Jump to a key chapter
Introduction to Microbial Genetics
Microbial genetics is a fascinating field that studies the organization and function of genetic material in microorganisms. It plays a crucial role in understanding more about the basic biological processes that drive life on Earth. As you explore this topic, you will gain insights into how these tiny organisms inherit and express characteristics, which is vital for advances in science and medicine.
What is Microbial Genetics?
Microbial genetics is a specialized branch of genetics focusing on microbes like bacteria, viruses, fungi, and protozoa. In this field, you learn about several complex processes, including DNA replication, transcription, and translation. These processes are crucial for understanding how microbes thrive, evolve, and interact with their environments.Microorganisms have a variety of genetic tools that allow them to adapt to various habitats and conditions. Some key genetic elements studied in microbes include:
- Plasmids: Small circular DNA molecules that exist independently of chromosomal DNA and can carry genes, including those for antibiotic resistance.
- Transposons: Segments of DNA that can move from one location to another within a genome, contributing to genetic diversity.
- Bacteriophages: Viruses that infect bacteria and can transfer genetic material between them.
Microbial Genetics: The study of genetic material organization, function, and behavior in microorganisms.
Importance of Microbial Genetics
Microbial genetics holds significant importance in various scientific fields and applications. Researchers utilize insights from microbial genetics to:
- Develop new antibiotics and combat infectious diseases by understanding how microbes develop resistance.
- Create innovative therapies and biotechnological tools, such as using plasmids for gene cloning and expression in green technologies.
- Advance knowledge in evolutionary biology by revealing microbe adaptation mechanisms to different environments.
- Control pathogenic microorganisms in agriculture and improve food safety and production methods.
Did you know that microbial genetics was pivotal in the discovery of the structure of DNA? In the early 20th century, studies on bacteriophages (viruses that infect bacteria) and bacterial transformation experiments provided key insights that led to the identification of DNA as the genetic material. These discoveries ultimately set the stage for the model of DNA as a double helix by Watson and Crick.
Microbial Genetics vs. Genomics
While both microbial genetics and genomics delve into the study of genes, the distinction between the two lies in their scope and focus.Microbial Genetics: This field primarily concentrates on the heredity and function of genes within individual microorganisms. You'll find it involves examining genetic variations, mutations, and the expressions related to structural, functional, and regulatory genes.Genomics: Genomics, on the other hand, takes a broader approach. It studies the entirety of an organism's genes – its genome. This involves sequencing, mapping, and analyzing genetic data to understand evolutionary relationships, genetic interplay, and comprehensive functions across species.Although closely related, these fields work together to provide an overall understanding of genetic blueprint influences on organism behavior and interactions.
Consider a practical application: When a new microbe that causes an infectious disease is discovered, researchers may first use microbial genetics to understand the specific genes related to pathogenicity. Subsequently, genomics might be used to sequence the entire genome, gaining insights into broader evolutionary aspects or potential gene targets for treatment.
Techniques in Microbial Genetics
Exploring techniques in microbial genetics is essential for unraveling the complexities of microbial life. These techniques serve as the backbone for current research and applications within the field.Through advancements in technology, a range of techniques has been developed to manipulate and study microbial genetic material, allowing researchers to understand microbial behaviors, functions, and interactions more comprehensively.
Common Techniques in Microbial Genetics
Understanding and manipulating genetic material in microorganisms require specialized techniques. Here are some widely used techniques in microbial genetics:
- Transformation: Introducing foreign DNA into a bacterial cell to study gene function or for genetic modification. This process can produce genetically altered microorganisms with desirable traits.
- Conjugation: A form of horizontal gene transfer where genetic material is exchanged between bacteria via direct contact, highlighting microbial interactions and evolution.
- Transduction: Utilizes bacteriophages to transfer DNA between bacteria, helping researchers study gene linkages and mapping.
- Polymerase Chain Reaction (PCR): Amplifies specific DNA sequences with high accuracy, facilitating deeper exploration of genetic sequences.
- Gene Knockout: This technique involves inactivating specific genes to study their functions and roles in microbial physiology.
For instance, scientists often use transformation to introduce genes coding for antibiotic resistance into bacteria as a way to study the mechanisms of resistance development. This insight aids in designing new antibiotics.
The method of conjugation was first discovered in the bacterium Escherichia coli by Joshua Lederberg and Edward Tatum in 1946. They revealed that bacteria could exchange genetic information, paving the way for understanding bacterial sexual reproduction and genetic mapping. This discovery earned Lederberg the Nobel Prize in 1958.
Tools and Technologies in Microbial Genetics
Innovation in tools and technologies has greatly expanded the capabilities of researchers in the field of microbial genetics. These advancements enable more precise manipulation and analysis of microbial DNA. Some key tools and technologies include:
- CRISPR-Cas9: A powerful gene-editing technology that allows researchers to accurately edit, delete, or insert specific genetic sequences within microbial genomes.
- Next-Generation Sequencing (NGS): Provides rapid sequencing of entire genomes, vastly improving the speed and detail at which microbial genetic information can be obtained.
- Bioinformatics: Utilizes computational tools to manage, analyze, and interpret vast amounts of genetic data, offering deeper insights into microbial genomics and evolution.
- Microarrays: Enables the analysis of gene expression patterns across thousands of genes simultaneously, facilitating broad studies on microbial responses and adaptation.
- DNA Synthesis and Cloning: Allows for the creation of synthetic DNA sequences, enabling the study of microbial gene functions and the development of engineered microbes for specific tasks.
CRISPR-Cas9 technology, originally discovered as an adaptive immune system in bacteria, has become a cornerstone for genetic research across all fields of biology, not just microbial genetics.
Applications of Microbial Genetics
Microbial genetics offers valuable insights and tools for various fields, leading to numerous real-world applications. Its versatility and adaptability make it a cornerstone in scientific, medical, and industrial advancements.
Real-World Applications of Microbial Genetics
Microbial genetics plays a crucial role in many real-world applications, offering solutions to problems across diverse sectors. Some notable applications include:
- Bioremediation: Utilizing genetically modified microorganisms to clean up pollutants and toxins from environments, microbial genetics enhances the efficiency of these processes by equipping microbes with genes that enable them to degrade various compounds.
- Food Production: Genetic techniques help in creating starter cultures for fermentation processes, improving both the quality and safety of food products such as yogurt, cheese, and sauerkraut.
- Environmental Monitoring: Microorganisms engineered to act as biosensors can detect and report on environmental changes, aiding in early warning systems for pollution and other ecological disturbances.
- Biofuel Production: Modified microbes can convert plant material into biofuels more efficiently, providing an alternative source of energy that is cleaner and more sustainable than fossil fuels.
An example of microbial genetics in action is the use of genetically modified Escherichia coli to produce insulin. By inserting the human insulin gene into the bacteria, they can efficiently produce insulin for therapeutic use, revolutionizing diabetes treatment.
The bacterium Geobacter has been genetically modified to improve its electricity-generating capabilities in microbial fuel cells, showcasing an innovative approach to sustainable energy solutions.
Impact on Medicine and Biotechnology
The impact of microbial genetics on medicine and biotechnology is profound, driving advancements that directly influence human health and technological innovation. Key impacts include:
- Drug Development: Understanding microbial genetics aids in the discovery and development of new antibiotics and antiviral agents by targeting microbial pathways and resistance mechanisms.
- Genetic Engineering: The manipulation of microbial genomes enables the production of recombinant proteins like insulin, growth hormones, and vaccines, enhancing therapeutic options.
- Disease Diagnosis: Genetic techniques help identify pathogens quickly and accurately, leading to faster diagnosis and treatment of infectious diseases.
- Gene Therapy Delivery: Engineered viruses, derived from microbial genetics studies, serve as vectors to deliver therapeutic genes for correcting genetic disorders.
In the field of synthetic biology, microbes are being engineered to perform complex tasks such as producing artificial meats or creating biosynthetic pathways for rare pharmaceuticals. This involves integrating multiple genes and pathways into microbial genomes, creating microorganisms capable of performing processes that naturally would not occur. This exemplifies the vast potential of microbial genetics to revolutionize industries and solve some of the world's most pressing problems.
Conjugation in Microbial Genetics
Conjugation is a fascinating process within microbial genetics that involves the transfer of genetic material between bacterial cells. This mode of genetic exchange allows bacteria to share beneficial traits, such as antibiotic resistance, across populations. Understanding conjugation is essential for studying microbial evolution and the spread of resistance.
Mechanisms of Conjugation in Microbial Genetics
The mechanism of conjugation is chiefly facilitated by direct contact between bacterial cells, usually mediated by a structure called the pilus. Here's how the process unfolds:
- A donor cell, containing the conjugative plasmid, forms a connection with a recipient cell via a pilus.
- The plasmid carries genetic information necessary for its transfer, including genes responsible for pilus formation and DNA mobilization.
- Once contact is established, the pilus retracts, bringing the cells close together.
- The donor cell replicates the plasmid DNA, transferring one copy to the recipient through the conjugation junction.
- As the DNA transfer occurs, the recipient cell synthesizes the complementary DNA strand, forming a complete plasmid.
- Eventually, the pilus disassembles, and the cells separate, each containing a copy of the plasmid.
Pilus: A hair-like appendage found on the surface of many bacteria, serving as a bridge for transferring plasmids during the conjugation process.
IMAGEImage URL: [Conjugation Process in Bacteria] (this is placeholder text for an image that you should envision) Description: Diagram showing pilus formation, plasmid transfer, and recipient cell conversion during bacterial conjugation.
In some bacteria, self-transmissible plasmids enable them to transfer not just plasmid DNA but sometimes fragments of chromosomal DNA to recipient cells. This process, known as Hfr conjugation (High frequency of recombination), can result in significant genetic exchanges and variations within bacterial populations. Hfr conjugation plays a crucial role in the genetic mapping of bacterial genomes, allowing researchers to determine gene locations and order on chromosomes.
Examples of Conjugation in Microbial Genetics
Conjugation in bacteria is a well-studied phenomenon, exemplified by various bacterial species and their interactions. An excellent example is the transfer of antibiotic resistance genes among pathogenic bacteria:In healthcare settings, the conjugation process can result in multidrug-resistant strains of bacteria by spreading resistance genes encoded on conjugative plasmids. For instance, the bacterium Escherichia coli can transfer plasmids carrying resistance genes to Salmonella through conjugation, posing challenges for disease treatment.The study of these mechanisms provides insights into bacterial resistance development, emphasizing the need for strategic approaches in antibiotic stewardship and infection control.
A noteworthy example includes the plasmid-mediated conjugation seen in Pseudomonas aeruginosa, where a plasmid carrying multidrug resistance genes is transferred to other Gram-negative bacteria in a hospital environment, underscoring the importance of effective sterilization protocols.
Preventing the spread of antibiotic resistance requires careful monitoring of plasmid transfer in microbial communities, highlighting conjugation as a key focus in microbial research and healthcare strategies.
microbial genetics - Key takeaways
- Microbial Genetics Definition: The study of genetic material organization, function, and behavior in microorganisms such as bacteria, viruses, fungi, and protozoa.
- Techniques in Microbial Genetics: Includes transformation, conjugation, transduction, PCR, gene knockout, and CRISPR-Cas9 for manipulating and studying microbial DNA.
- Applications of Microbial Genetics: Used in bioremediation, food production, environmental monitoring, biofuel production, and drug development.
- Microbial Genetics vs. Genomics: Genetics focuses on heredity and function of genes in microorganisms, while genomics studies the whole genome and its functions.
- Conjugation in Microbial Genetics: A method of horizontal gene transfer in bacteria involving direct contact and transfer of plasmids, contributing to genetic diversity and antibiotic resistance.
- Importance of Microbial Genetics: Crucial for developing new antibiotics, understanding microbial evolution, controlling pathogens, and advancing public health and biotechnology.
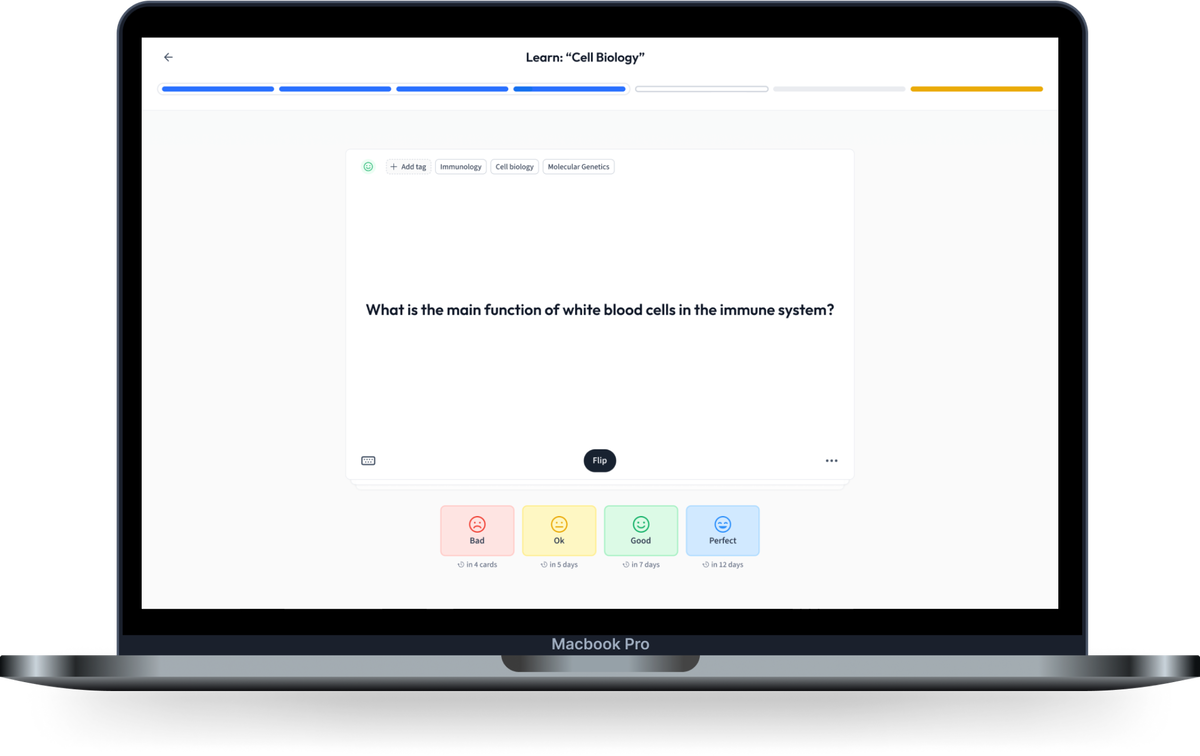
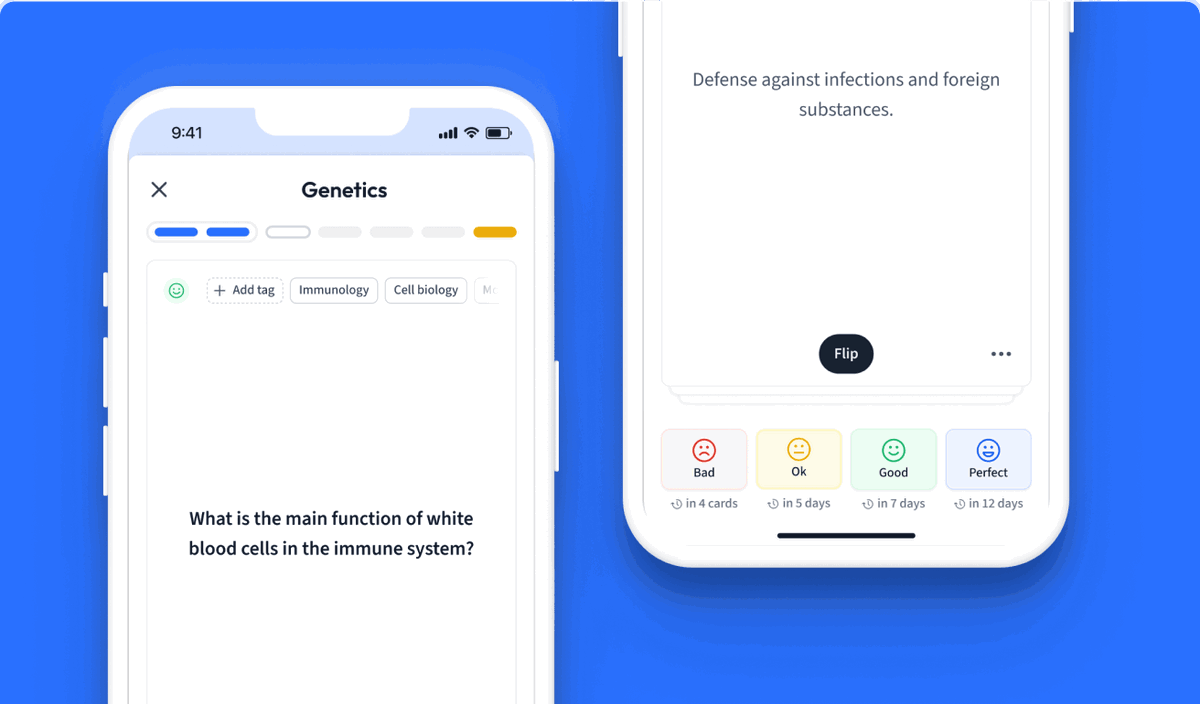
Learn with 12 microbial genetics flashcards in the free StudySmarter app
Already have an account? Log in
Frequently Asked Questions about microbial genetics
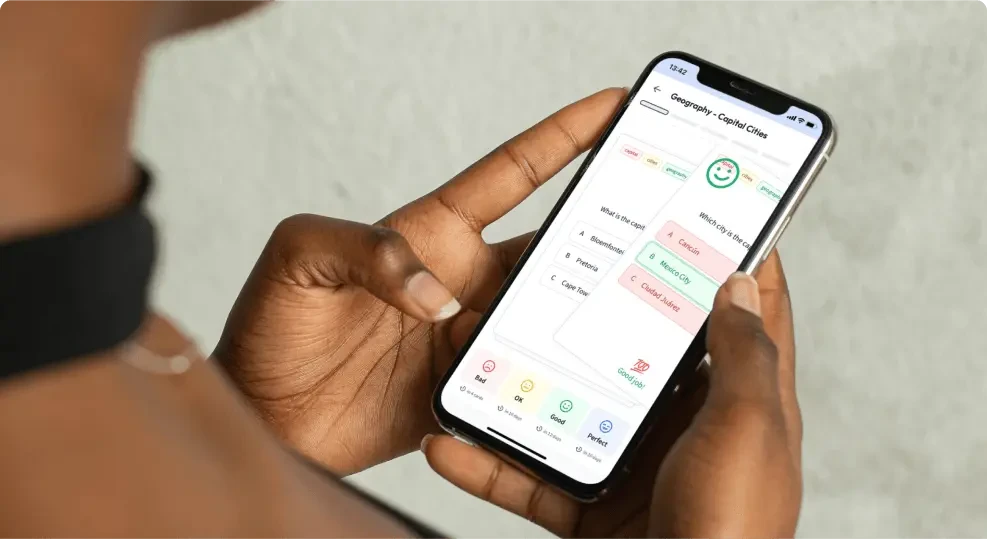
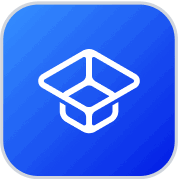
About StudySmarter
StudySmarter is a globally recognized educational technology company, offering a holistic learning platform designed for students of all ages and educational levels. Our platform provides learning support for a wide range of subjects, including STEM, Social Sciences, and Languages and also helps students to successfully master various tests and exams worldwide, such as GCSE, A Level, SAT, ACT, Abitur, and more. We offer an extensive library of learning materials, including interactive flashcards, comprehensive textbook solutions, and detailed explanations. The cutting-edge technology and tools we provide help students create their own learning materials. StudySmarter’s content is not only expert-verified but also regularly updated to ensure accuracy and relevance.
Learn more