Jump to a key chapter
Tissue Engineering Overview
Tissue engineering is a field within biomedical engineering that aims to develop biological substitutes to restore, maintain, or improve tissue function. It combines principles from biomaterials, cell biology, and engineering to create new structures that can replace damaged tissues. This innovative discipline is rapidly advancing, offering hope for numerous medical applications.
Definition of Tissue Engineering
Tissue Engineering is the science of designing and building tissues that can be used to treat medical conditions. It involves the use of a combination of cells, engineering materials, and suitable biochemical factors to improve or replace biological tissues.
The process generally involves four key steps:
- Cell sourcing: Obtaining cells that will form the new tissue.
- Scaffold fabrication: Creating a structure to support cell growth and tissue formation.
- Growth factor signaling: Using biochemical signals to guide tissue development.
- Tissue maturation: Allowing the cells to grow and function as intended.
A well-known example of tissue engineering is the creation of artificial skin for burn victims.
Applications in Dentistry
In dentistry, tissue engineering can significantly impact the treatment of oral conditions. It provides solutions for conditions that require tissue regeneration, such as:
- Periodontal disease
- Bone regeneration for dental implants
- Oral mucosa repair
Biological Scaffolds | Support cell growth, provide structure |
Growth Factors | Regulate cell differentiation |
Stem Cells | Differentiate into necessary cell types |
Computational Modeling | Simulates tissue growth |
An example of dental tissue engineering is using scaffolds infused with growth factors to encourage bone formation around dental implants. This process helps stabilize the implant, ensuring its long-term success.
The intersection of tissue engineering with nanotechnology is poised to revolutionize dentistry. Nanotechnology enables the fabrication of incredibly small-scale materials and devices that mimic natural tissue structures more closely. These nanoscale scaffolds can deliver medications or growth factors to specific sites more effectively, potentially accelerating healing and improving patient outcomes. Moreover, by controlling the scaffold's surface at the nanoscale, scientists can precisely direct cellular behavior, enhancing the success of tissue regeneration.
Tissue Engineering and Regenerative Medicine
Tissue engineering is revolutionizing the field of regenerative medicine by providing innovative solutions to repair, replace, or regenerate damaged tissues in the body. This interdisciplinary approach integrates principles of biomaterials science and engineering with cell biology to achieve its goals.
Connecting Dentistry and Regenerative Medicine
Regenerative medicine is increasingly making impactful contributions in the field of dentistry. With the advent of tissue engineering, dental professionals can now explore advanced methods to address oral health issues. This includes the regeneration of dental tissues such as gums and alveolar bone.The integration of regenerative medicine in dentistry allows for the utilization of techniques like:
- Stem cell therapy: Employing cells that can develop into various cell types needed for tissue repair.
- Scaffold use: Creating supportive structures that guide cell growth and tissue architecture.
- Biochemical signals: Applying growth factors that regulate cellular activities necessary for tissue regeneration.
For instance, in the treatment of jawbone defects due to tooth loss, tissue-engineered scaffolds can be used. These scaffolds, combined with growth factors, enhance bone regeneration, providing a sturdy base for dental implants.
A captivating area of study is the use of bioprinting in dentistry. This technology involves creating 3D structures layer-by-layer using biocompatible materials and living cells. The possibility of printing entire dental structures, potentially even a whole tooth, is on the horizon. This would enable custom solutions tailored to each patient's anatomical contours, resulting in a higher success rate of dental procedures. The ongoing research in bioprinting highlights the potential for unprecedented precision in dental restoration.
Benefits for Dental Health
Tissue engineering offers numerous benefits for dental health. As traditional dental treatments focus on repair and maintenance, regenerative methods aim to restore natural functionality. Here are some promising benefits:
- Enhanced healing: Faster recovery times due to the regeneration of tissues rather than synthetic replacements.
- Reduced complications: Lower risk of infection and side effects as biocompatible materials are used.
- Improved aesthetics: Natural regeneration results in better overall appearance compared to conventional methods.
In dental regeneration, the use of platelet-rich plasma (PRP) can accelerate the healing process, leveraging the patient's own growth factors to promote tissue repair.
Tissue Engineering Techniques
Tissue engineering involves a diverse range of techniques aimed at creating biological substitutes to restore damaged tissues. These techniques incorporate the use of scaffolds, biomaterials, and cells to replicate the structure and function of natural tissues. Let's explore some of these innovative approaches.
Scaffold Tissue Engineering
Scaffold tissue engineering plays a crucial role in providing a three-dimensional structure that supports cell attachment and tissue formation. Scaffolds serve as the physical support for cells to adhere, proliferate, and form the desired tissue structure.
A scaffold is a three-dimensional matrix that provides structural support for cell growth and tissue development. It mimics the extracellular matrix (ECM) of natural tissue, guiding cell organization and differentiation.
When designing scaffolds, several factors are considered:
- Biocompatibility: The scaffold must not provoke an immune response in the body.
- Mechanical properties: It should match or possess the strength of the surrounding tissue.
- Porosity: High porosity allows for cell migration and nutrient transport.
The development of hydrogel scaffolds is an exciting area of research. Hydrogels can adapt to irregular wound shapes and gradually degrade, allowing for natural tissue replacement without the need for surgical removal. By incorporating growth factors into these hydrogels, targeted tissue regeneration can be achieved more efficiently.
Biomaterials in Tissue Engineering
Biomaterials are essential in tissue engineering as they form the building blocks for constructing scaffolds and other components. These materials must be compatible with biological systems and promote desired cellular responses.
An example of biomaterial use includes poly(lactic-co-glycolic acid) (PLGA), widely used due to its biocompatibility and controlled degradation characteristics, making it suitable for temporary scaffolds.
Common types of biomaterials used include:
- Polymers: Synthetic options like PLGA and natural polymers like collagen.
- Metals: Titanium is often used for its strength in dental implants.
- Bioceramics: Used in hard tissue applications due to their bone-mimicking properties.
Cells in Tissue Engineering
Cells are a fundamental component of tissue engineering, responsible for performing the biological functions of the engineered tissue. The choice of cells is critical, as it influences the tissue's ability to integrate and function effectively.
In the context of tissue engineering, stem cells are undifferentiated cells with the potential to develop into various specialized cell types, making them ideal for regenerating damaged tissues.
Adult stem cells can be sourced from tissues such as bone marrow and adipose tissue, providing a versatile cell option for tissue engineering.
Types of cells commonly used include:
- Stem cells: Due to their pluripotency and ability to differentiate into various cell types.
- Primary cells: Harvested from tissues to closely match the target tissue type.
- Immortalized cell lines: Engineered for indefinite proliferation, useful in research applications.
Bone Tissue Engineering
Bone tissue engineering involves creating constructs that stimulate the growth of new bone tissue to repair or replace damaged bone. This field combines the principles of biology and engineering to develop materials and techniques that promote bone regeneration. Let's delve into the techniques and materials utilized in this innovative field.
Techniques in Bone Tissue Engineering
Various techniques are employed in bone tissue engineering to achieve the goal of effective bone regeneration. These approaches leverage modern advancements in technology and biological sciences:
- 3D Bioprinting: This technique involves printing cells and biomaterials to create a bone-like structure, allowing precise customization to fit the defect site.
- Osteoinductive Strategies: Using growth factors that stimulate osteogenesis, the formation of new bone cells.
- Scaffold Fabrication: Designing biodegradable scaffolds that mimic the extracellular matrix of bone, providing structural support for new tissue formation.
The use of gene therapy in bone tissue engineering is an emerging technique. By delivering specific genes that encode growth factors directly to the cells at the bone defect site, this approach aims to enhance the regenerative capacity and speed up the healing process. This method holds promise for treating non-healing bone fractures and large skeletal defects that are difficult to manage with traditional therapies.
Biomaterials for Bone Regeneration
Biomaterials form the foundation of bone tissue engineering by serving as the scaffolding on which bone regeneration occurs. The selection of appropriate biomaterials is crucial to the success of the regeneration process.
Type of Biomaterial | Characteristics and Uses |
Bioceramics | High compressive strength, osteoconductive; used in bone grafts |
Biopolymers | Biodegradable, good mechanical properties; utilized for scaffolds |
Metallic Biomaterials | Durable, high strength; often used in load-bearing implants |
Bioceramics like hydroxyapatite closely resemble the mineral component of bone, making them highly effective for bone regeneration applications.
Each type of biomaterial offers specific advantages through properties such as:
- Biocompatibility: Ensures that the material does not induce an adverse reaction in the body.
- Osteoconductivity: Provides a supportive environment for bone growth.
- Degradability: Allows the material to gradually dissolve as new bone forms, reducing long-term foreign presence in the body.
tissue engineering - Key takeaways
- Tissue engineering is a biomedical field aimed at developing biological substitutes to restore or improve tissue function, integrating biomaterials, cell biology, and engineering principles.
- Tissue engineering techniques involve key steps: cell sourcing, scaffold fabrication, growth factor signaling, and tissue maturation, to guide tissue development.
- Tissue engineering is a vital part of regenerative medicine, which focuses on repairing or regenerating damaged tissues, with applications in dentistry like periodontal disease and bone regeneration.
- Biomaterials in tissue engineering include polymers, metals, and bioceramics, selected for properties like biocompatibility, mechanical strength, and osteoconductivity.
- Cells in tissue engineering, such as stem cells, primary cells, and immortalized cell lines, are crucial for performing biological functions and ensuring successful tissue integration.
- Scaffold tissue engineering provides 3D structures for cell attachment, important for bone tissue engineering, to mimic natural extracellular matrices and guide new tissue formation.
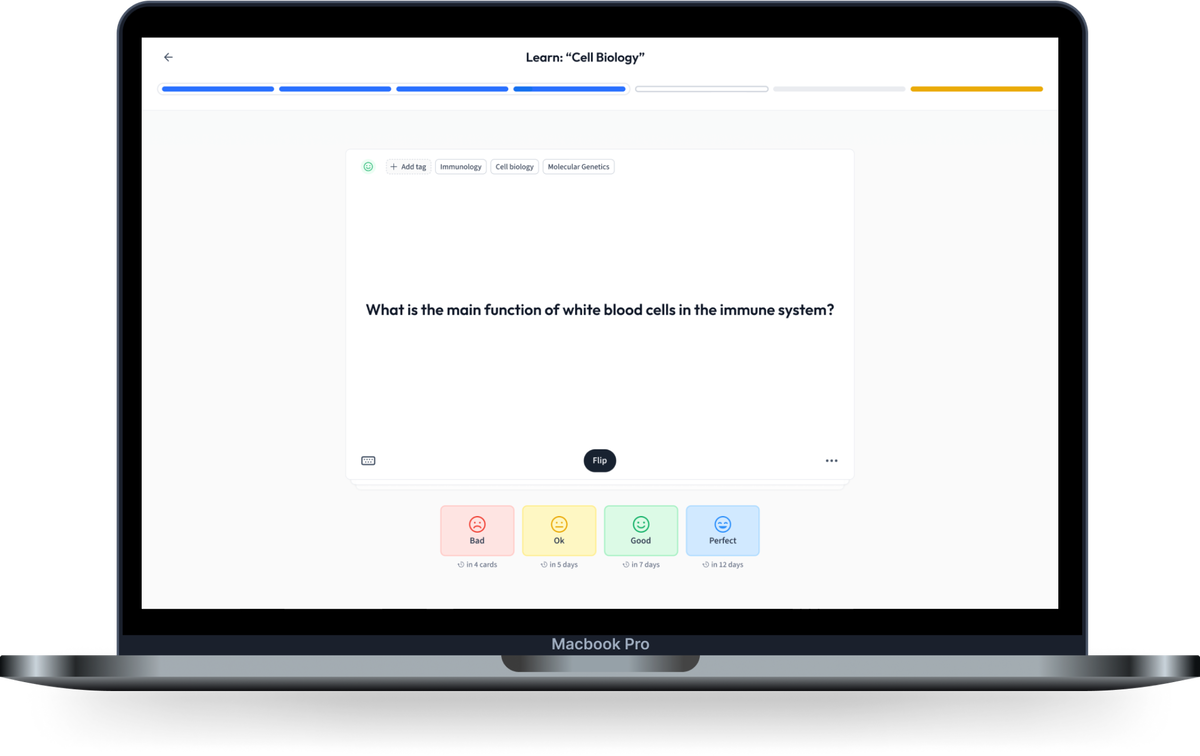
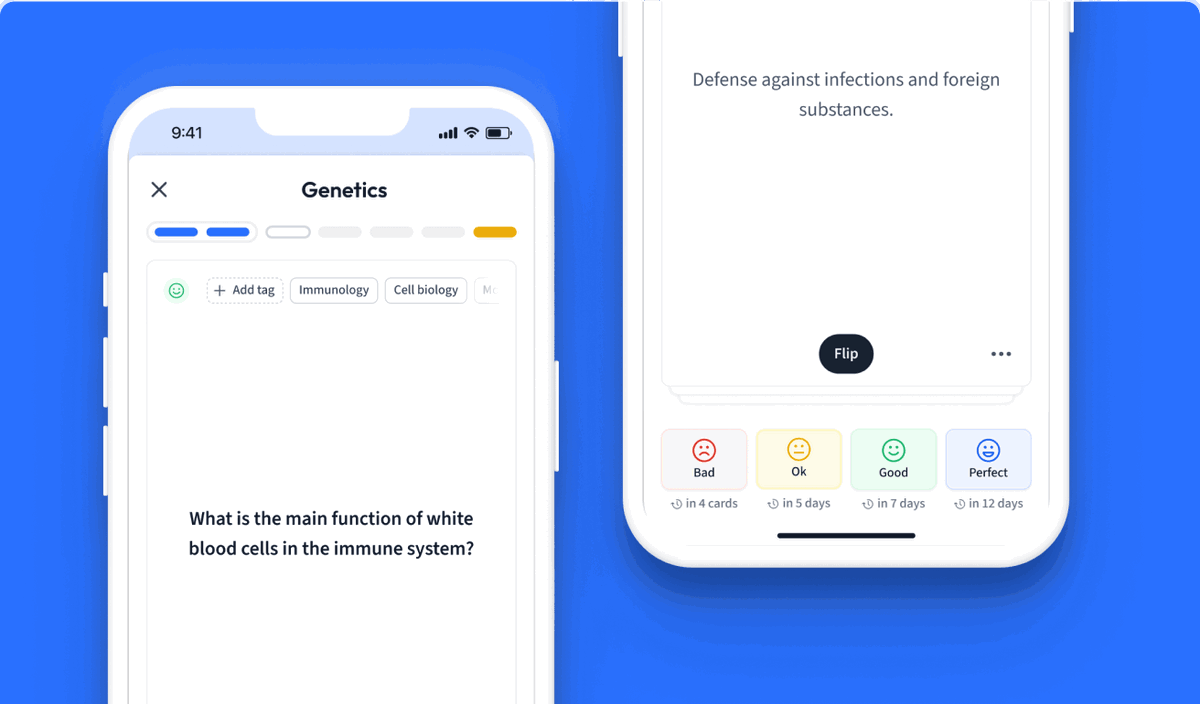
Learn with 12 tissue engineering flashcards in the free StudySmarter app
Already have an account? Log in
Frequently Asked Questions about tissue engineering
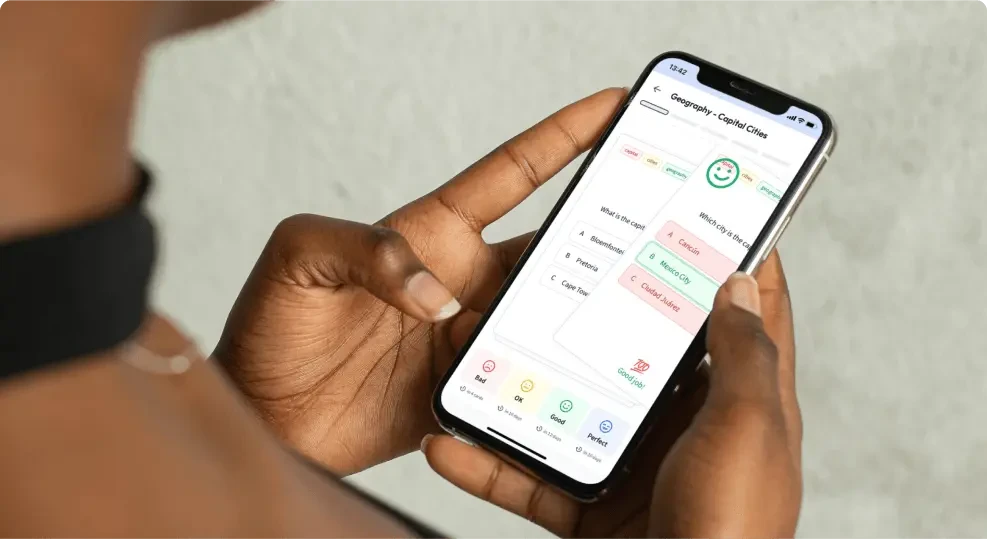
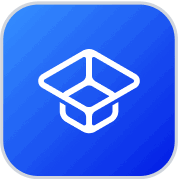
About StudySmarter
StudySmarter is a globally recognized educational technology company, offering a holistic learning platform designed for students of all ages and educational levels. Our platform provides learning support for a wide range of subjects, including STEM, Social Sciences, and Languages and also helps students to successfully master various tests and exams worldwide, such as GCSE, A Level, SAT, ACT, Abitur, and more. We offer an extensive library of learning materials, including interactive flashcards, comprehensive textbook solutions, and detailed explanations. The cutting-edge technology and tools we provide help students create their own learning materials. StudySmarter’s content is not only expert-verified but also regularly updated to ensure accuracy and relevance.
Learn more