Jump to a key chapter
Biofabrication Techniques Explained
Biofabrication is an exciting field at the intersection of biology, materials science, and engineering, offering innovative solutions to produce complex biological products. These products can range from tissues and organs to chemical compounds that have specific applications in medicine.
What is Biofabrication?
Biofabrication is an interdisciplinary approach that combines biological and engineering principles to produce structures, devices, or systems. It's instrumental in creating materials that mimic natural tissues.
Biofabrication involves using living cells, biomaterials, and bioactive molecules. It's a sophisticated process with varied techniques including:
- 3D Bioprinting: A technique which uses a 3D printer to create structures by depositing layers of living cells and biomaterial.
- Electrospinning: A method that creates fibers from polymer solutions or melts by applying a high-voltage electrical field.
- Microfluidics: This utilizes the manipulation of fluids at a micro-scale to develop intricate biological structures.
3D Bioprinting
3D bioprinting is perhaps the most well-known biofabrication technique. This method allows for the precise placement of cells and materials, layer by layer, to develop three-dimensional structures. The printed structures can be used in medical research and have the potential to replicate tissues or even organs.
An example of 3D bioprinting is creating a bioprinted skin graft for burn victims. By using the patient's own cells, doctors can print a new layer of skin that is less likely to be rejected by the body.
The process of 3D bioprinting begins with creating a digital model using CAD software. The model is then sliced into layers, and these instructions are sent to the bioprinter. Bioinks, which are typically composed of a mixture of cells and supportive scaffolding materials, are loaded into the printer. The bioprinter follows the digital model's instructions to deposit layers of bioink, forming the desired structure. This process ensures that the biological material remains viable and functional post-printing.
Applications of Biofabrication
Beyond the creation of tissues and organs, biofabrication has vast applications in regenerative medicine, drug testing, and the production of various biomaterials. It plays a crucial role in personalized medicine, allowing treatments and materials to be tailored to individual patients' needs.
In the field of drug testing, biofabrication enables the creation of organ-on-a-chip devices. These devices mimic the architecture and function of human organs, providing a more accurate testing platform compared to traditional methods.
Biofabrication is paving the way towards sustainable and ethical production of meat alternatives, offering potential solutions for the world’s growing food demands.
3D Bioprinting in Dentistry
The field of dentistry is experiencing a revolution thanks to 3D bioprinting. This advanced technology allows for the creation of dental components with high precision and personalization, directly impacting dental treatments and patient outcomes. By utilizing 3D bioprinting, dentists can create customized dental implants, crowns, and even bone structures that facilitate complex dental reconstructions.
Applications of 3D Bioprinting in Dentistry
3D bioprinting offers a range of applications in modern dentistry. Some notable applications include:
- Dental Implants: These can be customized to fit a patient's unique jaw structure, promoting better integration.
- Bone Regeneration: 3D bioprinting can create scaffolds that support bone growth, crucial for patients requiring significant reconstructive procedures.
- Soft Tissue Printing: The technology is also used to print soft tissues, assisting in treatments that require gum repairs or enhancements.
An example of 3D bioprinting in action is the development of a bioprinted jawbone. By using the patient's own cells to create the jawbone, the risk of rejection significantly decreases, and the healing process becomes faster and more efficient.
The process begins with a detailed 3D scan of the patient's dentition. Using this data, a digital model is constructed and fed into the bioprinter. The bioink used often contains a mixture of the patient's cells and a biocompatible polymer scaffold. This mix is layered meticulously by the printer to form the final dental structure. Advanced software ensures precision in placement, which is critical for ensuring the functional and aesthetic quality of the prosthetic or enhancement. This careful process can potentially revolutionize dental surgeries by reducing recovery times and improving success rates.
Benefits of 3D Bioprinting in Dentistry
The benefits of integrating 3D bioprinting into dentistry are profound:
- Enhanced personalization of dental devices, leading to more comfortable and effective treatments.
- Reduction in production time, allowing for quicker dental solutions.
- Potentially lower costs due to streamlined production processes.
- Use of biocompatible materials reduces the risk of adverse reactions.
3D bioprinting can significantly improve outcomes in orthodontics, offering printed aligners that fit perfectly to each patient's dental anatomy.
Scaffold Fabrication for Dental Applications
Scaffold fabrication is a cornerstone of modern dental applications, providing the necessary structure to support cell growth and tissue regeneration. This technique is invaluable in creating supportive frameworks, especially in complex dental restorations and regenerative therapies.
Understanding Scaffold Fabrication
Scaffold fabrication involves creating a three-dimensional structure that mimics the extracellular matrix of tissues. It serves as a template for tissue formation and is crucial in regenerative medicine.
Different materials and techniques are employed in scaffold fabrication to meet the diversity of dental needs. Key materials used include:
- Biopolymers: Materials like collagen and chitosan, which are biocompatible and promote cell attachment.
- Ceramics: Such as hydroxyapatite, ideal for bone regeneration due to their structural similarity to natural bone.
- Composites: A combination of materials to optimize mechanical strength and biological function.
Applications in Dentistry
In dentistry, scaffolds are used to facilitate the growth and repair of dental tissues. They play a crucial role in:
- Bone Regeneration: Scaffolds provide a framework for new bone growth, essential in jawbone reconstruction and implant placement.
- Soft Tissue Regeneration: Supporting gum tissue growth in periodontal treatments.
- Guided Tissue Regeneration (GTR): Using membranes to direct growth in a desired area, preventing unwanted tissue invasion.
In guided bone regeneration, a scaffold is placed over a bone defect. Over time, new bone cells populate the scaffold, gradually transforming it into a natural bone structure, which is vital for successful dental implant procedures.
Electrospinning is particularly notable in scaffold fabrication for its ability to create nanofibrous structures. These structures closely mimic the natural extracellular matrix, enhancing cell adhesion and proliferation. The process involves applying a high voltage to a polymer solution, causing nanoparticles to be drawn out and solidified into fibers. These fibers can then be arranged to form complex three-dimensional scaffolds. The advantage of electrospun scaffolds lies in their high surface area, porosity, and the ability to load them with various bioactive compounds, promoting targeted healing and regeneration.
Scaffold design in dental applications often incorporates growth factors to accelerate tissue regeneration and healing.
Tissue Engineering and Organ Printing
In the realm of medical science, tissue engineering and organ printing represent revolutionary advancements. These techniques hold the potential to address the shortage of organs available for transplantation and improve the quality of patient care. Tissue engineering involves the fabrication of living tissue, while organ printing is the creation of fully functional organs through a process similar to 3D bioprinting.
Biofabrication Methods Overview
Biofabrication methods are a suite of techniques used to create bioengineered products by combining cells, biomaterials, and biological molecules. These methods aim to produce functional biological systems or tissues that can replace or support failing human tissues.
Several biofabrication techniques underpin this field:
- 3D Bioprinting: Uses layer-by-layer deposition of cells and biomaterials to create complex structures.
- Electrospinning: Generates fibrous scaffolds through electric fields from polymer-based solutions.
- Lithography: Includes light or electron beam methods to pattern biomaterials at micro and nano scales.
- Self-assembly: Utilizes the natural ability of molecules to assemble into predetermined structures.
Biofabrication minimizes the risk of rejection by utilizing a patient's cells, leading to customized biological structures. The integration of mathematical modeling enhances the design precision and functionality of the fabricated tissues. For example, the mechanical properties of vascular structures can be modeled using:\[E = \frac{\text{stress}}{\text{strain}} = \frac{\frac{F}{A}}{\frac{\text{change in length}}{\text{original length}}}\]where - F is the force applied,- A is the cross-sectional area, - E denotes Young's modulus.
Advances in Organ Printing
Recent technological advances have dramatically impacted the field of organ printing. The goal is to engineer organs that are not only structurally accurate but also functional at physiological levels. This innovation is powered by developments in bioink formulations, printer technology, and post-printing conditioning of the printed tissues.
One significant advancement is the successful printing of a biocompatible heart valve. This demonstration used a combination of cells and hydrogel, engineered to replicate the mechanical and biological functions of a natural heart valve.
Continuous development in hydrogel technology is critical in organ printing to mimic the complex environment of the human body's extracellular matrix.
Applications of 3D Bioprinting
3D bioprinting is revolutionizing areas such as regenerative medicine, drug development, and clinical research. Here are several applications:
- Customized Implants: Tailored to individual anatomy to improve integration and functionality.
- Tissue Regeneration: Enabling growth of skin, bone, and cartilage tissues for transplantation.
- Drug Testing: Creating disease models to test pharmaceutical compounds effectively and ethically.
In personalized medicine, 3D bioprinted cancer models allow for the testing of treatment efficacy, avoiding trial and error on actual patients and reducing overall treatment time.
Scaffold Fabrication Techniques
Scaffolds are critical in supporting cell attachment and growth, and there are various techniques for fabricating them:
- Freeze Drying: Produces porous structures ideal for cell growth.
- Salt Leaching: Utilizes porogen removal to create porous scaffolds.
- Stereolithography: Laser-based technique for creating precise microstructures.
A scaffold fabricated through salt-leaching supports bone tissue growth following a fracture. The porosity allows for nutrient circulation, promoting rapid regeneration.
Matching the scaffold composition with tissue characteristics is crucial for effective tissue engineering.
Role of Biofabrication in Tissue Engineering
In tissue engineering, biofabrication provides a versatile platform to create biological substitutes that restore, maintain, or improve tissue function. This involves using biofabrication techniques to create tissue models that closely mimic human physiology, allowing for effective study and treatment of diseases.
The role of bioprinting in tissue engineering allows for precise control over cell position, density, and spatial distribution. Mathematical models such as the reaction-diffusion equation can predict concentration profiles in tissues to ensure nutrient diffusion and waste removal are optimized:\[\frac{\text{d}C}{\text{d}t} = D abla^2 C + R\]where:- C represents concentration,- D is the diffusion coefficient,- R is the rate of reaction.
biofabrication techniques - Key takeaways
- Biofabrication Techniques: An interdisciplinary approach integrating biological and engineering principles to fabricate complex structures and devices.
- 3D Bioprinting: A method of creating three-dimensional structures by layering living cells and biomaterials, used for precise cell placement.
- Scaffold Fabrication: The creation of three-dimensional structures to mimic extracellular matrices, supporting cell growth and tissue regeneration.
- Tissue Engineering: The use of biofabrication techniques such as bioprinting to restore or enhance tissue functionality.
- Organ Printing: A specific application of 3D bioprinting focused on creating functional organs for transplantation and research.
- Biofabrication Methods Overview: Includes 3D bioprinting, electrospinning, lithography, and self-assembly techniques to produce biological constructs.
Learn faster with the 12 flashcards about biofabrication techniques
Sign up for free to gain access to all our flashcards.
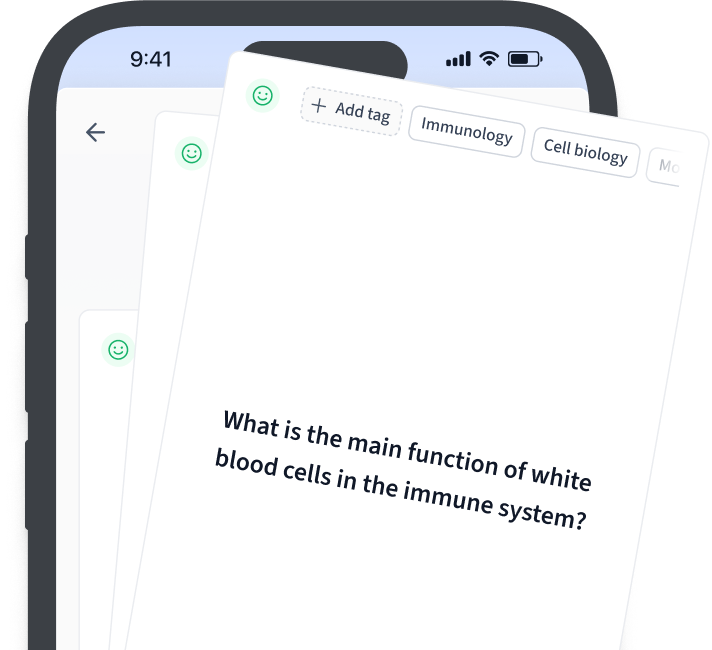
Frequently Asked Questions about biofabrication techniques
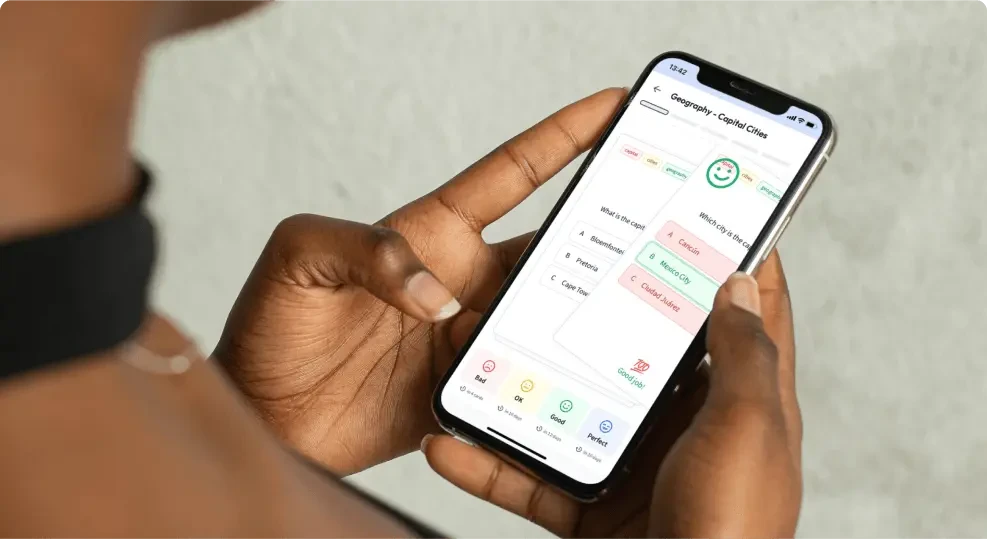
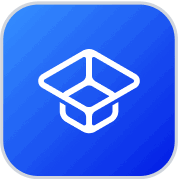
About StudySmarter
StudySmarter is a globally recognized educational technology company, offering a holistic learning platform designed for students of all ages and educational levels. Our platform provides learning support for a wide range of subjects, including STEM, Social Sciences, and Languages and also helps students to successfully master various tests and exams worldwide, such as GCSE, A Level, SAT, ACT, Abitur, and more. We offer an extensive library of learning materials, including interactive flashcards, comprehensive textbook solutions, and detailed explanations. The cutting-edge technology and tools we provide help students create their own learning materials. StudySmarter’s content is not only expert-verified but also regularly updated to ensure accuracy and relevance.
Learn more