Jump to a key chapter
Biomaterial Characterization Basics
In the field of medicine, understanding the properties and behaviors of materials used for biological purposes is essential. This understanding is broadly known as biomaterial characterization. It involves the assessment of physical, chemical, mechanical, and biological aspects of materials deemed suitable for medical applications.
Importance of Biomaterial Characterization
The characterization of biomaterials is crucial for several reasons. It ensures that materials are safe and effective for use in medical applications. Here are some key points to highlight the importance of biomaterial characterization:1. Safety Assurance: One of the primary goals is to ensure the material does not provoke any adverse biological responses, such as toxicity or inflammation.2. Performance Evaluation: Characterization helps in predicting how a biomaterial will perform under physiological conditions.3. Compliance with Regulations: There are strict regulatory standards that biomaterials must meet before they can be used clinically. Characterization enables compliance with these standards.4. Customization: Through characterization, materials can be tailored to fit specific medical needs, such as developing biodegradable implants perpendicular to their healing time.5. Longevity and Stability: Ensuring the long-term stability of the material within the body is vital, and characterization helps gauge this property.
- It determines if a material is suitable for prolonged use inside the human body.
- It detects any potential degradation over time.
Biomaterial characterization often involves multidisciplinary collaboration, involving fields like chemistry, physics, biology, and engineering.
Common Biomaterial Characterization Methods
There are several methods employed in the characterization of biomaterials. Each method aims to analyze different properties integral to the biomaterial's function and compatibility. The following are some commonly used methods:
- Physical Characterization: Techniques like X-Ray Diffraction (XRD) and Scanning Electron Microscopy (SEM) are used to analyze a material's surface and crystal structure.
- Chemical Characterization: Methods such as Fourier Transform Infrared Spectroscopy (FTIR) and Mass Spectrometry provide insight into the composition and chemical bonding of a material.
- Mechanical Testing: This includes tensile testing, compression testing, and hardness measurements to understand the material's mechanical properties.
- Biological Evaluation: In vitro and in vivo testing are conducted to assess the biological responses induced by the biomaterial.
- Contact Angle Measurement: Utilized to determine the hydrophilicity or hydrophobicity of the biomaterial surface.
A contact angle indicates the wetting of a liquid on a solid surface and helps in determining surface characteristics essential for biomaterial applications.
A deeper understanding of mechanical testing includes evaluating stress-strain behaviors, which are critical for materials subjected to load-bearing environments. This can involve dynamic mechanical analysis (DMA), which measures the material's viscoelastic properties, offering insight into their behavior over a range of temperatures. Such detailed characterizations ensure the material's longevity and functionality in bodily conditions that may fluctuate.
Biomaterial Characterization Techniques
Characterizing biomaterials accurately is paramount for their application in the medical field. It involves comprehensive techniques to assess various properties, ensuring materials are suitable and effective for implantation and other uses.
Physical and Chemical Techniques
When characterizing biomaterials, physical and chemical techniques form the foundation. They help determine surface properties, structural integrity, and chemical composition. Some commonly used techniques include:
- Scanning Electron Microscopy (SEM): Provides high-resolution images to assess surface morphology and topography.
- X-Ray Diffraction (XRD): Used for identifying crystalline structures and understanding material phase composition.
- Fourier Transform Infrared Spectroscopy (FTIR): Analyzes chemical bonds and functional groups present within the biomaterial.
- Energy Dispersive X-Ray Analysis (EDAX): Complements SEM by providing elemental analysis and mapping.
- Mass Spectrometry: Allows determination of the molecular composition and structure.
For instance, using FTIR, researchers can ascertain the presence of specific functional groups like hydroxyl or carboxyl groups, which can indicate potential bonding sites for drug delivery applications.
Technique | Purpose |
SEM | Surface Imaging |
FTIR | Chemical Bond Analysis |
XRD | Crystalline Structure Identification |
Combining multiple techniques provides a more comprehensive understanding of a biomaterial's properties and behaviors.
Advanced Biomaterial Characterization Techniques
Beyond the basic methods, advanced characterization techniques delve deeper into understanding complex material behaviors and interactions in biological systems. These are crucial for high-precision applications like tissue engineering and regenerative medicine.
- Atomic Force Microscopy (AFM): Offers nanoscale surface topography and mechanical property measurements.
- Dynamic Mechanical Analysis (DMA): Examines viscoelastic properties, providing insight into how materials behave under biological conditions.
- Raman Spectroscopy: Achieves detailed chemical and structural information.
- Nuclear Magnetic Resonance (NMR): Provides information on molecular structure and dynamics.
- Time-of-Flight Secondary Ion Mass Spectrometry (ToF-SIMS): Enables surface analysis at the molecular level.
Advanced techniques like Atomic Force Microscopy (AFM) go beyond visualizing surface textures; they allow for the measurement of molecular forces on biomaterials. This capability is critical when considering interactions with proteins and cells, assisting in the design of surfaces that encourage cellular adhesion or detachment based on the application demands. Similarly, Dynamic Mechanical Analysis (DMA) provides insights into how a material might perform under cyclical loading, mimicking conditions like those experienced in joint replacements or cardiovascular implants. Such thorough investigations help refine biomaterials for optimal compatibility and performance in real-world applications.
Surface Characterization of Biomaterials
In biomaterial science, understanding the surface properties is crucial as they determine how a material interacts with its environment. Surface characterization plays a vital role in ensuring a biomaterial's compatibility and functionality in medical applications.
Techniques for Surface Characterization
Surface characterization techniques are employed to explore the physical and chemical nature of biomaterial surfaces. These techniques help in adapting materials for various medical applications.
- Atomic Force Microscopy (AFM): Provides detailed imaging of surface topography at the nanoscale, crucial for analyzing surface roughness and texture.
- Contact Angle Measurement: Determines the wetting behavior of the surface, indicating hydrophobicity or hydrophilicity essential for biomaterial-tissue interactions.
- X-Ray Photoelectron Spectroscopy (XPS): Allows for elemental and chemical state analysis of the biomaterial surface, crucial for evaluating surface composition.
- Ellipsometry: Measures thin film thickness and optical properties, providing data vital for applications like coatings or layers on implants.
For example, an AFM analysis might show how surface roughness can aid in osteoblast adhesion, improving the integration of an orthopedic implant.
Technique | Purpose |
AFM | Surface Topography |
XPS | Elemental Analysis |
Ellipsometry | Film Thickness Measurement |
The choice of surface characterization technique often depends on the specific properties needed for the application.
Applications of Surface Characterization
Applications of surface characterization extend across various sectors within medicine, from enhancing implant designs to developing drug delivery systems. Such applications rely on accurate surface property analysis to optimize performance.
- Orthopedic Implants: Characterizing surface roughness and hardness can aid in the development of implants with improved osseointegration.
- Dental Applications: Surface modification techniques, assessed via characterization methods, are crucial for enhancing adhesion properties of dental materials.
- Drug Delivery Systems: Ensuring precise surface chemistry enables the control of drug release rates and targets within the body.
- Biocompatibility Analysis: Surface energy studies help in predicting interactions with bodily fluids and tissues, crucial for biocompatibility.
In drug delivery, precise surface characterization can lead to the innovation of nanoparticle systems designed to encapsulate therapeutics. By modifying surface chemistry, nanoparticles can evade immune detection, allowing for sustained drug release within target sites. This is pivotal in the treatment of conditions where targeted therapy could vastly reduce side effects compared to non-specific drug administration methods. Advanced surface studies can provide insights into developing stimuli-responsive surfaces that release drugs under specific conditions, such as pH changes or temperature variations within the body.
Mechanical Properties of Biomaterials
The mechanical properties of biomaterials are critical as they determine how materials respond to physical forces in biological environments. They play an essential role in ensuring the material's compatibility and functionality when implanted or used in medical devices.
Assessing Mechanical Properties
Evaluating the mechanical properties of biomaterials involves various tests and assessments to ensure they meet the desired specifications for medical applications. This includes measures of strength, elasticity, and stiffness.
- Tensile Testing: Measures the material's resistance to tension, providing data on ultimate tensile strength and elongation.
- Compression Testing: Assesses how a material behaves under axial loads and its ability to endure compressive stress.
- Flexural Testing: Determines a material's flexural modulus and strength, which is crucial for predicting how it will perform when bent.
- Hardness Testing: Evaluates a material's resistance to deformation, crucial for applications exposed to wear and tear.
- Fatigue Testing: Checks the material's behavior under cyclic loading conditions to assess long-term durability.
The elastic modulus is a measure of a material's ability to resist deformation under stress. It's calculated using the formula \[E = \frac{\text{{stress}}}{\text{{strain}}}\], where stress is the force causing deformation divided by the area to which the force is applied, and strain is the deformation experienced by the material.
For instance, a biomaterial used for bone implants often requires a closer match to human bone's elastic modulus, typically around \( 14 - 30 \text{ GPa} \), to ensure efficient load transfer and integration without causing stress shielding.
In-depth analysis of mechanical properties can involve advanced techniques such as nanoindentation, which provides a detailed map of local mechanical properties at the micro and nano-scale. This is particularly valuable in composite biomaterials, where properties can vary significantly within the material matrix. Moreover, understanding viscoelastic properties can be essential for polymers used in soft tissue applications, where the material must deform under stress and recover upon removal, mimicking the natural viscoelasticity of human tissues.
Impact on Biomaterial Performance
The mechanical properties of biomaterials directly affect their performance and reliability in physiological environments. A comprehensive understanding of these properties ensures that materials are suitable for their intended applications, influencing both clinical outcomes and patient safety.Some factors influenced by mechanical properties include:
- Load Bearing Capacity: Essential for implants and devices expected to support or transmit loads, such as joint replacements or spinal implants.
- Durability and Longevity: Mechanical resilience ensures prolonged functionality, reducing the risk of material failure and need for replacement.
- Compliance and Compatibility: Ensures that materials mimic the mechanical properties of surrounding tissues, enhancing healing and integration. This is often quantified using properties such as the elastic modulus and tensile strength.
- Response to Physiological Conditions: Materials must withstand moisture, temperature fluctuations, and biochemical interactions without degradation.
Using analytical software for simulating mechanical properties can provide predictions about long-term material performance and identify potential points of failure before physical testing.
Chemical Analysis of Biomaterials
Chemical analysis is pivotal in understanding the composition, purity, and interactions of biomaterials with biological environments. This analysis ensures biomaterials meet the necessary safety and efficacy standards for medical applications.The chemical properties of biomaterials directly influence their biological interactions, including cellular response, biocompatibility, and degradation rates.
Techniques for Chemical Analysis
Different chemical analysis techniques provide varied insights into the composition and potential biological interactions of biomaterials.Here are some techniques commonly used in the field:
- Fourier Transform Infrared Spectroscopy (FTIR): Utilized for identifying functional groups and understanding molecular structures due to its high-resolution spectra.
- Mass Spectrometry: Offers qualitative and quantitative analysis of biomaterial composition, highly beneficial for contaminant analysis.
- Nuclear Magnetic Resonance (NMR) Spectroscopy: Provides detailed molecular structure data and dynamics information.
- Raman Spectroscopy: Complements FTIR by offering insights into the vibrational modes of molecules, aiding in the identification of molecular constituents.
- Energy Dispersive X-Ray Spectroscopy (EDX): Often used alongside electron microscopy to determine elemental composition and is particularly useful for surface characterization.
For example, FTIR might be employed to confirm the presence of specific functional groups that interact with physiological molecules, such as the phosphate groups indicating the potential for bone tissue engineering.
Technique | Application |
FTIR | Functional Group Identification |
NMR | Molecular Structure Analysis |
EDX | Elemental Composition |
In chemical analysis, NMR spectroscopy plays a crucial role in elucidating complex molecular structures, especially in polymers used for biodegradable applications. Through NMR, researchers can discern different monomer units within a polymer matrix, which is essential for tailoring biodegradability rates to match specific medical requirements, such as drug delivery systems or temporary implants. Detailed molecular insights allow for precise adjustments to molecular weight and branching, directly affecting the material's performance.
Role in Biomaterial Development
Chemical analysis is integral to every stage of biomaterial development, from initial design to final product testing. Its implications in biomaterial innovation and safety are vast and varied.
- Material Design: Offers insights into the chemical composition necessary for desired bioactivity and mechanical properties, guiding the initial selection of materials.
- Quality Control: Ensures conformity with purity standards, detecting potential impurities or contaminants that could affect biocompatibility.
- Biocompatibility Testing: Vital for predicting interactions with biological systems, ensuring the material will function as intended without adverse reactions.
- Regulatory Compliance: Chemical analysis proofs are often required for approval by governing bodies to confirm material safety and performance.
- Innovation in Medical Applications: Facilitate the discovery of novel biomaterials and composites, advancing applications in drug delivery, tissue engineering, and regenerative medicine.
Combining multiple chemical analysis techniques can provide a more comprehensive understanding of a biomaterial's properties, enhancing development outcomes.
biomaterial characterization - Key takeaways
- Biomaterial Characterization: The assessment of physical, chemical, mechanical, and biological properties of materials for medical applications.
- Biomaterial Characterization Techniques: Methods include physical, chemical, mechanical, and biological evaluations, using tools like SEM, FTIR, XRD, and mass spectrometry.
- Surface Characterization of Biomaterials: Focuses on techniques to explore surface properties crucial for biocompatibility and functionality in medical applications.
- Mechanical Properties of Biomaterials: Includes tensile, compression, and hardness testing to evaluate material response to physical forces.
- Chemical Analysis of Biomaterials: Involves techniques like FTIR and NMR to understand composition and biological interactions.
- Biomaterial Characterization Methods: Combine techniques such as physical imaging and chemical analysis to ensure materials are suitable for medical use and meet regulatory standards.
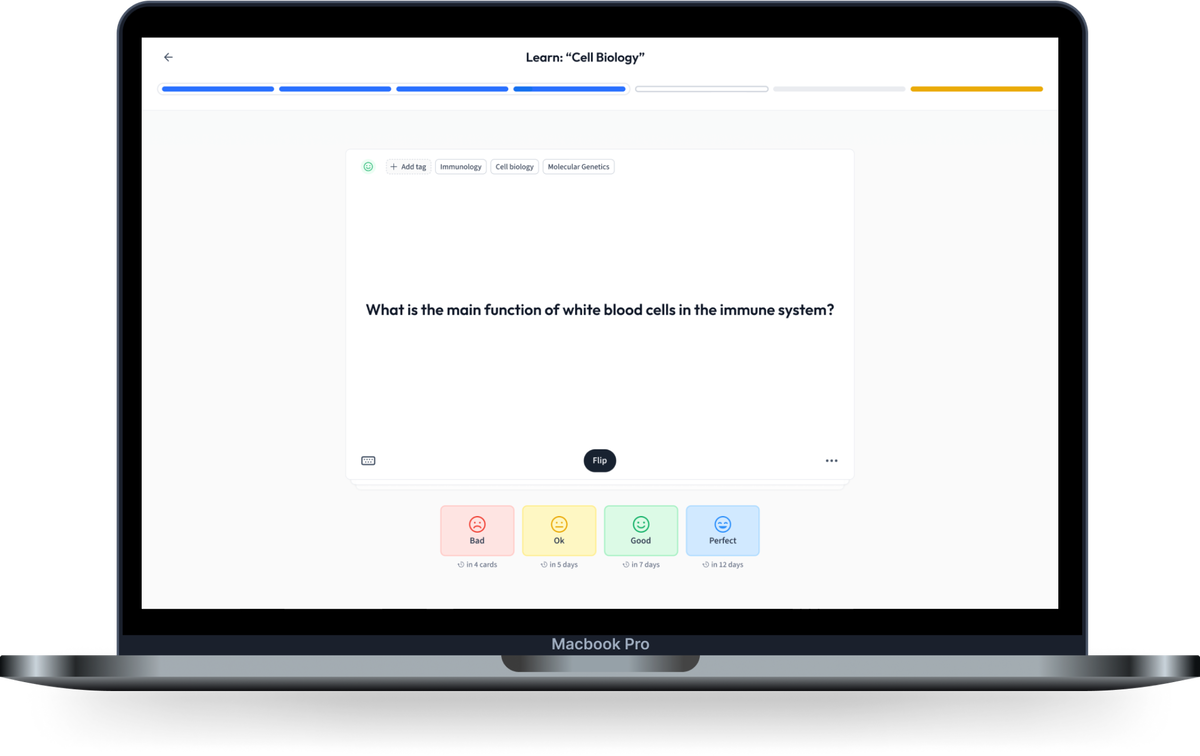
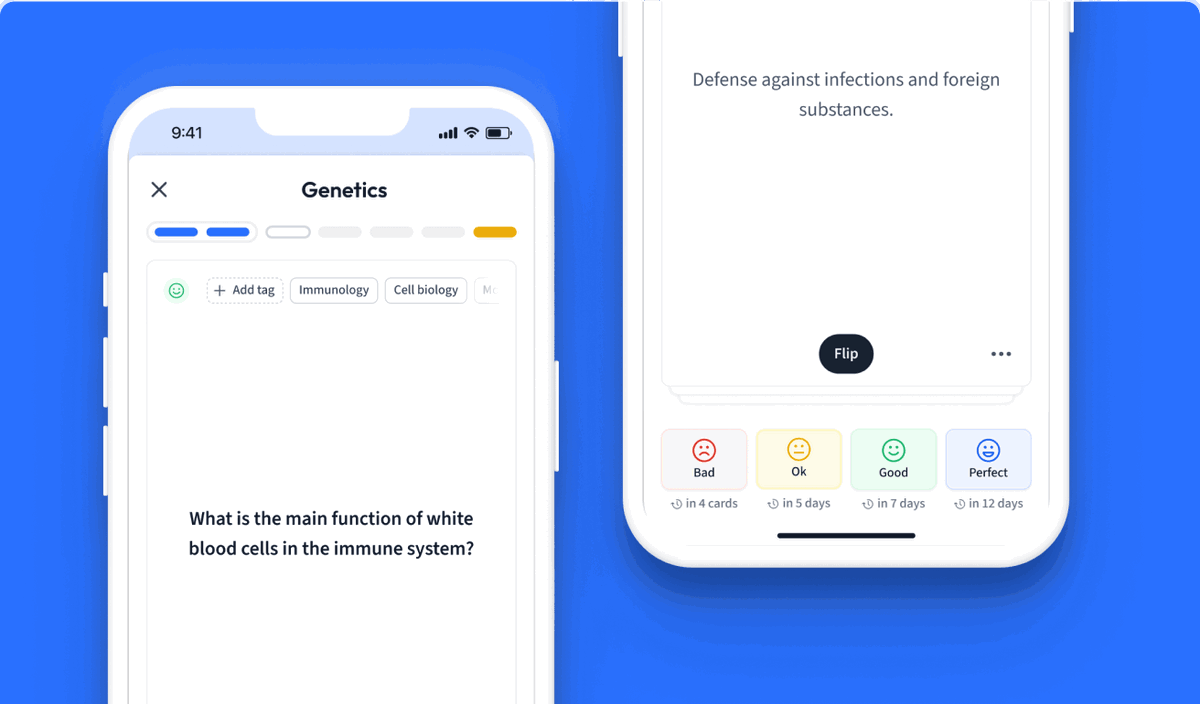
Learn with 10 biomaterial characterization flashcards in the free StudySmarter app
We have 14,000 flashcards about Dynamic Landscapes.
Already have an account? Log in
Frequently Asked Questions about biomaterial characterization
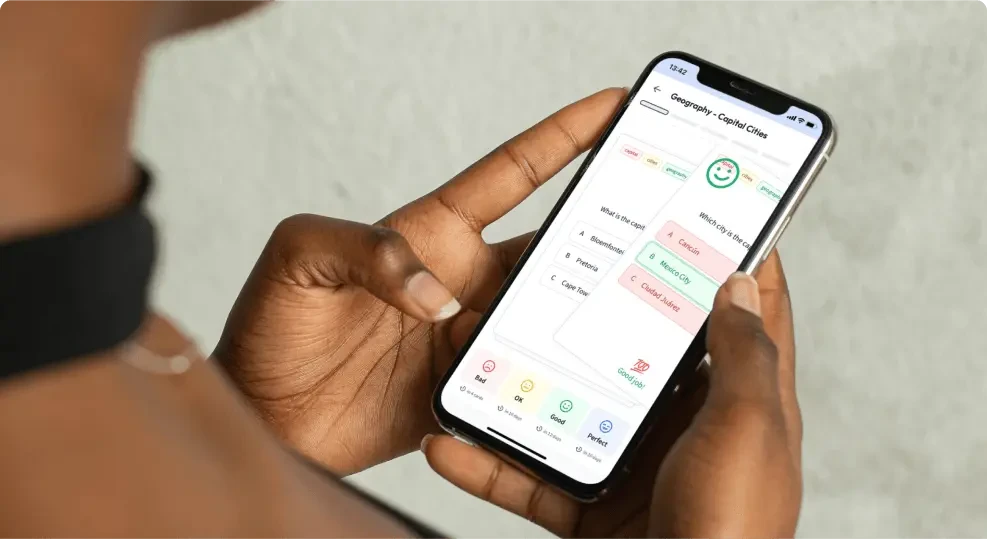
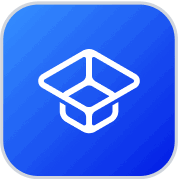
About StudySmarter
StudySmarter is a globally recognized educational technology company, offering a holistic learning platform designed for students of all ages and educational levels. Our platform provides learning support for a wide range of subjects, including STEM, Social Sciences, and Languages and also helps students to successfully master various tests and exams worldwide, such as GCSE, A Level, SAT, ACT, Abitur, and more. We offer an extensive library of learning materials, including interactive flashcards, comprehensive textbook solutions, and detailed explanations. The cutting-edge technology and tools we provide help students create their own learning materials. StudySmarter’s content is not only expert-verified but also regularly updated to ensure accuracy and relevance.
Learn more