Jump to a key chapter
What is X-ray Crystallography
X-ray Crystallography is a powerful technique used to determine the atomic and molecular structure of a crystal. This process involves directing X-rays at a crystal and measuring the angles and intensities of the scattered rays. By analyzing these patterns, researchers can create a three-dimensional picture of electron density within the crystal, which reveals the structure of the molecule.
Principles of X-ray Crystallography
The fundamental principle of X-ray Crystallography is based on the diffraction of X-rays. When X-rays hit a crystal, they are scattered in many different directions. The scattered X-rays can interfere constructively or destructively, creating a pattern of spots known as a diffraction pattern.The Bragg's Law is central to understanding these patterns. Bragg's Law is mathematically expressed as:
n\lambda = 2d\sin\theta
Here, n is an integer, \(\lambda\) is the wavelength of the X-rays, d is the distance between crystal planes, and \(\theta\) is the angle of incidence.By altering the angle \(\theta\), different planes within the crystal are observed, allowing a comprehensive analysis of its structure.
Example: Consider a crystal with plane spacing d of 0.2 nm and an X-ray wavelength \(\lambda\) of 0.1 nm. According to Bragg's Law, when \(\theta=30^\circ\), \(n\) is calculated as follows: \[ n=\frac{2\times0.2\times\sin30^\circ}{0.1} = 2 \]
Remember, X-ray Crystallography is a non-destructive technique, meaning the sample remains intact after examination.
Applications of X-ray Crystallography
X-ray Crystallography is used extensively across various scientific fields, primarily in understanding the structures of:
- Proteins and Enzymes
- Nucleic acids (like DNA)
- Minerals and Inorganic compounds
Modern X-ray Crystallography utilizes synchrotron radiation for data collection. Synchrotron sources provide high-intensity X-rays, significantly improving the quality of diffraction data. These allow for more precise determination of complex structures and faster data acquisition. Furthermore, advanced software algorithms can compute electron density maps rapidly, transforming the field and enabling a deeper insight into biological processes.
How Does X-ray Crystallography Work
X-ray Crystallography is a technique that uses X-rays to study the atomic structure of crystals. By directing X-rays at a crystal, the rays are diffracted, creating a pattern that can be analyzed to reveal the crystal's structure.
X-ray Crystallography Diffraction
X-ray diffraction is the key process in X-ray Crystallography. When X-rays strike a crystalline material, they are scattered in various directions. The resulting pattern, known as a diffraction pattern, is a direct consequence of the interference of the scattered rays.To understand this, you must consider Bragg's Law, which explains the condition for constructive interference.
n\lambda = 2d\sin\theta
In this equation, n is an integer, \(\lambda\) is the wavelength of the incident X-rays, d is the distance between atomic planes in the crystal, and \(\theta\) is the angle of incidence. Constructive interference occurs when the path difference between X-rays reflecting from different planes is an integer multiple of the wavelength.The diffraction pattern is captured using detectors and used to calculate electron density maps, which help to visualize the atomic arrangement within the crystal.
The accuracy of the data obtained from X-ray diffraction heavily depends on the quality and size of the crystal being studied.
X-ray Crystallography Explained
X-ray Crystallography involves a series of scientific and mathematical processes to determine the structure of a crystal. This begins with the alignment of a single crystal and exposure to an X-ray beam. Upon striking the crystal, the X-rays are scattered, and the diffraction pattern is recorded.The pattern consists of various scattered beams which reflect the symmetry and atomic arrangement of the crystal lattice. These scattered beams are analyzed to yield an electron density map. Advanced computer software assists in transforming this map into a 3D model of the molecule, elucidating the arrangement of atoms.
In modern X-ray Crystallography, advanced technologies such as synchrotron radiation sources have revolutionized data collection. These sources provide extremely intense X-rays that result in high-quality diffraction data. The use of synchrotrons enables scientists to explore smaller and more complex structures. Moreover, the introduction of cryogenic techniques allows researchers to maintain samples at low temperatures, reducing radiation damage. This technique enables accurate data collection and enhances the resolution of the final structure. A typical workflow in X-ray Crystallography includes several key steps:
- Crystallization of the sample
- Mounting on a goniometer
- Exposure to X-ray beam
- Detection of diffraction pattern
- Analytical processing to obtain electron density map
X-ray Crystallography Theory
Understanding X-ray Crystallography Theory involves studying how X-rays interact with the crystal lattice, producing a diffraction pattern that can be interpreted to reveal the structure of the crystal's molecules.
Fundamental Concepts in X-ray Crystallography
Central to X-ray Crystallography is the concept of diffraction. When X-rays hit a crystal, they are scattered, leading to interference patterns that are foundational to this technique.The lattice is another important concept; it is the periodic arrangement of molecules that provides the conditions necessary for diffraction. Furthermore, one should have a comprehensive understanding of symmetry, which refers to the repetitive and symmetrical nature of crystal structures leading to characteristic diffraction patterns.
Bragg's Law is crucial for understanding X-ray diffraction. It is given by: \[n\lambda = 2d\sin\theta\] where \(n\) is an integer, \(\lambda\) is the X-ray wavelength, \(d\) is the distance between crystal planes, and \(\theta\) is the angle of incidence.
The exact value of \(d\) in Bragg's Law helps in identifying specific planes within the crystal lattice.
For a wavelength \(\lambda = 1.54\, \text{Å}\) and an incident angle of \(30^\circ\), if the calculated \(d\)-spacing is \(2\, \text{Å}\), Bragg's Law gives us: \[n = \frac{2 \times 2 \times 0.5}{1.54}\approx1.3\] Since \(n\) must be an integer, diffraction occurs prominently when \(n=1\).
One should also consider the role of phase problems in crystallography, which involve complexities in determining the exact position of atoms due to missing phase information. Advanced mathematical techniques and algorithms are used to overcome these issues, including methods like direct methods and the use of Fourier transforms to reconstruct the phase.
Importance of X-ray Crystallography in Science
The scientific community widely uses X-ray Crystallography to understand the structure-function relationships in biomolecules. This method has revolutionized fields like structural biology and chemistry by providing detailed atomic structures.
Key areas where X-ray Crystallography has made significant impacts include:
- Biochemistry: Determining the 3D structures of proteins and enzymes, revealing active sites, and facilitating drug design.
- Material science: Analyzing the composition and properties of minerals and complex composites.
- Pharmacology: Assisting in creating new pharmaceuticals by understanding molecular interactions.
Nobel Prize Contributions: The impact of X-ray Crystallography has been recognized by numerous Nobel Prizes. One notable example is the elucidation of the DNA double helix structure by Watson and Crick, which was greatly aided by Crick's exposure to crystallographic data.
Applications of X-ray Crystallography in Medicine
X-ray Crystallography is an essential tool in modern medicine. By allowing scientists to visualize complex molecular structures, it enables significant advancements in understanding diseases and developing treatments.
Understanding Disease Mechanisms
The study of molecular structure through X-ray Crystallography provides insights into disease mechanisms. Visualizing molecules in high detail aids researchers in identifying how subtle changes in structure can lead to malfunction.For example, X-ray Crystallography has been instrumental in unraveling structures of pathogenic proteins, allowing scientists to pinpoint how mutations affect protein function and contribute to diseases like cystic fibrosis or diabetes.
X-ray Crystallography: A technique used to determine the atomic and molecular structure of a crystal by measuring the angles and intensities of diffracted X-ray beams.
An example includes the use of X-ray Crystallography to determine the structure of the protein hemoglobin to better understand sickle cell anemia. The mutation affecting the hemoglobin structure leads to the disease, and by using X-ray Crystallography, researchers were able to visualize the malformed structure.
Understanding structural alterations in proteins due to mutations is crucial in developing therapeutic targets.
Recent studies have shown that X-ray Crystallography can be combined with other techniques, such as cryo-electron microscopy, to offer even more detailed insights into macromolecular complexes involved in disease processes. This integrative approach provides a fuller picture, combining the atomic-level precision of X-ray analysis with the broad structural view provided by electron microscopy.
Drug Development and Design
In the realm of drug development, X-ray Crystallography is invaluable for rational drug design. Knowing the exact three-dimensional structure of biological targets, such as enzymes or receptors, allows researchers to design drugs that fit perfectly into active sites.This process often involves:
- Identifying target structures that play a role in disease
- Designing molecules that can bind effectively
- Using crystallographic data to refine potential drug candidates
For instance, the development of HIV protease inhibitors was largely based on X-ray Crystallography data. The technique enabled scientists to visualize the protease bound with inhibitors, guiding the design of effective antiviral drugs.
A fascinating development in X-ray Crystallography is fragment-based drug discovery (FBDD). This method screens small chemical fragments for binding to target proteins. Once identified, these fragments can be optimized into potent compounds by examining their binding mode at the atomic level. The advantage of FBDD is its efficiency in discovering novel binding sites and creating drugs with higher specificity and lower adverse effects.
x-ray crystallography - Key takeaways
- X-ray Crystallography: A technique used to determine the atomic and molecular structure of a crystal by directing X-rays at it and analyzing the diffraction pattern.
- Bragg's Law: Fundamental theory in X-ray Crystallography, expressed as n\lambda = 2d\sin\theta, crucial for understanding X-ray diffraction patterns.
- Diffraction Patterns: Created when X-rays scatter upon hitting a crystal; these patterns help visualize the crystal's atomic arrangement.
- Applications in Science: X-ray Crystallography is widely used in structural biology, chemistry, material science, and pharmacology for drug design and molecular analysis.
- Medical Applications: Used to visualize complex molecular structures, aiding in understanding disease mechanisms and drug development.
- Modern Advancements: Utilizes synchrotron radiation and cryogenic techniques to enhance data quality and resolution in structure analysis.
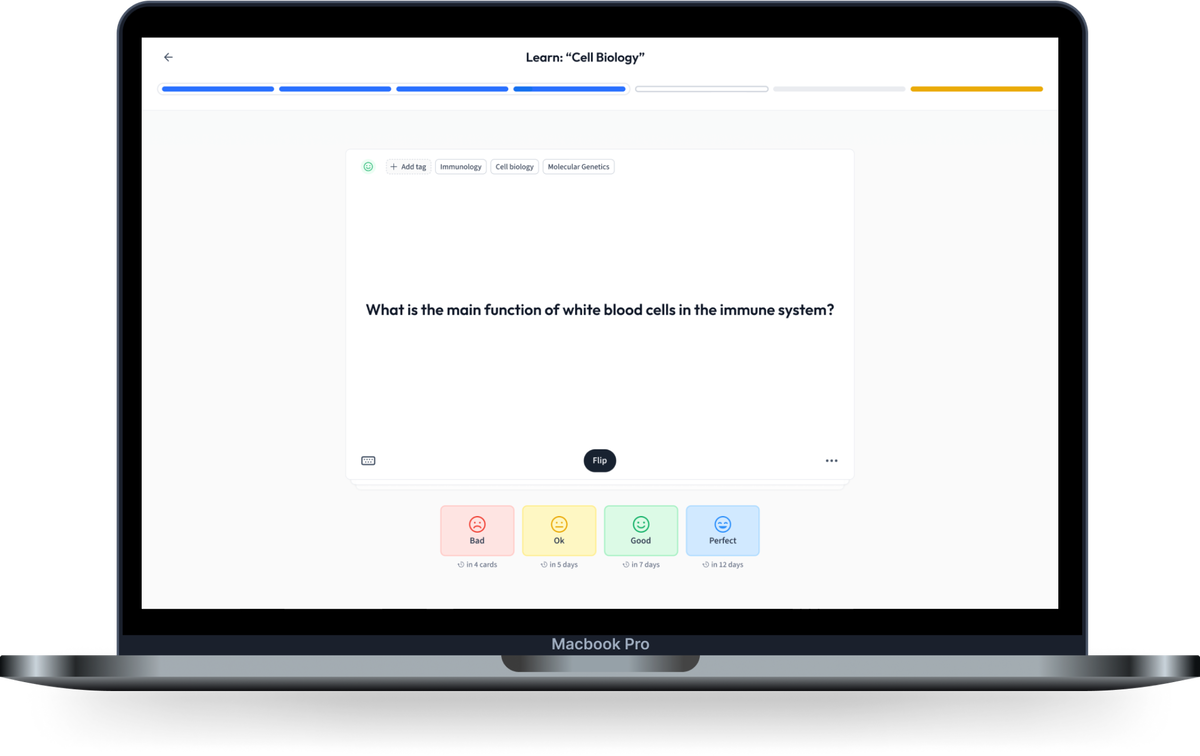
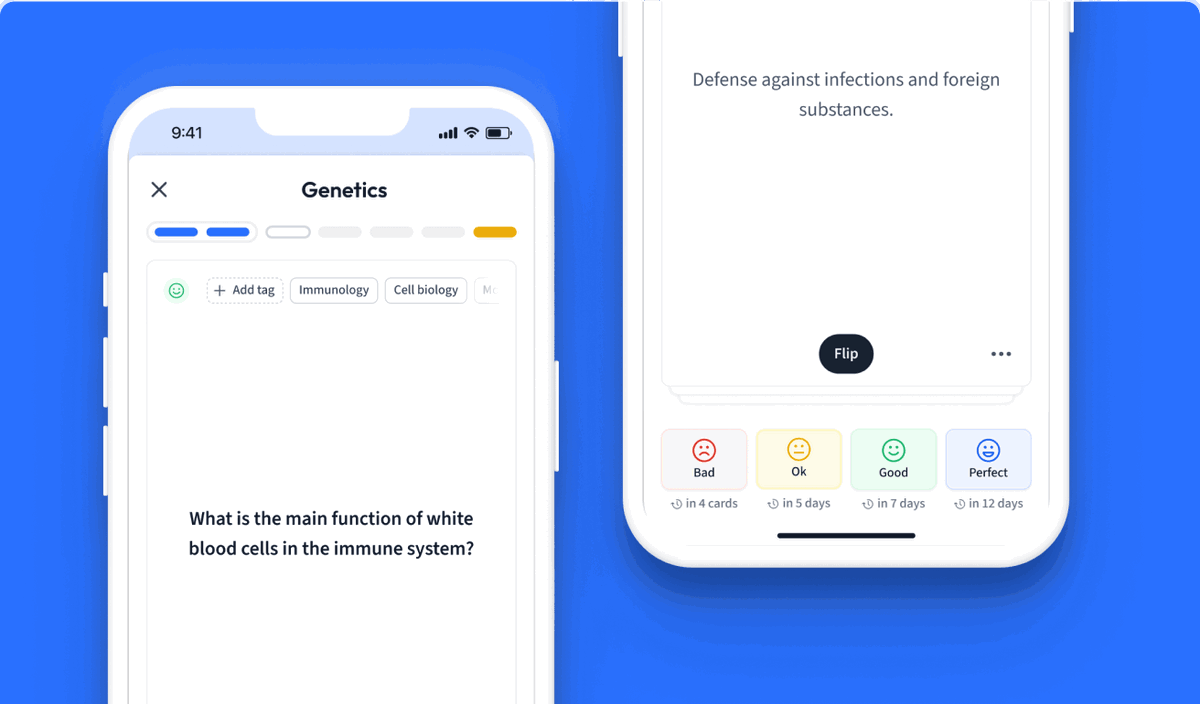
Learn with 12 x-ray crystallography flashcards in the free StudySmarter app
Already have an account? Log in
Frequently Asked Questions about x-ray crystallography
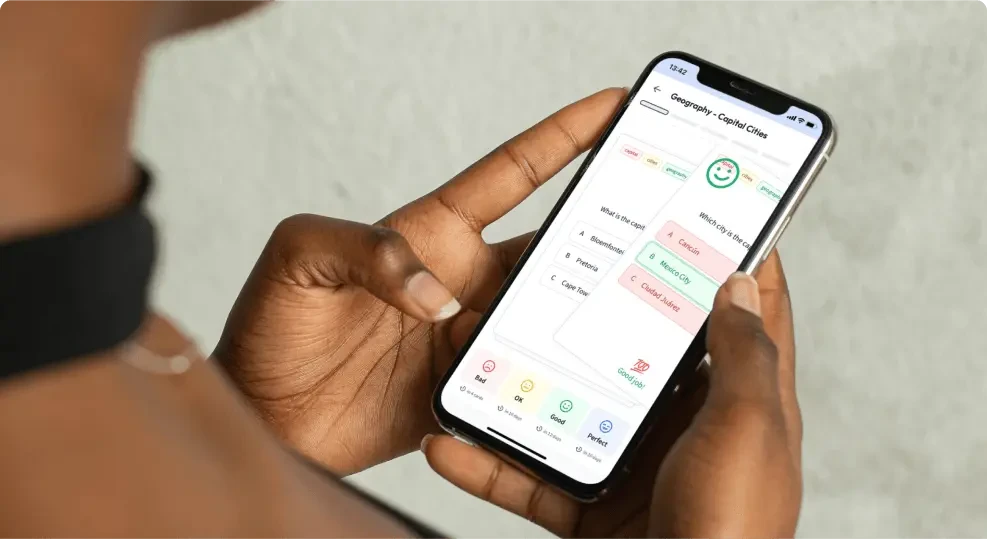
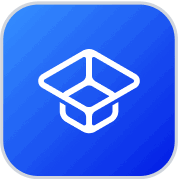
About StudySmarter
StudySmarter is a globally recognized educational technology company, offering a holistic learning platform designed for students of all ages and educational levels. Our platform provides learning support for a wide range of subjects, including STEM, Social Sciences, and Languages and also helps students to successfully master various tests and exams worldwide, such as GCSE, A Level, SAT, ACT, Abitur, and more. We offer an extensive library of learning materials, including interactive flashcards, comprehensive textbook solutions, and detailed explanations. The cutting-edge technology and tools we provide help students create their own learning materials. StudySmarter’s content is not only expert-verified but also regularly updated to ensure accuracy and relevance.
Learn more