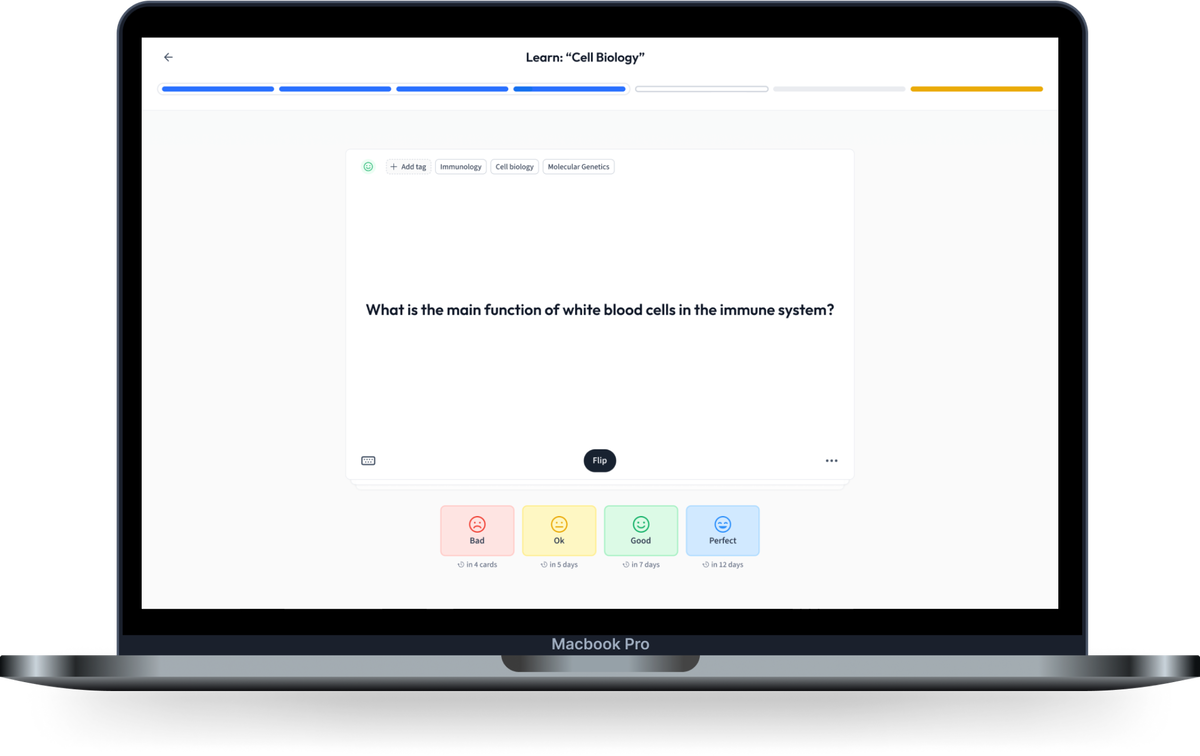
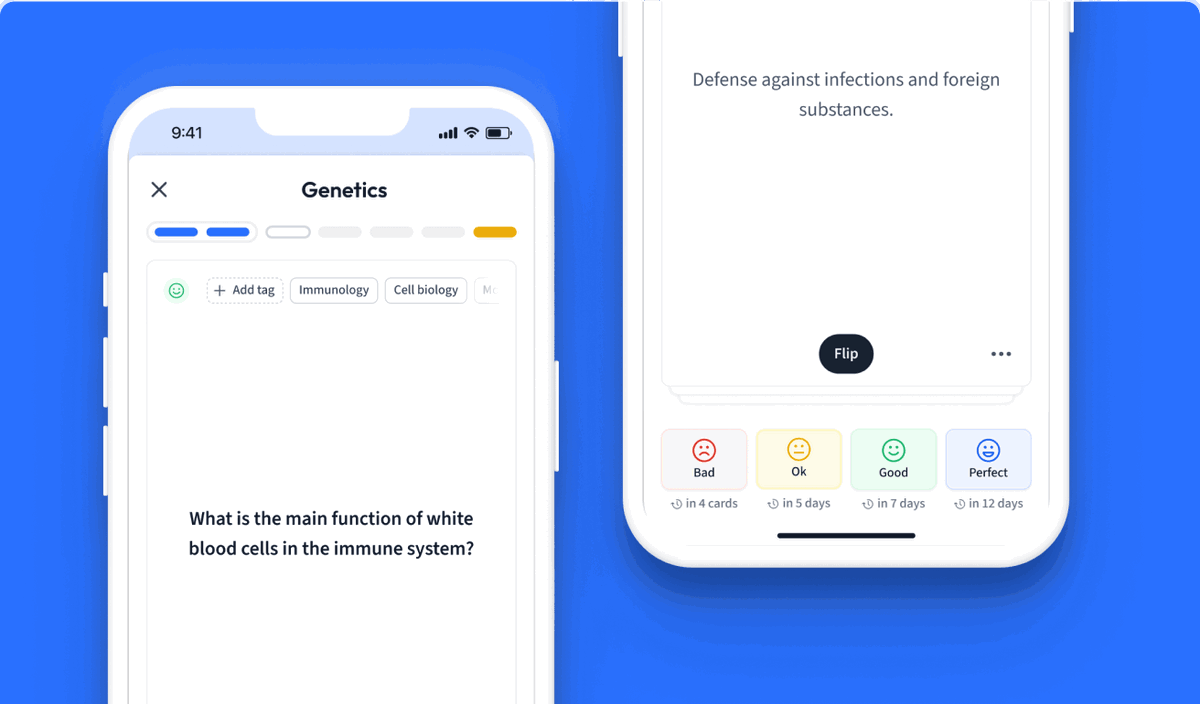
Learn with 12 subcellular proteomics flashcards in the free StudySmarter app
Already have an account? Log in
Frequently Asked Questions about subcellular proteomics
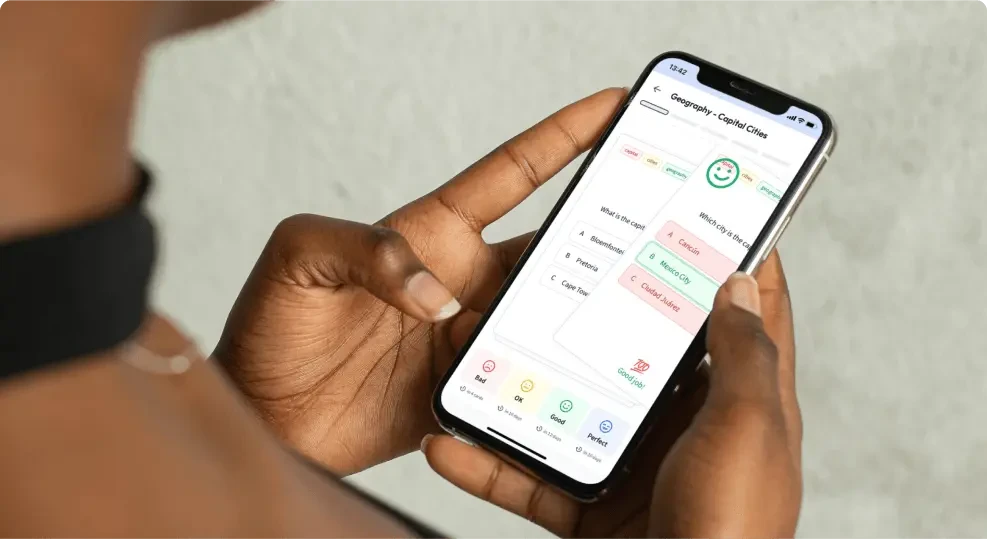
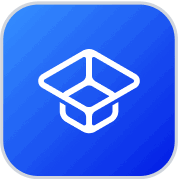
About StudySmarter
StudySmarter is a globally recognized educational technology company, offering a holistic learning platform designed for students of all ages and educational levels. Our platform provides learning support for a wide range of subjects, including STEM, Social Sciences, and Languages and also helps students to successfully master various tests and exams worldwide, such as GCSE, A Level, SAT, ACT, Abitur, and more. We offer an extensive library of learning materials, including interactive flashcards, comprehensive textbook solutions, and detailed explanations. The cutting-edge technology and tools we provide help students create their own learning materials. StudySmarter’s content is not only expert-verified but also regularly updated to ensure accuracy and relevance.
Learn more