Jump to a key chapter
Hemodynamics Definition
Hemodynamics is the study of blood flow within the circulatory system. It examines how blood moves through the heart and vessels, taking into account forces and properties such as pressure, flow, and resistance. Understanding hemodynamics is crucial in understanding cardiovascular function.
The term hemodynamics refers to the principles that govern the movement and regulation of blood flow through the cardiovascular system, influenced by factors like pressure, resistance, and flow rate.
To understand hemodynamics fully, you need to acquaint yourself with several key concepts:
- Blood Pressure: The force exerted by circulating blood on the walls of blood vessels. It is commonly measured in millimeters of mercury (mmHg).
- Cardiac Output: The volume of blood the heart pumps per minute. It is calculated as heart rate multiplied by stroke volume, where 'stroke volume' is the amount of blood pumped with each heartbeat.
- Vascular Resistance: The resistance encountered by the blood as it flows through the vessels, especially the arterioles. This affects the pressure and flow within the system.
Consider the equation for cardiac output: \[CO = SV \times HR\] where \[CO\] is cardiac output, \[SV\] is stroke volume, and \[HR\] is heart rate. If the heart rate is 70 beats per minute and the stroke volume is 70 mL, the cardiac output will be 4900 mL/min, or 4.9 L/min.
Hemodynamics involves complex interactions within the cardiovascular system. For more advanced insights, consider the Poiseuille's Law, which describes the flow of fluid in a tube: \[Q = \frac{\Delta P \cdot \pi \cdot r^4}{8 \cdot \eta \cdot l}\] where \[Q\] is the volumetric flow rate, \[\Delta P\] is the pressure gradient, \[r\] is the radius of the vessel, \[\eta\] is the dynamic viscosity, and \[l\] is the length of the tube. This law demonstrates how changes in the radius of blood vessels can significantly impact blood flow.
Remember, small changes in vessel diameter have a large impact on blood flow, due to the r^4 term in Poiseuille's law.
Hemodynamics Physiology
In the study of hemodynamics physiology, you will explore the dynamic aspects of blood flow and how it is regulated within the body. By understanding how various factors come into play, you will gain insights into cardiovascular health and disease management.The principles of hemodynamics are crucial for medical students and anyone interested in the function of the circulatory system. This knowledge is applicable in many medical fields, including cardiology and emergency medicine.
Hemodynamics physiology refers to the mechanisms and properties that influence how blood flows through the circulatory system. This study involves looking at pressure gradients, flow rates, and vascular resistance.
Components of Hemodynamics Physiology
Hemodynamics physiology involves key components, each playing a vital role in the circulatory system's function:
- Heart Function: Acts as the pump that drives blood circulation by generating pressure.
- Vascular System: Comprises arteries, veins, and capillaries, each with specific roles in carrying oxygenated and deoxygenated blood.
- Blood Viscosity: Refers to the thickness and stickiness of blood, impacting its flow rate.
Let’s illustrate hemodynamics using a simple analogy:Think of the circulatory system like a garden hose system. The heart functions like the pump providing water pressure, the arteries and veins are hoses of varying sizes, and the blood is the water flowing through them. Just as a kink in a hose affects water flow, issues within the vascular system can significantly impact blood circulation.
A deeper dive into hemodynamics physiology reveals how changes in the vascular tone—caused by autonomic nervous stimulation or chemical signals like hormones—can alter blood pressure and flow. This adjustment process is crucial for maintaining homeostasis under different physiological conditions, such as exercise or stress.The body has feedback mechanisms, like the baroreceptor reflex, that instantly adjust heart rate and vessel dilation to maintain stable blood flow. Understanding these processes is essential for diagnosing and treating conditions like hypertension and heart failure.
Understanding hemodynamics can enhance your ability to interpret blood pressure readings and recognize abnormalities such as hypertension.
Blood Pressure Regulation in Hemodynamics
Blood pressure regulation is a critical aspect of hemodynamics, ensuring that blood circulates efficiently throughout the body. Proper blood flow is vital for delivering oxygen and nutrients to tissues and removing waste products.Regulation of blood pressure involves a complex interplay of various factors that work together to maintain stability across different physiological conditions, such as exercise or at rest.
Primary Mechanisms of Blood Pressure Regulation
The body uses several mechanisms to regulate blood pressure, adapting to the ever-changing needs and activities of the organism. These include:
- Nervous System: The sympathetic nervous system increases heart rate and constricts blood vessels in response to stress, raising blood pressure.
- Renal System: The kidneys regulate blood volume by controlling the amount of sodium and water excreted, which impacts blood pressure.
- Hormones: Hormones such as adrenaline and angiotensin II play a key role in modulating heart function and vascular tone.
Blood pressure is the force exerted by circulating blood on the walls of the blood vessels. It is primarily determined by cardiac output, vascular resistance, and blood volume.
Consider the equation for mean arterial pressure (MAP):\[MAP = CO \times TPR\] where \(MAP\) is mean arterial pressure, \(CO\) is cardiac output, and \(TPR\) is total peripheral resistance. If cardiac output is 5 L/min and total peripheral resistance is 20 mmHg/L/min, the mean arterial pressure would be 100 mmHg.
A deeper exploration into blood pressure regulation reveals the importance of baroreceptors—specialized nerve cells in the carotid arteries and aorta that detect changes in blood pressure. When a sudden drop in blood pressure occurs, baroreceptors stimulate the autonomic nervous system to increase heart rate and constrict blood vessels, thereby raising blood pressure.Additionally, the renin-angiotensin-aldosterone system (RAAS) plays a vital role. When blood pressure drops, the kidneys release renin, an enzyme that triggers the production of angiotensin II, which constricts blood vessels and stimulates aldosterone release, leading to increased blood volume and pressure.
Understanding how the RAAS works can help in comprehending the treatment of hypertension, as many drugs target this system to lower blood pressure.
Cardiac Output and Stroke Volume
Understanding the concepts of cardiac output and stroke volume is essential in the study of hemodynamics. These terms describe key components of heart function and their impact on blood circulation. This section will help you explore how they contribute to efficient cardiovascular activity.
Cardiac Output Explained
Cardiac Output (CO) is a crucial measurement that represents the volume of blood the heart pumps in one minute. It is calculated by multiplying heart rate (HR) and stroke volume (SV). The formula is expressed as:\[CO = SV \times HR\]
- Heart Rate (HR): The number of heartbeats per minute.
- Stroke Volume (SV): The amount of blood pumped by the left ventricle of the heart in one contraction.
For example, if an individual's heart rate is 70 beats per minute, and the stroke volume is 70 mL, the cardiac output would be:\[CO = 70 \, \text{mL/beat} \times 70 \, \text{beats/minute} = 4900 \, \text{mL/minute} = 4.9 \, \text{L/minute}\]This calculation illustrates how changes in heart rate or stroke volume can affect cardiac output.
It’s interesting to note that cardiac output does not just increase linearly with exercise; other factors like stroke volume and heart contractility change as well. During intense exercise, both heart rate and stroke volume typically increase, but they do so to different extents.The body can modulate cardiac output through mechanisms like the Starling's law, which states that the stroke volume of the heart increases with an increase in blood volume filling the heart (end-diastolic volume) up to a certain point. This occurs because the fibers in the heart muscle stretch and can contract more forcefully.In conditions such as heart failure, where the heart cannot pump effectively, understanding and adjusting cardiac output can improve patient outcomes. Medications and lifestyle changes aim to optimize cardiac output and reduce symptoms.
Understanding Stroke Volume
Stroke volume (SV) is an important parameter that reflects the quantity of blood ejected by the heart's left ventricle in one contraction. Understanding stroke volume helps in evaluating cardiac function and circulatory efficiency. It is influenced by three primary factors:
- Preload: The initial stretching of the cardiac myocytes prior to contraction, which largely depends on venous return.
- Contractility: The inherent vigor of myocardial contraction, influenced by autonomic regulation and calcium availability.
- Afterload: The resistance that the heart must overcome to eject blood.
Consider the stroke volume calculation:Suppose the end-diastolic volume is 120 mL, and the end-systolic volume is 50 mL. Then the stroke volume would be:\[SV = 120 \, \text{mL} - 50 \, \text{mL} = 70 \, \text{mL/beat}\]This example helps demonstrate how measurements of end-diastolic and end-systolic volumes contribute to stroke volume calculations.
A comprehensive understanding of stroke volume includes recognizing how it’s affected by factors such as temperature, body position, and pathology. For instance, warm environments or fever can increase heart rate and stroke volume, whereas certain cardiac conditions and medications can diminish them.Moreover, exercise significantly impacts stroke volume. Initially, stroke volume rises due to increased venous return and sympathetic stimulation, enhancing both contractility and heart rate. As exercise continues, heart rate predominates in driving cardiac output.
Hemodynamic Monitoring Techniques
Hemodynamic monitoring techniques are essential tools in understanding and assessing cardiovascular function in clinical settings. These techniques provide critical data on blood pressure, cardiac output, and overall circulatory health. They are widely used in intensive care units, surgical settings, and to manage patients with cardiovascular diseases.Understanding these techniques can help in the diagnosis and treatment of various conditions related to the heart and blood vessels.
Invasive Hemodynamic Monitoring
Invasive monitoring involves inserting catheters directly into blood vessels or the heart to obtain precise physiological data. Some common types of invasive hemodynamic monitoring include:
- Arterial Catheterization: Provides continuous blood pressure monitoring and allows for arterial blood sampling.
- Pulmonary Artery Catheterization: Also known as a Swan-Ganz catheter, it measures cardiac output, pulmonary artery pressures, and other critical parameters.
- Central Venous Catheterization: Measures central venous pressure (CVP), reflecting right ventricular function and venous return.
Consider a patient in shock where a pulmonary artery catheter is used:This catheter helps measure cardiac output and pulmonary pressures, guiding treatment plans that can stabilize the patient by adjusting fluid therapy or medications.
Ensure that invasive monitoring is justified by clear clinical needs due to its potential complications.
Non-Invasive Hemodynamic Monitoring
Non-invasive methods offer safer alternatives to invasive techniques, reducing risks associated with catheters while still providing valuable insights into a patient's cardiovascular status. Key non-invasive monitoring methods include:
- Echocardiography: Uses ultrasound to visualize heart structures and assess blood flow.
- Electrical Cardiometry: Estimates cardiac output through skin electrodes measuring thoracic bioimpedance.
- Doppler Ultrasound: Measures blood flow velocity in specific vessels, aiding in assessing cardiac output and peripheral circulation.
A popular non-invasive technique is the use of impedance cardiography. This method assesses the cardiac function by measuring changes in electrical impedance across the thorax during the cardiac cycle. Impedance cardiography offers insight into volumetric aspects of cardiac performance and fluid status, beneficial in settings where quick and repeatable measurements are critical.Recent advancements include wearable devices that integrate photoplethysmography (PPG) with other sensors, providing continuous, real-time monitoring of heart rate and blood oxygen levels, without the need for heavy equipment or extensive setup.
hemodynamics - Key takeaways
- Hemodynamics Definition: Study of blood flow within the circulatory system, focusing on pressure, flow, and resistance.
- Blood Pressure Regulation: Blood pressure is essential for delivering oxygen and nutrients, managed by nervous and renal systems, and hormones.
- Cardiac Output: Volume of blood the heart pumps per minute, calculated as heart rate multiplied by stroke volume.
- Stroke Volume: Amount of blood pumped with each heartbeat, influenced by preload, contractility, and afterload.
- Hemodynamic Monitoring: Techniques to assess cardiovascular function, involving both invasive methods (arterial, central venous catheterization) and non-invasive methods (echocardiography, electrical cardiometry).
- Hemodynamics Physiology: Explores dynamic blood flow aspects, cardiovascular health, and disease management.
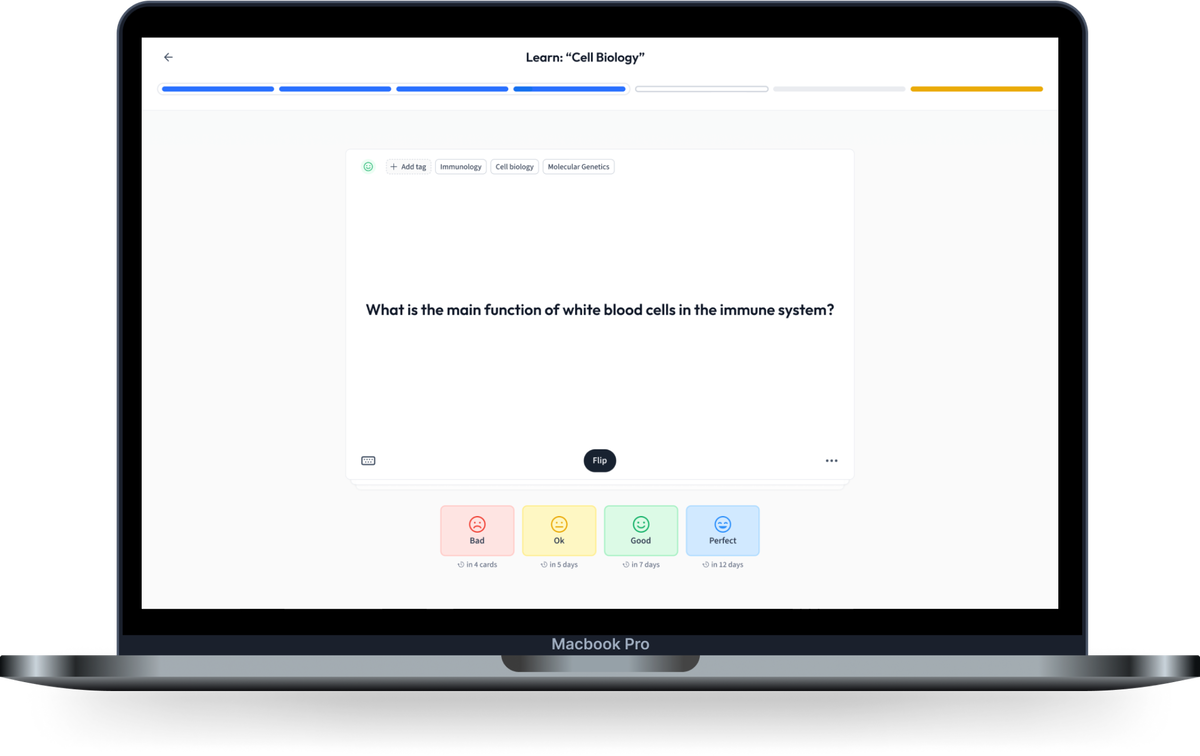
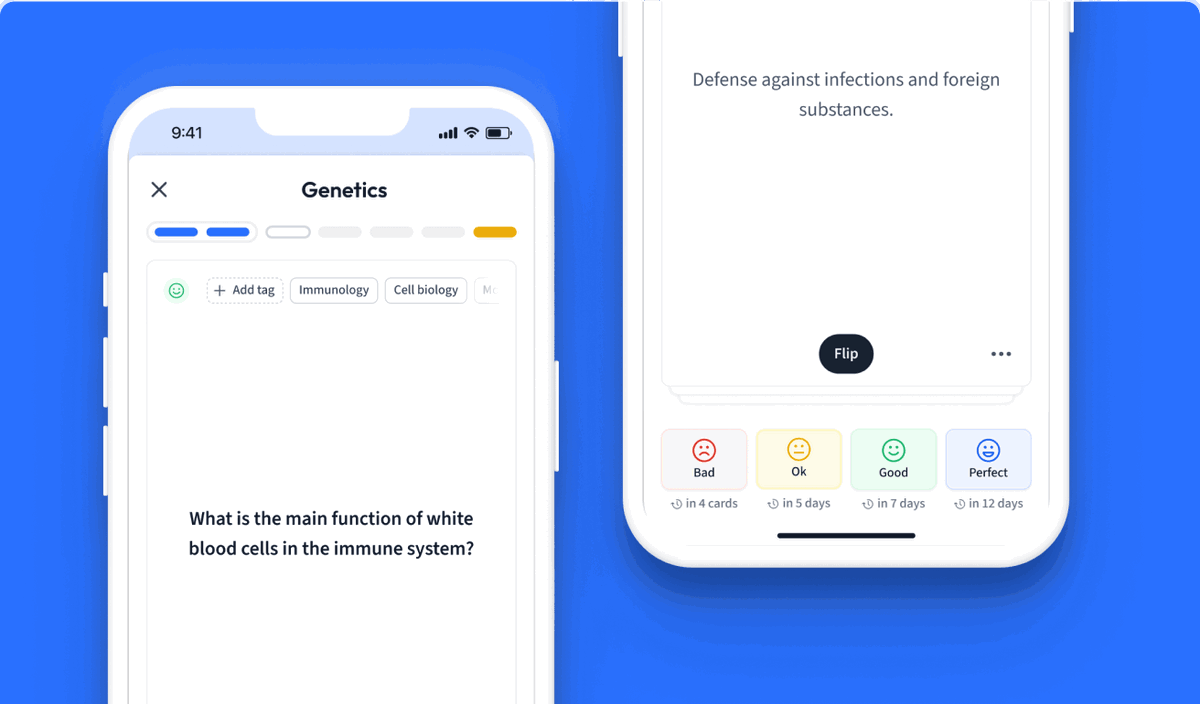
Learn with 10 hemodynamics flashcards in the free StudySmarter app
Already have an account? Log in
Frequently Asked Questions about hemodynamics
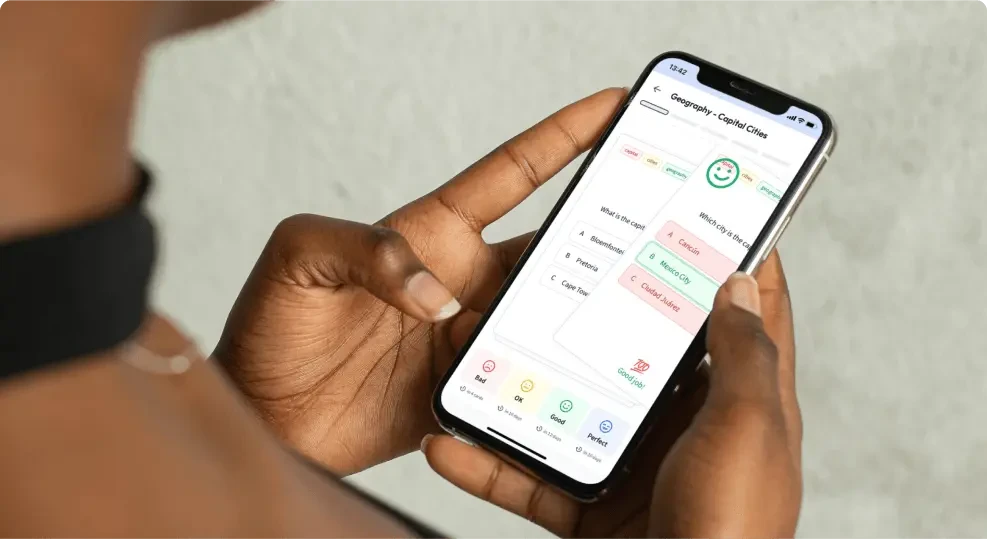
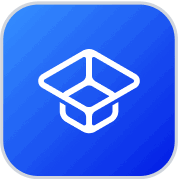
About StudySmarter
StudySmarter is a globally recognized educational technology company, offering a holistic learning platform designed for students of all ages and educational levels. Our platform provides learning support for a wide range of subjects, including STEM, Social Sciences, and Languages and also helps students to successfully master various tests and exams worldwide, such as GCSE, A Level, SAT, ACT, Abitur, and more. We offer an extensive library of learning materials, including interactive flashcards, comprehensive textbook solutions, and detailed explanations. The cutting-edge technology and tools we provide help students create their own learning materials. StudySmarter’s content is not only expert-verified but also regularly updated to ensure accuracy and relevance.
Learn more